-
PDF
- Split View
-
Views
-
Cite
Cite
Ziteng Wang, Ching Yee Fong, Evelyn Mei Ling Goh, Hooi Yan Moy, Eric Chun Yong Chan, Transesterification of Indazole-3-carboxamide Synthetic Cannabinoids: Identification of Metabolite Biomarkers for Diagnosing Co-abuse of 5F-MDMB-PINACA and Alcohol, Journal of Analytical Toxicology, Volume 46, Issue 9, November 2022, Pages 1016–1024, https://doi.org/10.1093/jat/bkab121
- Share Icon Share
Abstract
Concurrent use of alcohol with synthetic cannabinoids (SCs) has been widely recorded among drug abusers. The susceptibilities of three indazole-3-carboxamide type SCs with methyl ester moiety, 5F-MDMB-PINACA, 5F-MMB-PINACA, and MMB-FUBINACA, to transesterification in the presence of ethanol warranted further investigation in view of probable augmented toxicity. In vitro metabolite identification experiments were first performed using human liver microsomes (HLMs) to characterize the novel metabolites of the three parent SCs in the presence of ethanol. Formation of transesterified metabolite, hydrolyzed metabolite, and several oxidative metabolites in HLM in the presence of alcohol was further determined for each parent SC and the respective ethyl ester analog, 5F-EDMB-PINACA, 5F-EMB-PINACA, and EMB-FUBINACA, to quantitatively elucidate transesterification and hydrolysis activities. Our results suggested that all three SCs undergo carboxylesterase-mediated transesterification to their respective ethyl ester analog in the presence of ethanol, which was incubation time- and ethanol concentration-dependent. Each ethyl ester metabolite was sequentially and readily metabolized to novel oxidative metabolites with the intact ethyl ester moiety and the same hydrolyzed metabolite as derived from its parent SC. A smaller extent of transesterification was non-enzymatically driven. Notably, we proposed 5F-EDMB-PINACA oxidative defluorination metabolite as the biomarker for diagnosing the potential co-abuse of 5F-MDMB-PINACA and alcohol. Due to the comparable pharmacological activities between each SC and its ethyl ester metabolite, augmented toxicity associated with co-abuse of SCs and alcohol is probable and deserves further investigation.
Introduction
Synthetic cannabinoids (SCs) are a class of novel psychoactive substances (NPSs) that demonstrate high affinities for the cannabinoid type 1 and 2 receptors (CB1 and CB2). The emergence of these designer drugs has led to significant public health challenges due to their elusive chemical identities, unpredictable toxicity, and increasing abuse potential. The illicit manufacturing and sale of SCs dated back to the early 2000s. A total of 190 substances has been notified by the EU Early Warning System from 2008 to 2018 (1) and about 280 have been reported worldwide to the United Nations Office on Drugs and Crime (UNODC) in 2020 (2).
5F-MDMB-PINACA (5F-ADB; Methyl 2-[1-(5-fluoropentyl)-1H-indazole-3-carboxamido]-3,3-dimethylbutanoate) is an indazole-3-carboxamide type SC. The first appearance of 5F-MDMB-PINACA in the European drug market was in September 2014 and it was the most seized SC in the USA in 2018 (3, 4). 5F-MMB-PINACA (5F-AMB; methyl 2-[1-(5-fluoropentyl)-1H-indazole-3-carboxamido]-3-methylbutanoate) is another indazole-based SC. 5F-MMB-PINACA has been implicated in multiple cases since its first identification in an illegal herbal product sold via the internet in Japan in 2014 (5). MMB-FUBINACA (AMB-FUBINACA; FUB-AMB; methyl 2-[1-(4-fluorobenzyl)-1H-indazole-3-carboxamido]-3-methylbutanoate) is structurally similar to 5F-MMB-PINACA with the replacement of 5-fluoropentyl to 4-fluorophenyl substituent at the 1-position. It was first detected in confiscated drugs in 2015 (6) and was well known for the “Zombie” outbreak in New York in 2016 (7). All the three indazole-3-carboxamide variants (Figure 1) have a methyl ester side chain and ester hydrolysis was reported to be their major metabolic pathway (3, 8, 9). Notably, the ethyl ester analogs of the three SCs are also available in the illicit drug market namely, 5F-EDMB-PINACA (ethyl 2-[1-(5-fluoropentyl)-1H-indazole-3-carboxamido]-3,3-dimethylbutanoate), 5F-EMB-PINACA (5F-AEB; ethyl 2-[1-(5-fluoropentyl)-1H-indazole-3-carboxamido]-3-methylbutanoate), and EMB-FUBINACA (AEB-FUBINACA; FUB-AEB; ethyl 2-[1-(4-fluorobenzyl)-1H-indazole-3-carboxamido]-3-methylbutanoate), respectively (Figure 1).
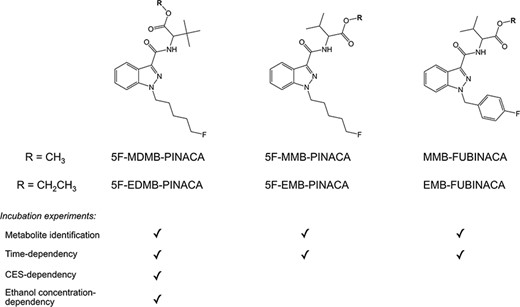
Chemical structures of 5F-MDMB-PINACA, 5F-MMB-PINACA, and MMB-FUBINACA and their corresponding ethyl ester analogs, 5F-EDMB-PINACA, 5F-EMB-PINACA, and EMB-FUBINACA. A tick (√) represents the type of incubation experiments performed on each compound namely metabolite identification, time-dependency, CES-dependency, or ethanol concentration-dependency experiment.
Transesterification, a process where an ester is transformed into another through interchange of the alkoxy moiety (Supplementary Figure S1), has important biological consequences. One widely reported example that has been studied in vitro and in vivo is the transesterification of cocaine (10, 11). In the presence of co-administered ethanol, around 34% of orally administrated cocaine could undergo transesterification with ethanol via carboxylesterases (CES) to form a new active ethyl ester metabolite, cocaethylene (12). Consequently, the socially plausible co-abuse of cocaine and ethanol has been reported to be associated with augmented morbidity and mortality (11).
