-
PDF
- Split View
-
Views
-
Cite
Cite
Svante Vikingsson, Shannon T Krauss, Ruth E Winecker, Ronald R Flegel, Eugene D Hayes, Update on Urine Adulterants and Synthetic Urine Samples to Subvert Urine Drug Testing, Journal of Analytical Toxicology, Volume 46, Issue 7, September 2022, Pages 697–704, https://doi.org/10.1093/jat/bkac029
- Share Icon Share
Abstract
To avoid a positive urine drug test, donors might try to subvert the test, either by adulterating the specimen with a product designed to interfere with testing or by substituting the specimen for a synthetic urine. A market search conducted in December of 2020 identified 3 adulterants and 32 synthetic urines, and a selection was procured based on specific criteria. Samples prepared with the 3 adulterants and 10 synthetic urines were submitted for testing at five forensic drug testing laboratories to perform immunoassay screening, chromatographic confirmation analysis and specimen validity testing (SVT). One adulterant determined to contain iodate reduced THC-COOH concentrations by 65% and the concentrations of 6-acetylmorphine, morphine, oxycodone, oxymorphone, hydrocodone and hydromorphone by 6–27%. Another adulterant determined to contain nitrite reduced THC-COOH concentrations by 22%, while the third did not affect drug screening or confirmatory testing. Both active adulterants could be identified through positive oxidant screens as well as through signal suppression in cloned enzyme donor immunoassay (CEDIA). The synthetic urines could not be identified either through traditional SVT or by the AdultaCheck10 dipstick. The Synthetic UrineCheck dipstick produced a difference in response between the authentic urine specimen and the synthetic urine samples, but the difference was small and difficult to observe. While most synthetic urines now contain uric acid, magnesium and caffeine, the results indicated that a biomarker panel including endogenous and exogenous markers of authentic urine performed well and clearly demonstrated the absence of biomarkers in the synthetic urines. The SVT assay also offers potential targets for future screening assays.
Introduction
In a recent report from the Government Accountability Office, it was estimated that 19% of the U.S. population misused drugs in 2018 (1). To address the safety risks of drug use in the workplace, there are several federally regulated workplace drug testing programs, including testing of federal employees, and various employees in transportation sector and at nuclear power plants. The Department of Health and Human Services (HHS) is tasked with certifying (accrediting) and overseeing laboratories performing federally regulated workplace drug testing, which it does through the Substance Abuse and Mental Health Services Administration and the National Laboratory Certification Program. This program is considered a best practice model, forming the basis for most corporate drug testing programs.
At the core of workplace drug testing in urine are the Mandatory Guidelines for Federal Workplace Drug Testing Programs in Urine (2) that regulate all aspects of urinary testing, including specimen collection, the scope of testing and reporting. According to the guidelines, a donor must be given visual privacy when providing the sample (except under certain circumstances). This unfortunately also offers the donor some opportunity to either substitute their samples with a different solution or adulterate the sample by adding a chemical to interfere with the testing and/or react with drugs present in the sample (3).
Analysis of drugs in biological matrices, and especially the immunoassays used for the screening of urine specimens, works best under physiological conditions. The general idea behind many adulteration products is to change these conditions to create a false negative result in the screening (4) and/or to chemically modify the analytes, creating a chemical compound not detected by the assay (5). Initially, household chemicals such as soap, bleach and vinegar were used for adulteration. However, over the last few decades, adulterants have evolved and now include oxidants (nitrite, pyridinium chlorochromate, peroxidase/peroxide) and glutaraldehyde (6). Adulteration has mainly been identified by specimen validity testing (SVT), which typically includes visual inspection, pH, creatinine analyses and a screen for oxidative chemicals. Adulterants can also interfere with immunoassays, producing abnormal results (2).
