-
PDF
- Split View
-
Views
-
Cite
Cite
Zheng-Bing Guan, Jun Yin, Kang Chen, Yan Shui, Yu-Jie Cai, Xiang-Ru Liao, The Hepatopancreas and Ovary are the Sites of Vitellogenin Synthesis in Female Red Swamp Crayfish (Procambarus Clarkii ()) (Decapoda: Astacoidea: Cambaridae), Journal of Crustacean Biology, Volume 36, Issue 5, 1 September 2016, Pages 637–641, https://doi.org/10.1163/1937240X-00002459
- Share Icon Share
Abstract
Vitellogenin (Vg) is the precursor of vitellin, the major yolk protein. The site of Vg synthesis in crustaceans remains controversial. This study investigated the synthesis site of Vg in female red swamp crayfish Procambarus clarkii (Girard, 1852). Results revealed that the mRNA of Vg was detected in the tissues of hepatopancreas of P. clarkii. The mRNA expression level of Vg increased in the previtellogenic stage and peaked in the vitellogenic stage in the hepatopancreas and ovary; the mRNA expression level of Vg in the hepatopancreas was approximately 20-25-fold of that of the ovary in the same ovarian stage. Eyestalk ablation could upregulate the mRNA expression level of Vg in both organs. Our RNAi experiment verified that the hepatopancreas and ovary are the Vg synthesis sites in female P. clarkii.
Introduction
Vitellogenin (Vg) is the precursor of vitellin (Vn) and is synthesized during the gonad maturation of female crustaceans. Gonad maturation in crustaceans is an energy-requiring process characterized by the rapid synthesis of Vn in the ovary during secondary vitellogenesis (Wilder et al., 2002; Tiu et al., 2009). Vn is the major yolk protein stored in oocytes, where this protein provides free amino acids, lipids, carbohydrates, carotenoids, and minerals to a growing and developing embryo and larva (Khalaila et al., 2004; Subramoniam, 2011). Vg is synthesized in the ovary and extra-ovarian tissues. During female maturation, extra-ovarian Vg is transported through the hemolymph to the ovary and sequestered into growing oocytes via receptor-mediated endocytosis. Vg undergoes proteolysis in oocytes to produce several subunits (Vns). The synthesis and accumulation of Vg are therefore crucial for oocyte and embryo development (Subramoniam, 2011).
The site of Vg synthesis in crustaceans remains controversial (Subramoniam, 2011; Girish et al., 2014). The sources of crustacean Vg have also been debated for many years. Some studies have revealed that Vg is synthesized mainly in the ovaries of species such as Penaeus semisulcatus de Haan, 1844 (Browdy et al., 1990) and Callinectes sapdiusRathbun, 1896 (Lee and Watson, 1995). Vg is synthesized exclusively in the hepatopancreas of other species such as Oziothelphusa senex (Fabricius, 1798) (Girish et al., 2014), Pandalus hypsinotusBrandt, 1851 (Tsutsui et al., 2004), Macrobrachium rosenbergii (De Man, 1879) (Yang et al., 2000), and Charybdis feriata (Linnaeus, 1758) (Mak et al., 2005). Several studies have also demonstrated that Vg is synthesized in both the hepatopancreas and ovary of species such as Macrobrachium nipponense (De Man, 1879) (Bai et al., 2015), Penaeus monodon (Fabricius, 1798) (Hiransuchalert et al., 2013), Scylla paramamosainEstampador, 1950 (Jia et al., 2013), Carcinus maenasLinnaeus, 1758 (Ding et al., 2010), Marsupenaeus japonicusSpence Bate, 1888 (Okumura et al., 2007), and Eriocheir sinensis H. Milne Edwards, 1853 (Li et al., 2006), but the contribution of the ovary is relatively less than that of the hepatopancreas.
The red swamp crayfish Procambarus clarkii (Girard, 1852) is a decapod crustacean of economic importance (Guan et al., 2014). The synthesis site of Vg in this species, however, has yet to be reported. In our study, an ORF fragment of the cDNA of P. clarkii Vg was isolated, and its tissue distribution analysis was subsequently undertaken through semi-quantitative RT-PCR. The expression profiles of the mRNA of Vg in the ovaries and hepatopancreas in different ovarian stages of intact and eyestalk-ablated crayfishes were subsequently examined through real-time quantitative PCR (real-time qPCR). Our results were validated through an RNA interference (RNAi) assay.