The high prevalence of concurrent consumption of alcohol with SCs is well documented (13–16). An in vitro study discovered CES-catalyzed transesterification of four SCs with quinolinyl ester moiety, namely, PB-22, NPB-22, 5F-PB-22, and 5F-NPB-22, to the formation of ethyl ester metabolites, which activated CB1 and CB2 less potently compared to the parent compounds (17). The appearance of 5F-PB-22 ethyl ester was subsequently verified in a postmortem blood sample (16). In addition, the cytochrome P450 enzyme (P450)-derived hydroxylated (oxidative) metabolites were concluded to be downstream metabolites of the ethyl ester metabolites and further suggested as unique surrogate metabolites for identifying the combined use of SCs and alcohol. Nonetheless, the hydrolysis of the ethyl ester metabolites of these SCs by CES to hydrolyzed metabolites was not reported. Conversely, for cocaine and methylphenidate which possess the methyl ester moiety, their ethyl ester metabolites yielded comparable biological activities and could be further metabolized by CES to the same hydrolyzed metabolites as their respective parent drugs (18, 19). The question then arises, what are the susceptibilities of the three indazole-3-carboxamide type SCs to transesterification, and their ethyl ester metabolites to enzymatic hydrolysis by CES versus hydroxylation by P450 (Supplementary Figure S1)? Addressing this question is pertinent in understanding the enzyme-substrate specificity of the ethyl ester metabolites and identifying the relevant metabolite biomarkers for diagnosing the co-abuse of SCs and alcohol.
To address this question, we judiciously selected the three SCs with methyl ester moiety and comprehensively investigated their in vitro metabolism in the presence and absence of alcohol (Figure 1). Our findings shed insights on the potential pharmacological impact of alcohol on the three SCs from the perspectives of transesterification and the potential metabolite biomarkers for the forensic diagnosis of co-abuse of these SCs with alcohol.
Materials and Methods
Chemicals and reagents
5F-MDMB-PINACA, 5F-EDMB-PINACA, 5F-MDMB-PINACA oxidative defluorination metabolite (5F-ADB metabolite 2; methyl-2-[1-(5-hydroxypentyl)-1H-indazole-3-carboxamido]-3,3-dimethylbutanoate), 5F-MDMB-PINACA hydrolysis metabolite (5F-ADB metabolite 7; 2-[1-(5-fluoropentyl)-1H-indazole-3-carboxamido]-3,3-dimethylbutanoic acid), 5F-MMB-PINACA, 5F-EMB-PINACA, 5F-MMB-PINACA hydrolysis metabolite (5F-AMB metabolite 7; 2-[1-(5-Fluoropentyl)-1H-indazole-3-carboxamido]-3-methylbutanoic acid), MMB-FUBINACA, EMB-FUBINACA, MMB-FUBINACA hydrolysis metabolite (AB-FUBINACA metabolite 3; 2-[1-(4-fluorobenzyl)-1H-indazole-3-carboxamido]-3-methylbutanoic acid), and AM2201 N-(4-hydroxypentyl) metabolite-d5 (internal standard, IS) were kindly provided by the Health Sciences Authority, Singapore. UltraPool human liver microsomes (HLMs) of 150 donors and Gentest NADPH Regenerating System were purchased from Corning (Corning, NY). Bis(4-nitrophenyl)phosphate (BNPP) was purchased from Sigma-Aldrich (St. Louis, MO). High-performance chromatography-grade acetonitrile (ACN), methanol, ethanol, and formic acid were obtained from Merck (Kenilworth, NJ). All other commercially available chemicals were of analytical grade.
General in vitro metabolism incubation assay
The general in vitro metabolism incubation assay was carried out as previously described (20, 21). In brief, the pre-reaction mixture comprised potassium phosphate buffer (100 mM, pH 7.4), HLM (0.5 mg/mL), and NADPH solution A and B. After pre-warming at 37°C and mixing at 120 rpm for 5 min in a shaking incubator, the reaction was initiated by adding the substrate (final concentration 1 µM) and alcohol (ethanol or methanol) into the pre-reaction mixture to a final volume of 100 μL. At predefined time points, the reaction was terminated by transferring 80 μL of reaction mixture into an equal volume of ice-cold ACN containing 0.1 μM of IS. Quenched samples were centrifuged at 2775 g, 4°C for 30 min, and the supernatant was then subjected to liquid chromatography--mass spectrometry (LC--MS) analysis.
Metabolite identification in the presence of ethanol
Parent compound (5F-MDMB-PINACA, 5F-MMB-PINACA or MMB-FUBINACA) was incubated in the reaction mixture containing 12 µL/mL (v/v) ethanol for 15 min. Another experiment for their ethyl ester analogs (5F-EDMB-PINACA, 5F-EMB-PINACA or EMB-FUBINACA) was similarly performed without adding ethanol to confirm the formation of their oxidative metabolites. Additional CES inhibitor BNPP was added for 5F-EMB-PINACA and EMB-FUBINACA to enhance the oxidative biotransformation.
The supernatant was analyzed via liquid chromatography coupled to a Q-Exactive Hybrid Quadrupole-Orbitrap Mass Spectrometer (Thermo Scientific, Fremont, CA) (see Supplementary Methods). Data were acquired with the Full Scan/data-dependent MS2 (Full MS/dd-MS2) acquisition mode. Target metabolites of the three parent compounds were identified based on previous data (3, 8, 9, 22, 23). Potential oxidative metabolites of the ethyl ester analogs were then putatively identified by assuming comparable fragmentation patterns.