To substitute a urine sample with a different solution, the donor must first smuggle the solution into the restroom (7). The donor must also ensure that the solution is visually similar to urine and is at body temperature (8). Both of these qualities will be checked by the collector before sending the specimen to the laboratory (2). Early substitution attempts included household products similar to urine (e.g., apple juice or beer) or urine donated by a friend or pet. However, the use of synthetic urines has become more common. Synthetic urines are mixes of urine constituents such as urea, creatinine, dyes and protein, designed to be similar in appearance and odor to urine and produce results consistent with unadulterated urine specimens collected from donors (authentic urine) in SVT assays (9). In a recent study by Kim et al. (10), eight synthetic urines were analyzed. One synthetic urine was identified as diluted due to low creatinine and specific gravity, while the other seven were reported as consistent with authentic urine. In addition, the authors reported that the Sciteck Synthetic UrineCheck dipstick could be used to identify synthetic urines, although the dipsticks could also falsely identify dilute specimens as synthetic urine.
The aim of this study was to survey the market for readily available adulterants and synthetic urines and evaluate their performance in regulated workplace drug testing. Additionally, the ability of alternative SVT assays, not required by the Mandatory Guidelines for Federal Workplace Drug Testing Programs in Urine (2), to identify synthetic urines was investigated.
Materials and Methods
A market survey was conducted, and based on the results, a selection of adulterants and synthetic urines were procured. Based on preliminary testing, samples prepared using 3 adulterants and 10 synthetic urines were tested by five forensic drug testing laboratories.
Market survey
In December 2020, a comprehensive market survey was conducted using the GoogleTM search engine to identify products sold as synthetic urines or urine adulterants. The search terms ‘urine adulterant’, ‘urine additive’, ‘synthetic urine’, ‘clean urine’, ‘fake urine’, ‘urine detox’ and ‘Creatine ethyl ester’ were used and subsequent links and referrals from those pages followed.
Based on the results of the survey, 3 adulterants and 16 synthetic urines were procured for testing. The 16 synthetic urines were selected to include products with differing manufacturers and costs. Additionally, efforts were made to avoid ordering duplicate products sold with different branding.
Preparation of adulterated samples
Adulterated samples were prepared using a commercial negative urine (UTAK, Valencia, CA), which contained a small amount of sodium azide for preservation. Negative adulterated samples were prepared according to the instructions accompanying the adulterants.
Positive adulterated samples were prepared in a pool of negative urine fortified with HHS regulated drug analytes at approximately 2× the initial test cutoffs when considering cross-reacting analytes. 6-Acetylmorphine was fortified at 20 ng/mL; phencyclidine (PCP) at 50 ng/mL; ∆9-tetrahydrocannabinol-9-carboxylic acid (∆9-THC-COOH), oxycodone and oxymorphone at 100 ng/mL; benzoylecgonine, hydrocodone and hydromorphone at 300 ng/mL; methamphetamine and amphetamine at 500 ng/mL; codeine at 2,000 ng/mL; and morphine at 2,310 ng/mL. A positive control urine without adulterant was also included. All analytes were obtained from Cerilliant (Round Rock, TX).
Testing methods
All samples underwent preliminary testing, including appearance, the AdultaCheck10 dipstick (Sciteck, Fletcher, NJ) and the Synthetic UrineCheck dipstick (Sciteck). Dipsticks were photographed together with the manufacturer-provided color guide, and the results were determined visually. For the Synthetic UrineCheck, the color of the dipstick pad was extracted from the photographs and converted to an arbitrary one-dimensional color intensity, or saturation value, based on the colors of the manufacturer’s color guide. This allowed for a more detailed and objective assessment of the subtle color differences.
Based on preliminary testing, samples were selected for further testing. All adulterated samples as well as 10 synthetic urines were submitted for testing to five forensic drug testing laboratories selected to provide a mix of screening and confirmation methods, as seen in Table I.