Materials and Methods
Experimental Animals, Eyestalk Ablation, and Tissue Collection
Domesticated female P. clarkii broodstock (length |$12.1-13.6\;{\rm{cm}}$| and weight 29.8-36.3 g) were collected from Jiangsu Baolong Breed Aquatics (Dafeng City, Jiangsu Province, P.R. China) in January 2015. The brooders were maintained in |$40\;{\rm{l}}$| aquaria containing filtered and aerated water at room temperature |$(25^\circ {\rm{C}})$|. The crayfish broodstock was fed with a commercially available diet twice daily. For tissue distribution analysis, various tissues of female broodstock were collected, immediately placed in liquid |${{\rm{N}}_2}$|, and stored at |$ - 80^\circ {\rm{C}}$| until use. The unilateral eyestalk was cut near the base by using a scalpel. The wounds were cauterized with a soldering iron before the animals were returned to the water. The ovarian developmental stages of P. clarkii were classified into early developmental, previtellogenetic, vitellogenetic, and mature ovaries according to the gonadosomatic index (GSI) (Sarojini et al., 1995; Guan et al., 2013).
Total RNA Isolation and First-Strand CDNA Synthesis
Total RNA was extracted from crayfish tissues by using TRIzol™ reagent (Invitrogen, Waltham, MA, USA). The extracted total RNA |$(10\;\mu {\rm{g}})$| was treated with DNase I (|$0.2\;{\rm{U}}/\mu {\rm{g}}\;{\rm{RNA}}$|; GE Healthcare, Chicago, IL, USA) at |$37^\circ {\rm{C}}$| for 30 min and subjected to phenol-chloroform extraction. DNase-I-treated total RNA |$(1.5\;\mu {\rm{g}})$| was reverse-transcribed by using reverse transcriptase XL (AMV; Takara, Dalian, P.R. China) in accordance with the manufacturer’s protocol.
Tissue Distribution Analysis
The mRNA expression of Vg in various tissues of female P. clarkii was examined through RT-PCR. The amplification reaction was conducted in a |$25\;\mu {\rm{l}}$| reaction mixture. A pair of primers (pcg1 and pcg2; Table 1) was used to amplify a |$915\;{\rm{bp}}$| fragment of the cDNA of Vg. The PCR conditions were as follows: 30 cycles |$(94^\circ {\rm{C}}/30\;{\rm{s}},\;57^\circ {\rm{C}}/30\;{\rm{s}},\;72^\circ {\rm{C}}/1\;{\rm{min}})$| and |$72^\circ {\rm{C}}$| for 8 min. The endogenously expressed mRNA of |$\beta $|-actin in crayfish (GenBank accession no. KR135165) was used as an internal control. A pair of primers (pcg5 and pcg6; Table 1) was used to amplify a |$344\;{\rm{bp}}$| fragment of the mRNA of |$\beta $|-actin in crayfish. The same schedule was used to amplify |$\beta $|-actin; however, 26 cycles instead of 30 cycles were set in this procedure. The assay was performed in accordance with previously described methods (Guan et al., 2007).
Primer sequences used in this study. The T7 promoter sequence is underlined.