Determination of transesterification and hydrolysis activities
Time-dependent transesterification activities for the three parent compounds and their ethyl ester analogs as substrates were assessed with co-incubation of 6 µL/mL (v/v) ethanol or methanol, respectively. Samples were collected at 0, 5, 15, 30, and 60 min for 5F-MDMB-PINACA/5F-EDMB-PINACA and 0, 15, and 30 min for the other four compounds. Reaction mixture containing 5F-MDMB-PINACA with 6 µL/mL (v/v) ethanol, or 5F-EDMB-PINACA with 6 µL/mL (v/v) methanol, in the presence/absence of 100 µM BNPP, was utilized to explore the involvement of CES in transesterification. Samples were quenched at 15 min. 5F-MDMB-PINACA was also incubated for 15 min with ethanol concentrations of 0, 3, 6, and 12 µL/mL (v/v) to determine alcohol concentration-dependent effect. The incubation experiments were performed in triplicates. Another experiment replacing HLM with potassium phosphate buffer was conducted in parallel to explore non-enzyme mediated transesterification.
Samples were injected into an ultra-high performance liquid chromatography-Xevo TQD tandem mass spectrometry instrument (Waters, Milford, MA) (see Supplementary Methods). Product ion information obtained from metabolite identification experiments was utilized for multiple reaction monitoring to quantify compounds. Oxidative metabolites of 5F-MMB-PINACA and MMB-FUBINACA were not included for analysis due to the low abundance observed in metabolite identification experiments. The peak area ratio of each compound to the IS was calculated for further analysis and comparison.
Results
Metabolite identification for parent compounds and their ethyl ester analogs
In all incubation samples, each parent compound, its ethyl ester analog and the ester hydrolysis metabolite were identified according to the similar product ion spectra, except for a mass shift of 14 Da for precursor ion derived from methyl moiety versus ethyl moiety (+14 Da) or carboxylic acid moiety (−14 Da). This mass shift feature was also applicable to the respective oxidative metabolite of each parent compound and its ethyl ester analog, because of the structural similarity.
When the ethyl ester analog was used as the substrate, the ester hydrolysis metabolite, which is identical to that of the parent compound, could be identified as expected. Several unique oxidative metabolites were detected as well. When the parent compound was incubated in the presence of ethanol, the ester hydrolysis metabolite and several oxidative metabolites were identified as previously reported (3, 8, 9, 22, 23). In addition, ethyl ester analog appeared as the transesterified metabolite, together with its oxidative metabolites as described above, suggesting sequential metabolism. Details of metabolite identification for each compound are summarized in Supplementary Results. The appearances, formulae, precursor masses, diagnostic product ions, mass errors, and retention times of metabolites of the three parent SCs formed within two incubation systems are summarized in Table I. Supplementary Figures S2–S4 illustrate the characteristic product ions and fragmentation pathways of all detected SCs and metabolites.
Metabolite Identification for Parent SCs, Namely, 5F-MDMB-PINACA, 5F-MMB-PINACA, and MMB-FUBINACA and Their Respective Ethyl Ester Analogs
. | . | . | . | Chromatography and mass spectrometry data . | ||||
---|---|---|---|---|---|---|---|---|
. | SCs and metabolites . | Parent SC as a substrate in the presence of ethanol . | Ethyl ester analog as a substrate in the absence of ethanol . | Retention time (min) . | Formula . | Precursor ion (m/z) . | Mass error (ppm) . | Major product ions (m/z) . |
1 | 5F-MDMB-PINACA | Substrate | 8.29 | C20H28FN3O3 | 378.2195 | 1.99 | 213, 233, 251, 318 | |
2 | 5F-EDMB-PINACA | √ | Substrate | 8.73 | C21H30FN3O3 | 392.2335 | −2.28 | 213, 233, 251, 318 |
3 | 5F-MDMB-PINACA ester hydrolysis metabolite | √ | √ | 7.19 | C19H26FN3O3 | 364.2000 | −0.85 | 213, 233, 251, 318 |
4 | 5F-MDMB-PINACA oxidative defluorination metabolite | √ | × | 6.83 | C20H29N3O4 | 376.2231 | 0.05 | 213, 231, 316 |
5 | 5F-EDMB-PINACA oxidative defluorination metabolite | √ | √ | 7.27 | C21H31N3O4 | 390.2396 | 2.22 | 213, 231, 316 |
1 | 5F-MMB-PINACA | Substrate | 7.85 | C19H26FN3O3 | 364.2030 | −0.26 | 213, 251, 304 | |
2 | 5F-EMB-PINACA | √a | Substrate | 8.26 | C20H28FN3O3 | 378.2192 | 1.20 | 213, 251, 304 |
3 | 5F-MMB-PINACA ester hydrolysis metabolite | √ | √ | 6.80 | C18H24FN3O3 | 350.1876 | 0.44 | 213, 233, 251, 304 |
4 | 5F-MMB-PINACA pentyl chain hydroxylation metabolite | √ | × | 6.55 | C19H26FN3O4 | 380.1985 | 1.29 | 249, 267, 320 |