Initial screening methods . | ||||||||
---|---|---|---|---|---|---|---|---|
. | ∆9-THC-COOH . | BZE . | MOR . | HYC . | OXYC . | 6AM . | PCP . | MAMP . |
Lab A | Thermo DRI | Thermo DRI | Thermo DRI | Thermo DRI | Thermo DRI | Thermo CEDIA | Thermo DRI | Thermo DRI |
Lab B | Roche KIMS | Roche KIMS | Roche KIMS | Thermo DRI | Thermo DRI | Thermo CEDIA | Roche KIMS | Thermo CEDIA |
Lab C | Thermo DRI | Thermo DRI | Thermo DRI | Thermo DRI | Thermo DRI | Thermo CEDIA | Thermo DRI | Thermo CEDIA |
Lab D | Thermo DRI | Thermo DRI | Thermo DRI | Thermo DRI | Thermo DRI | Thermo CEDIA | Thermo DRI | Thermo DRI |
Lab E | Thermo DRI | Thermo DRI | Thermo DRI | Thermo DRI | Thermo DRI | Immunalysis | Thermo DRI | Thermo DRI |
Confirmatory methods | ||||||||
∆9-THC-COOH | BZE | MOR/ COD | HYC/ HYM | OXYC/ OXYM | 6AM | PCP | MAMP/ AMP | |
Lab A | GC–MS Base hyd. SPE BSTFA | GC–MS SPE HFIP/HFBA | LC−MS-MS Enz. hyd. No ext. | LC–MS-MS Enz. hyd. No ext. | LC–MS-MS Enz. hyd. No ext. | GC–MS SPE MTBSTFA | GC–MS SPE No der. | GC–MS LIQ/LIQ HFBA |
Lab B | GC–MS Base hyd. LIQ/LIQ TBDMS | GC–MS LIQ/LIQ BSTFA | GC–MS Enz. hyd. LIQ/LIQ BSTFA | LC–MS-MS Enz. hyd. LIQ/LIQ | LC–MS-MS Enz. hyd. LIQ/LIQ | GC–MS LIQ/LIQ BSTFA | GC–MS LIQ/LIQ No der. | GC–MS LIQ/LIQ HFBA |
Lab C | GC–MS Base hyd. LIQ/LIQ BSTFA | GC–MS SPE PFAA | LC–MS-MS Enz. Hyd. No ext. | LC–MS-MS Enz. Hyd. No ext. | LC–MS-MS No ext. | GC–MS LIQ/LIQ No der. | GC–MS SPE MBTFA | |
Lab D | LC–MS-MS Base hyd. No ext. | LC–MS-MS No ext. | LC–MS-MS Enz. hyd. LIQ/LIQ | LC–MS-MS Enz. hyd. LIQ/LIQ | LC–MS-MS Enz. hyd. LIQ/LIQ | LC–MS-MS No ext. | LC–MS-MS No ext. | LC–MS-MS No ext. |
Lab E | GC–MS Base hyd. LIQ/LIQ MTBSTFA | GC–MS LIQ/LIQ HFIP/PFAA | GC–MS LIQ/LIQ HFIP/PFAA | GC–MS Enz. hyd. LIQ/LIQ PA | GC–MS Enz. hyd. LIQ/LIQ PA | GC–MS LIQ/LIQ PFAA | GC–MS LIQ/LIQ No der. | GC–MS LIQ/LIQ PFAA |
Initial screening methods . | ||||||||
---|---|---|---|---|---|---|---|---|
. | ∆9-THC-COOH . | BZE . | MOR . | HYC . | OXYC . | 6AM . | PCP . | MAMP . |
Lab A | Thermo DRI | Thermo DRI | Thermo DRI | Thermo DRI | Thermo DRI | Thermo CEDIA | Thermo DRI | Thermo DRI |
Lab B | Roche KIMS | Roche KIMS | Roche KIMS | Thermo DRI | Thermo DRI | Thermo CEDIA | Roche KIMS | Thermo CEDIA |