Primer . | Direction . | Nucleotide sequence |$(5^\prime \to 3^\prime)$| . | Usage . |
---|---|---|---|
pcg1 | Forward | |${5^\prime }{\rm{-AATGTGCAAATTCCTTTACACACTCC-}}{3^\prime }$| | Vg RT-PCR |
pcg2 | Reverse | |${5^\prime }{\rm{-CTATTTACAGTAAGTAGGGAAGACCAG-}}{3^\prime }$| | Vg RT-PCR |
pcg5 | Forward | |${5^\prime }{\rm{-AGGACTCTTACGTGGGAGACGAG-}}{3^\prime }$| | |$\beta $|-actin RT-PCR |
pcg6 | Reverse | |${5^\prime }{\rm{-AGGAACAGTGTGTGACACGCCATC-}}{3^\prime }$| | |$\beta $|-actin RT-PCR |
pcg3 | Forward | |${5^\prime }{\rm{-TGTGGCGTGGAGGTACGAC-}}{3^\prime }$| | Vg real-time qPCR |
pcg4 | Reverse | |${5^\prime }{\rm{-GCTCACAACCATGGCGGTG-}}{3^\prime }$| | Vg real-time qPCR |
P3 | Forward | |${5^\prime }{\rm{-TGGTGCATGGCCGTTCTTA-}}{3^\prime }$| | 18S rRNA real-time qPCR |
P4 | Reverse | |${5^\prime }{\rm{-AATTGCTGGAGATCCGTCGAC-}}{3^\prime }$| | 18S rRNA real-time qPCR |
VR-1 | Forward | |${5^\prime }{\rm{-TAATACGACTCACTATAGGGAATGTGCAAATTCCTTTACAC-}}{3^\prime }$| | Vg dsRNA synthesis |
VR-2 | Reverse | |${5^\prime }{\rm{-TAATACGACTCACTATAGGGCAGTAAGTAGGGAAGACCAG-}}{3^\prime }$| | Vg dsRNA synthesis |
P7 | Forward | |${5^\prime }{\rm{-TAATACGACTCACTATAGGGCGACGTAAACGGCCACAAGT-}}{3^\prime }$| | GFP dsRNA synthesis |
P8 | Reverse | |${5^\prime }{\rm{-TAATACGACTCACTATAGGGCTTGTACAGCTCGTCCATGC-}}{3^\prime }$| | GFP dsRNA synthesis |
Primer . | Direction . | Nucleotide sequence |$(5^\prime \to 3^\prime)$| . | Usage . |
---|---|---|---|
pcg1 | Forward | |${5^\prime }{\rm{-AATGTGCAAATTCCTTTACACACTCC-}}{3^\prime }$| | Vg RT-PCR |
pcg2 | Reverse | |${5^\prime }{\rm{-CTATTTACAGTAAGTAGGGAAGACCAG-}}{3^\prime }$| | Vg RT-PCR |
pcg5 | Forward | |${5^\prime }{\rm{-AGGACTCTTACGTGGGAGACGAG-}}{3^\prime }$| | |$\beta $|-actin RT-PCR |
pcg6 | Reverse | |${5^\prime }{\rm{-AGGAACAGTGTGTGACACGCCATC-}}{3^\prime }$| | |$\beta $|-actin RT-PCR |
pcg3 | Forward | |${5^\prime }{\rm{-TGTGGCGTGGAGGTACGAC-}}{3^\prime }$| | Vg real-time qPCR |
pcg4 | Reverse | |${5^\prime }{\rm{-GCTCACAACCATGGCGGTG-}}{3^\prime }$| | Vg real-time qPCR |
P3 | Forward | |${5^\prime }{\rm{-TGGTGCATGGCCGTTCTTA-}}{3^\prime }$| | 18S rRNA real-time qPCR |
P4 | Reverse | |${5^\prime }{\rm{-AATTGCTGGAGATCCGTCGAC-}}{3^\prime }$| | 18S rRNA real-time qPCR |
VR-1 | Forward | |${5^\prime }{\rm{-TAATACGACTCACTATAGGGAATGTGCAAATTCCTTTACAC-}}{3^\prime }$| | Vg dsRNA synthesis |
VR-2 | Reverse | |${5^\prime }{\rm{-TAATACGACTCACTATAGGGCAGTAAGTAGGGAAGACCAG-}}{3^\prime }$| | Vg dsRNA synthesis |
P7 | Forward | |${5^\prime }{\rm{-TAATACGACTCACTATAGGGCGACGTAAACGGCCACAAGT-}}{3^\prime }$| | GFP dsRNA synthesis |
P8 | Reverse | |${5^\prime }{\rm{-TAATACGACTCACTATAGGGCTTGTACAGCTCGTCCATGC-}}{3^\prime }$| | GFP dsRNA synthesis |
Primer sequences used in this study. The T7 promoter sequence is underlined.