5 | 5F-MMB-PINACA oxidative defluorination metabolite | √ | × | 6.34 | C19H27N3O4 | 362.2077 | 0.74 | 213, 231, 302 |
6 | 5F-EMB-PINACA pentyl chain hydroxylation metabolite | × | √ | 7.06 | C20H28FN3O4 | 394.2156 | 4.92 | 267, 320 |
7 | 5F-EMB-PINACA oxidative defluorination metabolite | × | √ | 6.83 | C20H29N3O4 | 376.2202 | −0.77 | 213, 231, 302 |
8 | 5F-EMB-PINACA indazole hydroxylation metabolite | × | √ | 7.64 | C20H28FN3O4 | 394.2110 | −0.68 | 229, 267, 320 |
1 | MMB-FUBINACA | Substrate | 8.01 | C21H22FN3O3 | 384.1707 | −2.85 | 109. 253, 271, 324 | |
2 | EMB-FUBINACA | √a | Substrate | 8.42 | C22H24FN3O3 | 398.1877 | 0.64 | 109, 253, 271, 324 |
3 | MMB-FUBINACA ester hydrolysis metabolite | √ | √ | 7.00 | C20H20FN3O3 | 370.1559 | −0.66 | 109. 253, 271, 324 |
4 | MMB-FUBINACA defluorobenzyl metabolite | √ | × | 5.85 | C14H17N3O3 | 276.1344 | 0.48 | 145, 163, 216 |
5 | MMB-FUBINACA butane moiety hydroxylation metabolite | √ | × | 6.87 | C21H22FN3O4 | 400.1672 | 1.22 | 109, 271 |
6 | EMB-FUBINACA defluorobenzyl metabolite | √b | √ | 6.38 | C15H19N3O3 | 290.1497 | −0.75 | 145, 163, 216 |
7 | EMB-FUBINACA butane moiety hydroxylation metabolite | × | √ | 7.05 | C22H24FN3O4 | 414.1822 | −0.39 | 109, 253, 271 |
8 | EMB-FUBINACA indazole hydroxylation metabolite | × | √ | 7.78 | C22H24FN3O4 | 414.1822 | −0.39 | 109, 269, 287, 340 |
9 | EMB-FUBINACA 4-fluoro-methylbenzene hydroxylation metabolite | × | √ | 7.71 | C22H24FN3O4 | 414.1822 | −0.39 | 163, 287, 340 |
. | . | . | . | Chromatography and mass spectrometry data . | ||||
---|---|---|---|---|---|---|---|---|
. | SCs and metabolites . | Parent SC as a substrate in the presence of ethanol . | Ethyl ester analog as a substrate in the absence of ethanol . | Retention time (min) . | Formula . | Precursor ion (m/z) . | Mass error (ppm) . | Major product ions (m/z) . |
1 | 5F-MDMB-PINACA | Substrate | 8.29 | C20H28FN3O3 | 378.2195 | 1.99 | 213, 233, 251, 318 | |
2 | 5F-EDMB-PINACA | √ | Substrate | 8.73 | C21H30FN3O3 | 392.2335 | −2.28 | 213, 233, 251, 318 |
3 | 5F-MDMB-PINACA ester hydrolysis metabolite | √ | √ | 7.19 | C19H26FN3O3 | 364.2000 | −0.85 | 213, 233, 251, 318 |
4 | 5F-MDMB-PINACA oxidative defluorination metabolite | √ | × | 6.83 | C20H29N3O4 | 376.2231 | 0.05 | 213, 231, 316 |
5 | 5F-EDMB-PINACA oxidative defluorination metabolite | √ | √ | 7.27 | C21H31N3O4 | 390.2396 | 2.22 | 213, 231, 316 |
1 | 5F-MMB-PINACA | Substrate | 7.85 | C19H26FN3O3 | 364.2030 | −0.26 | 213, 251, 304 | |
2 | 5F-EMB-PINACA | √a | Substrate | 8.26 | C20H28FN3O3 | 378.2192 | 1.20 | 213, 251, 304 |
3 | 5F-MMB-PINACA ester hydrolysis metabolite | √ | √ | 6.80 | C18H24FN3O3 | 350.1876 | 0.44 | 213, 233, 251, 304 |
4 | 5F-MMB-PINACA pentyl chain hydroxylation metabolite | √ | × | 6.55 | C19H26FN3O4 | 380.1985 | 1.29 | 249, 267, 320 |
5 | 5F-MMB-PINACA oxidative defluorination metabolite | √ | × | 6.34 | C19H27N3O4 | 362.2077 | 0.74 | 213, 231, 302 |
6 | 5F-EMB-PINACA pentyl chain hydroxylation metabolite | × | √ | 7.06 | C20H28FN3O4 | 394.2156 | 4.92 | 267, 320 |
7 | 5F-EMB-PINACA oxidative defluorination metabolite | × | √ | 6.83 | C20H29N3O4 | 376.2202 | −0.77 | 213, 231, 302 |
8 | 5F-EMB-PINACA indazole hydroxylation metabolite | × | √ | 7.64 | C20H28FN3O4 | 394.2110 | −0.68 | 229, 267, 320 |
1 | MMB-FUBINACA | Substrate | 8.01 | C21H22FN3O3 | 384.1707 | −2.85 | 109. 253, 271, 324 | |
2 | EMB-FUBINACA | √a | Substrate | 8.42 | C22H24FN3O3 | 398.1877 | 0.64 | 109, 253, 271, 324 |
3 | MMB-FUBINACA ester hydrolysis metabolite | √ | √ | 7.00 | C20H20FN3O3 | 370.1559 | −0.66 | 109. 253, 271, 324 |
4 | MMB-FUBINACA defluorobenzyl metabolite | √ | × | 5.85 | C14H17N3O3 | 276.1344 | 0.48 | 145, 163, 216 |
5 | MMB-FUBINACA butane moiety hydroxylation metabolite | √ | × | 6.87 | C21H22FN3O4 | 400.1672 | 1.22 | 109, 271 |
6 | EMB-FUBINACA defluorobenzyl metabolite | √b | √ | 6.38 | C15H19N3O3 | 290.1497 | −0.75 | 145, 163, 216 |
7 | EMB-FUBINACA butane moiety hydroxylation metabolite | × | √ | 7.05 | C22H24FN3O4 | 414.1822 | −0.39 | 109, 253, 271 |
8 | EMB-FUBINACA indazole hydroxylation metabolite | × | √ | 7.78 | C22H24FN3O4 | 414.1822 | −0.39 | 109, 269, 287, 340 |
9 | EMB-FUBINACA 4-fluoro-methylbenzene hydroxylation metabolite | × | √ | 7.71 | C22H24FN3O4 | 414.1822 | −0.39 | 163, 287, 340 |
Tick (√) and Cross (×) Represent the Absence and Presence of Metabolites Detected Based on Selective Chromatography and Mass Spectrometry Data. All substrates were detected.
Intensity was too low to trigger MS2 scan. Signal was confirmed by precursor ion and retention time.
Intensity was too low to trigger MS2 scan. Signal was putatively confirmed by that in EMB-FUBINACA sample.