Lab C | Thermo DRI | Thermo DRI | Thermo DRI | Thermo DRI | Thermo DRI | Thermo CEDIA | Thermo DRI | Thermo CEDIA |
Lab D | Thermo DRI | Thermo DRI | Thermo DRI | Thermo DRI | Thermo DRI | Thermo CEDIA | Thermo DRI | Thermo DRI |
Lab E | Thermo DRI | Thermo DRI | Thermo DRI | Thermo DRI | Thermo DRI | Immunalysis | Thermo DRI | Thermo DRI |
Confirmatory methods | ||||||||
∆9-THC-COOH | BZE | MOR/ COD | HYC/ HYM | OXYC/ OXYM | 6AM | PCP | MAMP/ AMP | |
Lab A | GC–MS Base hyd. SPE BSTFA | GC–MS SPE HFIP/HFBA | LC−MS-MS Enz. hyd. No ext. | LC–MS-MS Enz. hyd. No ext. | LC–MS-MS Enz. hyd. No ext. | GC–MS SPE MTBSTFA | GC–MS SPE No der. | GC–MS LIQ/LIQ HFBA |
Lab B | GC–MS Base hyd. LIQ/LIQ TBDMS | GC–MS LIQ/LIQ BSTFA | GC–MS Enz. hyd. LIQ/LIQ BSTFA | LC–MS-MS Enz. hyd. LIQ/LIQ | LC–MS-MS Enz. hyd. LIQ/LIQ | GC–MS LIQ/LIQ BSTFA | GC–MS LIQ/LIQ No der. | GC–MS LIQ/LIQ HFBA |
Lab C | GC–MS Base hyd. LIQ/LIQ BSTFA | GC–MS SPE PFAA | LC–MS-MS Enz. Hyd. No ext. | LC–MS-MS Enz. Hyd. No ext. | LC–MS-MS No ext. | GC–MS LIQ/LIQ No der. | GC–MS SPE MBTFA | |
Lab D | LC–MS-MS Base hyd. No ext. | LC–MS-MS No ext. | LC–MS-MS Enz. hyd. LIQ/LIQ | LC–MS-MS Enz. hyd. LIQ/LIQ | LC–MS-MS Enz. hyd. LIQ/LIQ | LC–MS-MS No ext. | LC–MS-MS No ext. | LC–MS-MS No ext. |
Lab E | GC–MS Base hyd. LIQ/LIQ MTBSTFA | GC–MS LIQ/LIQ HFIP/PFAA | GC–MS LIQ/LIQ HFIP/PFAA | GC–MS Enz. hyd. LIQ/LIQ PA | GC–MS Enz. hyd. LIQ/LIQ PA | GC–MS LIQ/LIQ PFAA | GC–MS LIQ/LIQ No der. | GC–MS LIQ/LIQ PFAA |
6AM, 6-acetylmorphine; ∆9-THC-COOH, ∆9-tetrahydrocannabinol-9-carboxylic acid; AMP, amphetamine; BSTFA, bis(trimethylsilyl)trifluoroacetamide; BZE, benzoylecgonine; COD, codeine; der, derivatization; enz, enzyme; ext, extraction; HFBA, heptafluorobutyric anhydride; HFIP, hexafluoroisopropanol; HYC, hydrocodone; hyd, hydrolysis; HYM, hydromorphone; KIMS, kinetic interaction of microparticle in solution; LIQ/LIQ, liquid−liquid extraction; MAMP, methamphetamine; MBTFA, methyl-bis-trifluoroacetamide; MOR, morphine; MTBSTFA, methyl-tert-butyldimethylsilyltrifluoroacetamide; OXYC, oxycodone; OXYM, oxymorphone; PA, propionic anhydride; PFAA, pentafluoropropionic acid anhydride; SPE, solid phase extraction; TBDMS, tert-butyldimethylsilyl.