Primer . | Direction . | Nucleotide sequence |$(5^\prime \to 3^\prime)$| . | Usage . |
---|---|---|---|
pcg1 | Forward | |${5^\prime }{\rm{-AATGTGCAAATTCCTTTACACACTCC-}}{3^\prime }$| | Vg RT-PCR |
pcg2 | Reverse | |${5^\prime }{\rm{-CTATTTACAGTAAGTAGGGAAGACCAG-}}{3^\prime }$| | Vg RT-PCR |
pcg5 | Forward | |${5^\prime }{\rm{-AGGACTCTTACGTGGGAGACGAG-}}{3^\prime }$| | |$\beta $|-actin RT-PCR |
pcg6 | Reverse | |${5^\prime }{\rm{-AGGAACAGTGTGTGACACGCCATC-}}{3^\prime }$| | |$\beta $|-actin RT-PCR |
pcg3 | Forward | |${5^\prime }{\rm{-TGTGGCGTGGAGGTACGAC-}}{3^\prime }$| | Vg real-time qPCR |
pcg4 | Reverse | |${5^\prime }{\rm{-GCTCACAACCATGGCGGTG-}}{3^\prime }$| | Vg real-time qPCR |
P3 | Forward | |${5^\prime }{\rm{-TGGTGCATGGCCGTTCTTA-}}{3^\prime }$| | 18S rRNA real-time qPCR |
P4 | Reverse | |${5^\prime }{\rm{-AATTGCTGGAGATCCGTCGAC-}}{3^\prime }$| | 18S rRNA real-time qPCR |
VR-1 | Forward | |${5^\prime }{\rm{-TAATACGACTCACTATAGGGAATGTGCAAATTCCTTTACAC-}}{3^\prime }$| | Vg dsRNA synthesis |
VR-2 | Reverse | |${5^\prime }{\rm{-TAATACGACTCACTATAGGGCAGTAAGTAGGGAAGACCAG-}}{3^\prime }$| | Vg dsRNA synthesis |
P7 | Forward | |${5^\prime }{\rm{-TAATACGACTCACTATAGGGCGACGTAAACGGCCACAAGT-}}{3^\prime }$| | GFP dsRNA synthesis |
P8 | Reverse | |${5^\prime }{\rm{-TAATACGACTCACTATAGGGCTTGTACAGCTCGTCCATGC-}}{3^\prime }$| | GFP dsRNA synthesis |
Primer . | Direction . | Nucleotide sequence |$(5^\prime \to 3^\prime)$| . | Usage . |
---|---|---|---|
pcg1 | Forward | |${5^\prime }{\rm{-AATGTGCAAATTCCTTTACACACTCC-}}{3^\prime }$| | Vg RT-PCR |
pcg2 | Reverse | |${5^\prime }{\rm{-CTATTTACAGTAAGTAGGGAAGACCAG-}}{3^\prime }$| | Vg RT-PCR |
pcg5 | Forward | |${5^\prime }{\rm{-AGGACTCTTACGTGGGAGACGAG-}}{3^\prime }$| | |$\beta $|-actin RT-PCR |
pcg6 | Reverse | |${5^\prime }{\rm{-AGGAACAGTGTGTGACACGCCATC-}}{3^\prime }$| | |$\beta $|-actin RT-PCR |
pcg3 | Forward | |${5^\prime }{\rm{-TGTGGCGTGGAGGTACGAC-}}{3^\prime }$| | Vg real-time qPCR |
pcg4 | Reverse | |${5^\prime }{\rm{-GCTCACAACCATGGCGGTG-}}{3^\prime }$| | Vg real-time qPCR |
P3 | Forward | |${5^\prime }{\rm{-TGGTGCATGGCCGTTCTTA-}}{3^\prime }$| | 18S rRNA real-time qPCR |
P4 | Reverse | |${5^\prime }{\rm{-AATTGCTGGAGATCCGTCGAC-}}{3^\prime }$| | 18S rRNA real-time qPCR |
VR-1 | Forward | |${5^\prime }{\rm{-TAATACGACTCACTATAGGGAATGTGCAAATTCCTTTACAC-}}{3^\prime }$| | Vg dsRNA synthesis |
VR-2 | Reverse | |${5^\prime }{\rm{-TAATACGACTCACTATAGGGCAGTAAGTAGGGAAGACCAG-}}{3^\prime }$| | Vg dsRNA synthesis |
P7 | Forward | |${5^\prime }{\rm{-TAATACGACTCACTATAGGGCGACGTAAACGGCCACAAGT-}}{3^\prime }$| | GFP dsRNA synthesis |
P8 | Reverse | |${5^\prime }{\rm{-TAATACGACTCACTATAGGGCTTGTACAGCTCGTCCATGC-}}{3^\prime }$| | GFP dsRNA synthesis |
Real-Time QPCR Analysis of the MRNA Expression Level of Vg in the Hepatopancreas and Ovary of Intact and Eyestalk-Ablated Crayfishes