Metabolite Identification for Parent SCs, Namely, 5F-MDMB-PINACA, 5F-MMB-PINACA, and MMB-FUBINACA and Their Respective Ethyl Ester Analogs
. | . | . | . | Chromatography and mass spectrometry data . | ||||
---|---|---|---|---|---|---|---|---|
. | SCs and metabolites . | Parent SC as a substrate in the presence of ethanol . | Ethyl ester analog as a substrate in the absence of ethanol . | Retention time (min) . | Formula . | Precursor ion (m/z) . | Mass error (ppm) . | Major product ions (m/z) . |
1 | 5F-MDMB-PINACA | Substrate | 8.29 | C20H28FN3O3 | 378.2195 | 1.99 | 213, 233, 251, 318 | |
2 | 5F-EDMB-PINACA | √ | Substrate | 8.73 | C21H30FN3O3 | 392.2335 | −2.28 | 213, 233, 251, 318 |
3 | 5F-MDMB-PINACA ester hydrolysis metabolite | √ | √ | 7.19 | C19H26FN3O3 | 364.2000 | −0.85 | 213, 233, 251, 318 |
4 | 5F-MDMB-PINACA oxidative defluorination metabolite | √ | × | 6.83 | C20H29N3O4 | 376.2231 | 0.05 | 213, 231, 316 |
5 | 5F-EDMB-PINACA oxidative defluorination metabolite | √ | √ | 7.27 | C21H31N3O4 | 390.2396 | 2.22 | 213, 231, 316 |
1 | 5F-MMB-PINACA | Substrate | 7.85 | C19H26FN3O3 | 364.2030 | −0.26 | 213, 251, 304 | |
2 | 5F-EMB-PINACA | √a | Substrate | 8.26 | C20H28FN3O3 | 378.2192 | 1.20 | 213, 251, 304 |
3 | 5F-MMB-PINACA ester hydrolysis metabolite | √ | √ | 6.80 | C18H24FN3O3 | 350.1876 | 0.44 | 213, 233, 251, 304 |
4 | 5F-MMB-PINACA pentyl chain hydroxylation metabolite | √ | × | 6.55 | C19H26FN3O4 | 380.1985 | 1.29 | 249, 267, 320 |
5 | 5F-MMB-PINACA oxidative defluorination metabolite | √ | × | 6.34 | C19H27N3O4 | 362.2077 | 0.74 | 213, 231, 302 |
6 | 5F-EMB-PINACA pentyl chain hydroxylation metabolite | × | √ | 7.06 | C20H28FN3O4 | 394.2156 | 4.92 | 267, 320 |
7 | 5F-EMB-PINACA oxidative defluorination metabolite | × | √ | 6.83 | C20H29N3O4 | 376.2202 | −0.77 | 213, 231, 302 |
8 | 5F-EMB-PINACA indazole hydroxylation metabolite | × | √ | 7.64 | C20H28FN3O4 | 394.2110 | −0.68 | 229, 267, 320 |
1 | MMB-FUBINACA | Substrate | 8.01 | C21H22FN3O3 | 384.1707 | −2.85 | 109. 253, 271, 324 | |
2 | EMB-FUBINACA | √a | Substrate | 8.42 | C22H24FN3O3 | 398.1877 | 0.64 | 109, 253, 271, 324 |
3 | MMB-FUBINACA ester hydrolysis metabolite | √ | √ | 7.00 | C20H20FN3O3 | 370.1559 | −0.66 | 109. 253, 271, 324 |
4 | MMB-FUBINACA defluorobenzyl metabolite | √ | × | 5.85 | C14H17N3O3 | 276.1344 | 0.48 | 145, 163, 216 |
5 | MMB-FUBINACA butane moiety hydroxylation metabolite | √ | × | 6.87 | C21H22FN3O4 | 400.1672 | 1.22 | 109, 271 |
6 | EMB-FUBINACA defluorobenzyl metabolite | √b | √ | 6.38 | C15H19N3O3 | 290.1497 | −0.75 | 145, 163, 216 |
7 | EMB-FUBINACA butane moiety hydroxylation metabolite | × | √ | 7.05 | C22H24FN3O4 | 414.1822 | −0.39 | 109, 253, 271 |
8 | EMB-FUBINACA indazole hydroxylation metabolite | × | √ | 7.78 | C22H24FN3O4 | 414.1822 | −0.39 | 109, 269, 287, 340 |
9 | EMB-FUBINACA 4-fluoro-methylbenzene hydroxylation metabolite | × | √ | 7.71 | C22H24FN3O4 | 414.1822 | −0.39 | 163, 287, 340 |
. | . | . | . | Chromatography and mass spectrometry data . | ||||
---|---|---|---|---|---|---|---|---|
. | SCs and metabolites . | Parent SC as a substrate in the presence of ethanol . | Ethyl ester analog as a substrate in the absence of ethanol . | Retention time (min) . | Formula . | Precursor ion (m/z) . | Mass error (ppm) . | Major product ions (m/z) . |
1 | 5F-MDMB-PINACA | Substrate | 8.29 | C20H28FN3O3 | 378.2195 | 1.99 | 213, 233, 251, 318 | |
2 | 5F-EDMB-PINACA | √ | Substrate | 8.73 | C21H30FN3O3 | 392.2335 | −2.28 | 213, 233, 251, 318 |
3 | 5F-MDMB-PINACA ester hydrolysis metabolite | √ | √ | 7.19 | C19H26FN3O3 | 364.2000 | −0.85 | 213, 233, 251, 318 |
4 | 5F-MDMB-PINACA oxidative defluorination metabolite | √ | × | 6.83 | C20H29N3O4 | 376.2231 | 0.05 | 213, 231, 316 |
5 | 5F-EDMB-PINACA oxidative defluorination metabolite | √ | √ | 7.27 | C21H31N3O4 | 390.2396 | 2.22 | 213, 231, 316 |
1 | 5F-MMB-PINACA | Substrate | 7.85 | C19H26FN3O3 | 364.2030 | −0.26 | 213, 251, 304 | |
2 | 5F-EMB-PINACA | √a | Substrate | 8.26 | C20H28FN3O3 | 378.2192 | 1.20 | 213, 251, 304 |
3 | 5F-MMB-PINACA ester hydrolysis metabolite | √ | √ | 6.80 | C18H24FN3O3 | 350.1876 | 0.44 | 213, 233, 251, 304 |
4 | 5F-MMB-PINACA pentyl chain hydroxylation metabolite | √ | × | 6.55 | C19H26FN3O4 | 380.1985 | 1.29 | 249, 267, 320 |
5 | 5F-MMB-PINACA oxidative defluorination metabolite | √ | × | 6.34 | C19H27N3O4 | 362.2077 | 0.74 | 213, 231, 302 |
6 | 5F-EMB-PINACA pentyl chain hydroxylation metabolite | × | √ | 7.06 | C20H28FN3O4 | 394.2156 | 4.92 | 267, 320 |
7 | 5F-EMB-PINACA oxidative defluorination metabolite | × | √ | 6.83 | C20H29N3O4 | 376.2202 | −0.77 | 213, 231, 302 |
8 | 5F-EMB-PINACA indazole hydroxylation metabolite | × | √ | 7.64 | C20H28FN3O4 | 394.2110 | −0.68 | 229, 267, 320 |