Initial screening methods . | ||||||||
---|---|---|---|---|---|---|---|---|
. | ∆9-THC-COOH . | BZE . | MOR . | HYC . | OXYC . | 6AM . | PCP . | MAMP . |
Lab A | Thermo DRI | Thermo DRI | Thermo DRI | Thermo DRI | Thermo DRI | Thermo CEDIA | Thermo DRI | Thermo DRI |
Lab B | Roche KIMS | Roche KIMS | Roche KIMS | Thermo DRI | Thermo DRI | Thermo CEDIA | Roche KIMS | Thermo CEDIA |
Lab C | Thermo DRI | Thermo DRI | Thermo DRI | Thermo DRI | Thermo DRI | Thermo CEDIA | Thermo DRI | Thermo CEDIA |
Lab D | Thermo DRI | Thermo DRI | Thermo DRI | Thermo DRI | Thermo DRI | Thermo CEDIA | Thermo DRI | Thermo DRI |
Lab E | Thermo DRI | Thermo DRI | Thermo DRI | Thermo DRI | Thermo DRI | Immunalysis | Thermo DRI | Thermo DRI |
Confirmatory methods | ||||||||
∆9-THC-COOH | BZE | MOR/ COD | HYC/ HYM | OXYC/ OXYM | 6AM | PCP | MAMP/ AMP | |
Lab A | GC–MS Base hyd. SPE BSTFA | GC–MS SPE HFIP/HFBA | LC−MS-MS Enz. hyd. No ext. | LC–MS-MS Enz. hyd. No ext. | LC–MS-MS Enz. hyd. No ext. | GC–MS SPE MTBSTFA | GC–MS SPE No der. | GC–MS LIQ/LIQ HFBA |
Lab B | GC–MS Base hyd. LIQ/LIQ TBDMS | GC–MS LIQ/LIQ BSTFA | GC–MS Enz. hyd. LIQ/LIQ BSTFA | LC–MS-MS Enz. hyd. LIQ/LIQ | LC–MS-MS Enz. hyd. LIQ/LIQ | GC–MS LIQ/LIQ BSTFA | GC–MS LIQ/LIQ No der. | GC–MS LIQ/LIQ HFBA |
Lab C | GC–MS Base hyd. LIQ/LIQ BSTFA | GC–MS SPE PFAA | LC–MS-MS Enz. Hyd. No ext. | LC–MS-MS Enz. Hyd. No ext. | LC–MS-MS No ext. | GC–MS LIQ/LIQ No der. | GC–MS SPE MBTFA | |
Lab D | LC–MS-MS Base hyd. No ext. | LC–MS-MS No ext. | LC–MS-MS Enz. hyd. LIQ/LIQ | LC–MS-MS Enz. hyd. LIQ/LIQ | LC–MS-MS Enz. hyd. LIQ/LIQ | LC–MS-MS No ext. | LC–MS-MS No ext. | LC–MS-MS No ext. |
Lab E | GC–MS Base hyd. LIQ/LIQ MTBSTFA | GC–MS LIQ/LIQ HFIP/PFAA | GC–MS LIQ/LIQ HFIP/PFAA | GC–MS Enz. hyd. LIQ/LIQ PA | GC–MS Enz. hyd. LIQ/LIQ PA | GC–MS LIQ/LIQ PFAA | GC–MS LIQ/LIQ No der. | GC–MS LIQ/LIQ PFAA |
Initial screening methods . | ||||||||
---|---|---|---|---|---|---|---|---|
. | ∆9-THC-COOH . | BZE . | MOR . | HYC . | OXYC . | 6AM . | PCP . | MAMP . |
Lab A | Thermo DRI | Thermo DRI | Thermo DRI | Thermo DRI | Thermo DRI | Thermo CEDIA | Thermo DRI | Thermo DRI |
Lab B | Roche KIMS | Roche KIMS | Roche KIMS | Thermo DRI | Thermo DRI | Thermo CEDIA | Roche KIMS | Thermo CEDIA |
Lab C | Thermo DRI | Thermo DRI | Thermo DRI | Thermo DRI | Thermo DRI | Thermo CEDIA | Thermo DRI | Thermo CEDIA |
Lab D | Thermo DRI | Thermo DRI | Thermo DRI | Thermo DRI | Thermo DRI | Thermo CEDIA | Thermo DRI | Thermo DRI |
Lab E | Thermo DRI | Thermo DRI | Thermo DRI | Thermo DRI | Thermo DRI | Immunalysis | Thermo DRI | Thermo DRI |
Confirmatory methods | ||||||||
∆9-THC-COOH | BZE | MOR/ COD | HYC/ HYM | OXYC/ OXYM | 6AM | PCP | MAMP/ AMP | |
Lab A | GC–MS Base hyd. SPE BSTFA | GC–MS SPE HFIP/HFBA | LC−MS-MS Enz. hyd. No ext. | LC–MS-MS Enz. hyd. No ext. | LC–MS-MS Enz. hyd. No ext. | GC–MS SPE MTBSTFA | GC–MS SPE No der. | GC–MS LIQ/LIQ HFBA |