Real-time qPCR experiment was performed to detect Vg transcripts in different hepatopancreas and ovary samples from P. clarkii. The manipulation of eyestalk ablation in domesticated female P. clarkii broodstock induces ovarian maturation 15 days after unilateral eyestalk ablation occurs (Guan et al., 2013). In the eyestalk-ablated group, the brooder ovaries were collected before (day 0, |$n = 10$|) and after the unilateral eyestalk ablation on day 1 (previtellogenic), day 7 (vitellogenic), and day 15 (mature; |$n = 10$| for each time point) (Guan et al., 2013). A pair of Vg primers (pcg3 and pcg4; Table 1) was used to amplify a |$130\;{\rm{bp}}$| product, and 18S rRNA was used as an internal control to verify qPCR. Two primers, namely, P3 and P4 (Table 1), were used in the process. The assay was performed in accordance with previously described methods (Guan et al., 2013).
RNAi Knockdown of Vg Expression in Eyestalk-Ablated Crayfish
The PCR-generated partial DNA fragments of the cDNA of Vg in P. clarkii and the green fluorescent protein (GFP) gene served as a linearized DNA template for single-stranded (ss) RNA synthesis. The T7 promoter sequence was incorporated into the DNA templates by using appropriate primers (VR-1, VR-2, P7, and P8; Table 1) with a T7 promoter at their |${5^\prime }$|-ends. The T7 Ribo-MAX™ Express RNAi System (Promega, USA) that synthesized ssRNAs was used in accordance with the manufacturer’s instructions. The dsRNA |$(915\;{\rm{bp}})$| of Vg was generated in accordance with previously reported methods. The dsRNA |$(655\;{\rm{bp}})$| specific to GFP gene was generated by applying the same procedure and used as a control to exclude the non-specific dsRNA effect. The assay was performed in accordance with previous methods (Guan et al., 2013).
Results
MRNA Expression of Vg in Different Tissues of Female P. clarkii
According to an EST sequence (GenBank accession no. KR135171), an ORF fragment, including |$915\;{\rm{bp}}$|, of the cDNA of P. clarkii Vg was cloned from the total RNA of the hepatopancreas through RT-PCR. This fragment, encoding 304 amino acids (aa), is identical to that of the Vg of the following crustaceans at an amino acid level: Homarus americanus H. Milne Edwards, 1837,|$(61\% )$|, Cherax quadricarinatus (von Martens, 1868) |$(59\% )$|, Pandalopsis japonicaBalss, 1914,|$(50\% )$|, Litopenaeus vannamei (Boone, 1931) |$(48\% )$|, Fenneropenaeus merguiensis (De Man, 1888) |$(48\% )$|, and Penaeus semisulcatus|$(48\% )$|. The mRNA expression of Vg in different tissues was analyzed through semi-quantitative RT-PCR. The cDNA fragment of the expected size |$915\;{\rm{bp}}$| of Vg was detected in the hepatopancreas and ovary but was not found in eyestalks, brain, intestine, muscle, gills, and stomach (Fig. 1). The mRNA of |$\beta $|-actin was used as an internal control for each sample.
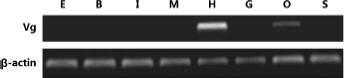
Tissue distribution analysis of the mRNA of Vg in female Procambarus clarkii broodstock through RT-PCR (30 cycles). E, eyestalk; B, brain; I, intestine; M, muscle; H, hepatopancreas; G, gills; O, ovary; S, stomach.