1 | MMB-FUBINACA | Substrate | 8.01 | C21H22FN3O3 | 384.1707 | −2.85 | 109. 253, 271, 324 | |
2 | EMB-FUBINACA | √a | Substrate | 8.42 | C22H24FN3O3 | 398.1877 | 0.64 | 109, 253, 271, 324 |
3 | MMB-FUBINACA ester hydrolysis metabolite | √ | √ | 7.00 | C20H20FN3O3 | 370.1559 | −0.66 | 109. 253, 271, 324 |
4 | MMB-FUBINACA defluorobenzyl metabolite | √ | × | 5.85 | C14H17N3O3 | 276.1344 | 0.48 | 145, 163, 216 |
5 | MMB-FUBINACA butane moiety hydroxylation metabolite | √ | × | 6.87 | C21H22FN3O4 | 400.1672 | 1.22 | 109, 271 |
6 | EMB-FUBINACA defluorobenzyl metabolite | √b | √ | 6.38 | C15H19N3O3 | 290.1497 | −0.75 | 145, 163, 216 |
7 | EMB-FUBINACA butane moiety hydroxylation metabolite | × | √ | 7.05 | C22H24FN3O4 | 414.1822 | −0.39 | 109, 253, 271 |
8 | EMB-FUBINACA indazole hydroxylation metabolite | × | √ | 7.78 | C22H24FN3O4 | 414.1822 | −0.39 | 109, 269, 287, 340 |
9 | EMB-FUBINACA 4-fluoro-methylbenzene hydroxylation metabolite | × | √ | 7.71 | C22H24FN3O4 | 414.1822 | −0.39 | 163, 287, 340 |
Tick (√) and Cross (×) Represent the Absence and Presence of Metabolites Detected Based on Selective Chromatography and Mass Spectrometry Data. All substrates were detected.
Intensity was too low to trigger MS2 scan. Signal was confirmed by precursor ion and retention time.
Intensity was too low to trigger MS2 scan. Signal was putatively confirmed by that in EMB-FUBINACA sample.
Time-dependent transesterification activity of 5F-MDMB-PINACA
The time course of formation of the metabolites from 5F-MDMB-PINACA in the presence of ethanol is shown in Figure 2A. Consistent with previous findings (20), 5F-MDMB-PINACA was eliminated in the incubation system rapidly, with hydrolysis metabolite being the most abundant metabolite. The amount of 5F-EDMB-PINACA formed was consistently lower than that of hydrolysis metabolite and decreased over time to a similar level of that observed in buffer sample, of which the abundance remained relatively stable. Moreover, oxidative defluorination metabolites of both 5F-MDMB-PINACA and 5F-EDMB-PINACA were persistently observed.

Transesterification and hydrolysis activities of 5F-MDMB-PINACA under different incubation conditions. Data are shown as mean ± SD. (A) Different incubation duration with ethanol concentration at 6 µL/mL (v/v). (B) In the absence/presence of CES inhibitor BNPP for 15 min incubation with ethanol concentration at 6 µL/mL (v/v). (C) Different ethanol concentration (v/v) in incubation system for 15 min incubation.
Involvement of CES in the transesterification of 5F-MDMB-PINACA
As shown in Figure 2B, BNPP significantly decreased the formation of 5F-EDMB-PINACA to a similar level of that observed in buffer sample. BNPP also resulted in reduction of hydrolysis metabolite and oxidative defluorination metabolite of 5F-EDMB-PINACA, while elevated abundances of parent SC and its oxidative defluorination metabolite were observed.
Ethanol concentration-dependent transesterification of 5F-MDMB-PINACA
Dependency of transesterification on the concentration (v/v) of enriched ethanol is illustrated in Figure 2C. 5F-EDMB-PINACA and its subsequent oxidative defluorination metabolite were not detected in the absence of ethanol and their abundance elevated with increasing concentration of ethanol up to 12 µL/mL (v/v). The abundance of 5F-EDMB-PINACA in buffer sample without enzyme was affected by the concentration (v/v) of ethanol as well.
Comparable transesterification of 5F-EDMB-PINACA in the presence of methanol
5F-EDMB-PINACA displayed similar transesterification reaction in the presence of methanol to generate 5F-MDMB-PINACA and its subsequent oxidative defluorination metabolite. The reaction was demonstrated to be time dependent and CES mediated (Supplementary Figure S5). Small amount of 5F-MDMB-PINACA could also be detected in buffer sample, suggesting non-enzymatic transesterification.
Transesterification and hydrolysis activities of 5F-MMB-PINACA/5F-EMB-PINACA and MMB-FUBINACA/EMB-FUBINACA
Supplementary Figure S6 presents time-dependent transesterification and hydrolysis activities of 5F-MMB-PINACA and MMB-FUBINACA when co-incubated with ethanol. Likewise, the substrates showed rapid decline to a level below limit of detection after 15 min of incubation, and hydrolysis metabolites became the most abundant compound. Formation of the ethyl ester metabolites was almost negligible and eliminated after 15 min as well. These ethyl ester analogs were possibly transesterified time dependently as well in the presence of methanol (Supplementary Figure S6). Additionally, 5F-EMB-PINACA and 5F-MMB-PINACA were non-enzymatically generated in buffer sample without enzyme.