MRNA Expression Profiles of Vg in the Hepatopancreas and Ovary During the Ovarian Development of P. clarkii
Real-time qPCR was performed to determine the mRNA expression of Vg in naturally maturing and unilateral eyestalk-ablated crayfishes. In the ovary, the mRNA expression level of Vg increased in the previtellogenic stage, significantly increased in the vitellogenic stage |$(P \lt 0.05)$|, and clearly decreased in the mature stage of intact broodstock (Fig. 2A). In eyestalk-ablated broodstock, a low mRNA expression level of Vg was also observed in the ovaries in early developmental stages. Its expression was significantly increased in the previtellogenic stage and peaked in the vitellogenic stage |$(P \lt 0.05)$|. This expression in the mature ovaries was slightly decreased afterward (Fig. 2A).
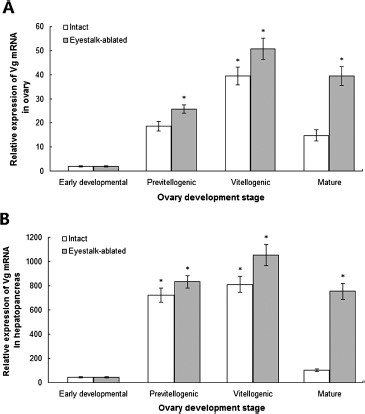
Real-time qPCR analysis of the mRNA expression level of Vg in the ovary (A) and hepatopancreas (B) in different ovarian development stages of intact and eyestalk-ablated female Procambarus clarkii broodstock. Data are represented as |${\rm{mean}} \pm {\rm{SD}}$||$(n = 10)$|. *Significant difference at |$P \lt 0.05$|.
In the hepatopancreas, the relative mRNA expression level of Vg was significantly increased in the previtellogenic stage, peaked in the vitellogenic stages |$(P \lt 0.05)$|, and remarkably decreased in the mature ovaries of intact broodstock (Fig. 2B). In the eyestalk-ablated broodstock, its expression was also significantly increased in the previtellogenic stage, peaked in the vitellogenic stage |$(P \lt 0.05)$|, and slightly decreased in the mature ovaries (Fig. 2B). The mRNA levels of Vg in the ovaries and hepatopancreas of eyestalk-ablated broodstock were greater than those of the tissues in the same ovarian stages in the intact broodstock. The relative mRNA expression levels of Vg in the hepatopancreas were approximately 20-fold to 25-fold greater than those in the tissues in previtellogenic, vitellogenic, and mature ovaries (Fig. 2).
Expression of Vg After Its RNAi Knockdown in Eyestalk-Ablated Crayfish
The mRNA expression patterns of Vg in the ovaries and hepatopancreas of crayfish were further confirmed via the RNAi experiment by using the eyestalk-ablated crayfish as our study model. After the unilateral eyestalk was removed, the experimental broodstock was injected immediately with the dsRNA |$(0.5\;\mu {\rm{g/g}})$| of Vg. The PBS injection and the GFP dsRNA injection were used as controls. The silencing effect percentage was calculated by excluding the relative expression of the Vg dsRNA-treated group from the PBS group through real-time qPCR analysis. The constant expression levels of the Vg transcripts in the two control groups were compared, and the results revealed a significant reduction of the Vg transcripts in the ovary in the knockdown group on days 1, 7, and 15 post-injection (Fig. 3A). The data demonstrated that injecting Vg dsRNA into the crayfish elicited a silencing effect (approx. |$50\% $|) in the ovary on day 1 post-injection. The maximum effect (approx. |$85\% $|) in the ovary was observed on day 15 post-injection. A stronger inhibitory effect on the mRNA expression of Vg emerged in the hepatopancreas (Fig. 3B). The results indicated that a specific Vg knockdown was achieved at a transcription level. The PBS and the GFP dsRNA did not show any detectable effect on the mRNA expression of Vg. The mRNA reduction induced by Vg dsRNA injection was therefore a gene-specific effect. The ovaries of the Vg-silenced crayfishes were kept in the previtellogenic stage on the basis of their color and GSI values.