Discussion
In this study, we investigated the in vitro metabolism of three indazole-3-carboxamide type SCs in the presence and absence of alcohol and established the susceptibilities of their ethyl ester metabolites to non-enzymatic chemical formation, enzymatic hydrolysis by CES and enzymatic hydroxylation by P450. We showed that 5F-MDMB-PINACA, 5F-MMB-PINACA, and MMB-FUBINACA readily underwent transesterification and hydrolysis upon incubation with HLM in the presence of ethanol. We demonstrated that transesterification was catalyzed by CES with time- and ethanol concentration-dependency. Additionally, ethyl ester metabolites were amenable to further hydrolysis and several oxidative reactions, yielding substrate specificity which was comparable to the respective parent SCs.
Medications as well as alcohol were present in 82% of postmortem cases associated with the use of NPS (24). For SC-related fatalities, consumption and/or detection of ethanol was continuously reported (13–16). For example, a blood alcohol concentration (BAC) of 0.09 g/kg (0.11 µL/mL) and a femoral blood concentration of 5F-MDMB-PINACA at 0.38 ng/mL (4.8 × 107 µL/mL) were determined in one fatal case (13). In another case with 27 mg/dL (0.34 µL/mL) of ethanol in blood, both MMB-FUBINACA and EMB-FUBINACA were presented in multiple postmortem materials (25). While the phenomenon was explained as the co-administration of a mixture of SCs, the in vivo transesterification of MMB-FUBINACA to EMB-FUBINACA could be another possible clinical scenario as hinted by our findings.
Most SCs have poor metabolic stability and are susceptible to biotransformation in vitro and in vivo. Hence, the metabolite profiling of each parent SC and its ethyl ester metabolite in biological samples becomes critical for documenting its co-abuse with alcohol. A total of 12 phase I metabolites were previously detected for 5F-MDMB-PINACA in HLM incubation sample and the dominant biotransformation was hydrolysis of the ester group (3, 22). Additionally, the oxidative defluorination metabolite with the intact methyl ester moiety was among the five most abundant 5F-MDMB-PINACA metabolites (3, 22). Here, we postulated that the oxidative defluorination metabolite was the potential metabolite of 5F-EDMB-PINACA, and both oxidative defluorination metabolites could be derived in situ from the transesterification of parent 5F-MDMB-PINACA, which were corroboratively identified in our 5F-MDMB-PINACA incubation sample (Table I). This sequential metabolism in the presence of ethanol was in accordance with previous four SCs with quinolinyl ester moiety (17). The proposed metabolic pathway of 5F-MDMB-PINACA and 5F-EDMB-PINACA in the presence of alcohol is summarized in Figure 3.
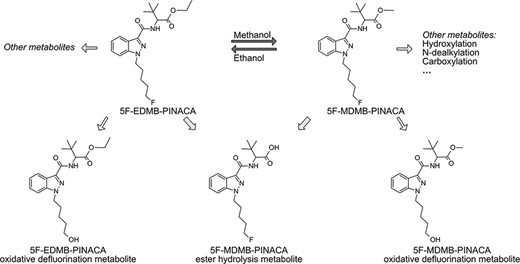
Proposed metabolic pathways for 5F-MDMB-PINACA and 5F-EDMB-PINACA in the presence of ethanol or methanol, respectively.
For 5F-MMB-PINACA and MMB-FUBINACA, the major biotransformation pathway was ester hydrolysis to form carboxylic acid as well, with further oxidation and glucuronidation yielding sequential phase I and II metabolites (8, 9, 23). Notably, their non-hydrolytic metabolites were produced at relatively lower abundances. Pentyl chain hydroxylated, pentanoic acid, and oxidative defluorination metabolites of 5F-MMB-PINACA were ranked as the last three abundant metabolites among its 17 identified metabolites and were only detected in the 10 min and 1 h samples (8). Likewise, hydroxylation and dehydrogenation metabolites were the 9th and 11th abundant for MMB-FUBINACA, respectively (9). The low intensities of these metabolites corroborated our preliminary findings. Consequently, in metabolite identification experiments, we incubated 5F-EMB-PINACA and EMB-FUBINACA with CES inhibitor BNPP to increase the production of non-hydrolytic metabolites for better characterization. Although several oxidative metabolites were generated as expected, they still could not be observed when the parent SC was incubated with ethanol, except for defluorobenzyl metabolite of EMB-FUBINACA, which was detected at a low level and verified by the relevant ions detected in EMB-FUBINACA sample (Table I). The low intensities of 5F-EMB-PINACA and EMB-FUBINACA in respective transesterification samples failed to trigger sequential parent ion fragmentation in MS analysis as well, and they were confirmed based on their precursor ion m/z and retention time (Table I).
Apirakkan et al. reported that oxidative metabolites were the metabolites of ethyl ester metabolites, which were derived from upstream parent SCs in the presence of alcohol. These sequential oxidative metabolites were proposed as biomarkers for forensic monitoring (17). Cocaethylene and ethylphenidate, the ethyl ester metabolites of cocaine and methylphenidate, were found to be substrates of both CES and P450, generating the identical hydrolysis metabolite benzoylecgonine and ritalinic acid, respectively, as well as specific oxidative metabolites (18, 19, 26, 27). Here, we hypothesized first that the ethyl ester metabolite of a given SC was amenable to hydrolysis reaction forming the same hydrolyzed metabolite as its parent SC, and second, the sequential oxidative metabolite of the ethyl ester metabolite could distinguish the source of the substrate. In this study, we have successfully proven these hypotheses based on our example of 5F-MDMB-PINACA (Table I).
Ethanol has been shown to inhibit CES-catalyzed hydrolysis in vitro and in vivo (28) and produce transesterified products of several substrates, e.g., cocaethylene, ethylphenidate and ethyl clopidogrel. This organic reaction could also occur in the absence of enzymes (17, 26), which was in accordance with our results that small amount of ethyl ester metabolite was detected in buffer samples without enzyme (Figure 2 and Supplementary Figure S6). When BNPP was added into incubation system to inhibit CES catalysis of 5F-MDMB-PINACA or 5F-EDMB-PINACA, the formation of the respective ethyl ester metabolite could still be observed but decreased to a similar level to that observed in the buffer control (Figure 2B and Supplementary Figure S5A). Additionally, both enzymatic and non-enzymatic transesterification demonstrated ethanol concentration-dependency (Figure 2C), which was consistent with previous finding (29). These results further confirmed that 5F-EDMB-PINACA and 5F-MDMB-PINACA were true transesterified metabolites of their respective substrates.
Cocaethylene underwent ethyl ester exchange, being converted to 2H5-cocaethylene in the presence of 2H6-ethanol (30). A series of 12 parabens with C1–C12 alkyl chain in ester moiety could be actively transesterified by CES when incubated with C1–C12 alcohols (31), suggesting the susceptibleness of the ester-to-ester transformation catalyzed by various alcohols. Similarly, the three ethyl ester metabolites in our study, 5F-EDMB-PINACA, 5F-EMB-PINACA, and EMB-FUBINACA, were found to produce their respective parent compound in the presence of methanol (Supplementary Figure S5B, S6B, and S6D).
A computational study suggested that both hydrolytic and transesterification reactions are favorable processes when the substrate is an ester and can be efficiently catalyzed by CES (32). While for dabigatran etexilate, a substrate with two ester bonds, the metabolites were not affected by ethanol in the incubation system at various concentrations up to 100 mM (5.8 µL/mL). Substrate specificity regarding transesterification and hydrolysis activities was similarly observed for our three SCs. In time-dependent experiments of 5F-MDMB-PINACA and 5F-EDMB-PINACA, enzymatically transesterified metabolites and the sequential oxidative defluorination metabolites could be continuously detected (Figure 2A, Supplementary Figure S5A). For 5F-MMB-PINACA/5F-EMB-PINACA and MMB-FUBINACA/EMB-FUBINACA, both pairs of substrates and ethyl ester metabolites became undetectable rapidly, and their oxidative metabolites were not detected as well (Supplementary Figure S6). Andersson et al. and our laboratory previously reported that the in vitro half-life (t1/2) and microsomal intrinsic clearance (in vitro CLint) of 5F-MDMB-PINACA and 5F-MMB-PINACA were 7.82 min, 0.18 mL/min/mg and 1.0 min, 0.67 mL/min/mg, respectively (8, 20). Different metabolic stabilities were also observed for the metabolites. For instance, the oxidative defluorination metabolite of 5F-MDMB-PINACA was detected in HLM after 3 h incubation (3), while the oxidative metabolites of 5F-MMB-PINACA were only found in the 10 min and 1 h samples at low intensity (8). Given the structural similarity, we posit that the metabolic stability profile of each parent SC is recapitulated in the respective ethyl ester metabolite, which partly explained the differences in results between 5F-MDMB-PINACA and the other two parent SCs (5F-MMB-PINACA and MMB-FUBINACA) for both the metabolite identification and time-dependent experiments. Our postulation is reasonable as the pharmacokinetics of cocaine and cocaethylene were shown to be comparable in dogs (19). Taken together, the metabolic stability profile of parent compound should be taken into consideration when evaluating transesterification reactions among different SC substrates and proposing sequential metabolites as potential biomarkers.
The metabolism of ethanol is predominantly mediated by cytosolic alcohol dehydrogenase (ADH) and aldehyde dehydrogenase (ALDH) in the liver (33). The HLM system does not contain these cytosolic enzymes. Consequently, the utilization of HLM did not recapitulate the metabolism of ethanol by ADH and ALDH in our static incubation experiments. To overcome this limitation, future studies should utilize human hepatocytes or liver S9 fractions to characterize the metabolic interplay between transesterification of ethanol and its ADH- and ALDH-mediated metabolism. Despite this limitation, our findings along with previous in vitro evidence, illuminated the potential formation of transesterified metabolites of SCs in the presence of detectable blood alcohol among SC consumers.
Apirakkan et al. observed dramatically reduced CB1 and CB2 activation for the ethyl ester metabolites of four SCs with quinolinyl ester moiety in the presence of ethanol (17). As the three ethyl ester metabolites in our study are also illicit drugs available in the market, their potency and efficacy at CB1 and CB2 have been reported except for 5F-EDMB-PINACA. Estimated half-maximal effective concentration (EC50) and maximal response (Emax) of three parent SCs, 5F-EMB-PINACA and EMB-FUBINACA from multiple literatures were summarized in Supplementary Table I. In brief, all the five SCs exhibited greater or similar potency at both CB1 and CB2 than the reference JWH-018 or CP55940 and functioned as high efficacy receptor agonists as expected. Comparable potency could also be observed between parent 5F-MMB-PINACA and its transesterified metabolite 5F-EMB-PINACA, suggesting potential augmentation of psychoactive and toxic effects of 5F-MMB-PINACA when it is consumed with alcohol. However, such comparison for the other two SCs based on the same assay was unavailable currently. Future potency testing incorporating both pairs of parent SCs and the ethyl ester metabolites should be explored to establish their relative pharmacological potencies.
Conclusions
Alcohol consumption is common amongst illicit and recreational drug users. Here, we have provided in vitro evidence of the CES-catalyzed formation of ethyl ester metabolites of three SCs in the presence of alcohol. The comparable pharmacological activities of the parent SCs and their ethyl ester metabolites could result in enhanced psychoactive effect. Although the consumption of a mixture of multiple SCs is of high prevalence, our study demonstrated that oxidative metabolites generated by ethyl ester metabolite could be investigated as the biomarker for diagnosing the potential co-abuse of specific SC and alcohol, as exemplified by the 5F-EDMB-PINACA oxidative defluorination metabolite of 5F-MDMB-PINACA.
Supplementary Data
Supplementary Data is available at Journal of Analytical Toxicology online.
Acknowledgments
The authors would like to acknowledge the support from Health Sciences Authority (HSA) of Singapore.
Funding
This work was supported by the National University of Singapore (NUS) Postdoctoral Fellowship awarded to Ziteng Wang.
Data availability
The data underlying this article will be shared on reasonable request to the corresponding author.