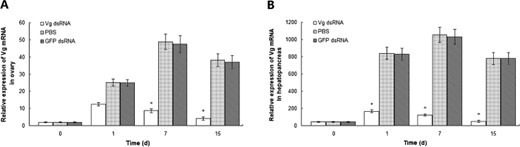
Real-time qPCR analysis of the RNAi effects of Vg on its mRNA expression in the ovary (A) and hepatopancreas (B) of eyestalk-ablated female Procambarus clarkii broodstock (from days 1 to 15). Data are represented as |${\rm{mean}} \pm {\rm{SD}}$||$(n = 10)$|. *Significant difference at |$P \lt 0.05$|.
Discussion
This study aimed to explore the sites of Vg synthesis in female P. clarkii. Vg is secreted by the liver in vertebrates, the fat body in insects, and the intestine in nematodes (Subramoniam, 2011; Girish et al., 2014). The major site of Vg production in crustaceans remains controversial nevertheless. In our study, the RT-PCR results indicated that the mRNA of Vg in female P. clarkii was highly expressed in the hepatopancreas, followed by the ovary, In contrast, the mRNA of Vg in female P. clarkii was not expressed in eyestalks, brain, intestine, muscle, gills, and stomach. This result is consistent with that in some crustacean species; thus, species specificity is possibly accounted for the multiple synthesis sites of Vg in crustaceans. This study suggested the potential coexistence of an extra-ovarian Vg synthesis pathway and an ovarian auto-synthesis pathway in P. clarkii.
Vg exhibits variable transcript levels in an oogenic cycle (Raviv et al., 2006; Phiriyangkul et al., 2007). In several crustaceans, the mRNA synthesis of Vg increases from the previtellogenic stage to the vitellogenic or the maturation stage of the ovary and then decreases after oviposition is completed (Okumura et al., 2007; Jia et al., 2013). We found that the mRNA expression level of Vg in female P. clarkii increased from the previtellogenic stage and peaked in the vitellogenic stage in the hepatopancreas and ovary. Moreover, the relative mRNA expression level of Vg was much higher (approximately 20-fold to 25-fold) in the hepatopancreas than in the ovary. On the basis of Vg tissue distribution and expression pattern during ovarian development, we concluded that the hepatopancreas and ovary are both sites of Vg synthesis in P. clarkii. The hepatopancreas is the main synthesis site.
Eyestalk ablation likely cuts off the source of GIH and results in rapid vitellogenesis in female decapod crustaceans (Subramoniam, 2011). Our research revealed that the mRNA of Vg was remarkably upregulated in the hepatopancreas and ovary one day after the unilateral eyestalk was removed. The mRNA level of Vg in the hepatopancreas and ovary was greater than that in the same ovarian stage in intact females. This difference suggested that eyestalk hormone regulates Vg transcription, and its target tissues for Vg inhibition are both the hepatopancreas and ovary. Although eyestalk ablation can cause a rapid increase in the mRNA of hepatopancreas and ovarian Vg during vitellogenesis and final maturation, low-quality spawns are observed in eyestalk-ablated decapod crustaceans such as P. monodon (Wongprasert et al., 2006; Hiransuchalert et al., 2013). Alternative measures should therefore be established to induce ovarian development and maturation in economically valuable crustaceans without the use of eyestalk ablation and thus obtain high-quality spawners.
The RNAi experiment revealed that the Vg RNAi treatment effectively inhibited the ovarian development of P. clarkii, and the mRNA expression level of Vg was significantly lower in the hepatopancreas and ovary tissues than in the control tissues. These observations suggested that the knockdown of Vg expression can hinder the ovarian development and maturation of P. clarkii. The RNAi data confirmed the Vg expression sites in the hepatopancreas and ovary of female P. clarkii and revealed the functional involvement of Vg during oogenesis in this economically valuable species.
Acknowledgements
This work was supported by the National Natural Science Foundation of China (31472003), a Project Funded by the Priority Academic Program Development of Jiangsu Higher Education Institutions, the 111 Project (No. 111-2-06), and the Jiangsu province “Collaborative Innovation Center for Advanced Industrial Fermentation” industry development program. Thanks to the anonymous reviewers for their valuable suggestions.