Lab B | GC–MS Base hyd. LIQ/LIQ TBDMS | GC–MS LIQ/LIQ BSTFA | GC–MS Enz. hyd. LIQ/LIQ BSTFA | LC–MS-MS Enz. hyd. LIQ/LIQ | LC–MS-MS Enz. hyd. LIQ/LIQ | GC–MS LIQ/LIQ BSTFA | GC–MS LIQ/LIQ No der. | GC–MS LIQ/LIQ HFBA |
Lab C | GC–MS Base hyd. LIQ/LIQ BSTFA | GC–MS SPE PFAA | LC–MS-MS Enz. Hyd. No ext. | LC–MS-MS Enz. Hyd. No ext. | LC–MS-MS No ext. | GC–MS LIQ/LIQ No der. | GC–MS SPE MBTFA | |
Lab D | LC–MS-MS Base hyd. No ext. | LC–MS-MS No ext. | LC–MS-MS Enz. hyd. LIQ/LIQ | LC–MS-MS Enz. hyd. LIQ/LIQ | LC–MS-MS Enz. hyd. LIQ/LIQ | LC–MS-MS No ext. | LC–MS-MS No ext. | LC–MS-MS No ext. |
Lab E | GC–MS Base hyd. LIQ/LIQ MTBSTFA | GC–MS LIQ/LIQ HFIP/PFAA | GC–MS LIQ/LIQ HFIP/PFAA | GC–MS Enz. hyd. LIQ/LIQ PA | GC–MS Enz. hyd. LIQ/LIQ PA | GC–MS LIQ/LIQ PFAA | GC–MS LIQ/LIQ No der. | GC–MS LIQ/LIQ PFAA |
6AM, 6-acetylmorphine; ∆9-THC-COOH, ∆9-tetrahydrocannabinol-9-carboxylic acid; AMP, amphetamine; BSTFA, bis(trimethylsilyl)trifluoroacetamide; BZE, benzoylecgonine; COD, codeine; der, derivatization; enz, enzyme; ext, extraction; HFBA, heptafluorobutyric anhydride; HFIP, hexafluoroisopropanol; HYC, hydrocodone; hyd, hydrolysis; HYM, hydromorphone; KIMS, kinetic interaction of microparticle in solution; LIQ/LIQ, liquid−liquid extraction; MAMP, methamphetamine; MBTFA, methyl-bis-trifluoroacetamide; MOR, morphine; MTBSTFA, methyl-tert-butyldimethylsilyltrifluoroacetamide; OXYC, oxycodone; OXYM, oxymorphone; PA, propionic anhydride; PFAA, pentafluoropropionic acid anhydride; SPE, solid phase extraction; TBDMS, tert-butyldimethylsilyl.
The laboratories also conducted SVT testing as detailed in Supplementary Table S1 Creatinine was determined by the Jaffe assay (Thermo Scientific, Waltham, MA), specific gravity by 4-place refractometer (Rudolph, Hackettstown, NJ), pH by pH meter (various) and oxidant activity by colorimetric assay (Thermo Scientific or Siemens Medical Solutions, Malvern, PA) and, in some laboratories, ion chromatography (Thermo Scientific).
Alternative SVT
One of the five laboratories analyzed the adulterated samples and the synthetic urines with two additional SVT assays to evaluate their performance in discriminating authentic urine from synthetic urine. In the first assay, uric acid and magnesium were measured by colorimetry. In the second assay, a seven-analyte biomarker panel was analyzed by liquid chromatography tandem mass spectrometry (LC-MS-MS). The panel was based on previous research (11) and included uric acid, caffeine, methylhistidine, theophylline, normetanephrine, urobilin and theobromine.
Results
By preparing samples and submitting them for testing at forensic drug testing laboratories, the ability of three adulterants to interfere with assays typically used for screening and confirmation of drugs in urine was evaluated. In addition, the ability of traditional and alternative SVT assays to identify adulterated and synthetic urine was evaluated.
Market survey
In the market survey, 32 synthetic urine products were identified. Most were pre-mixed (n = 23), but dehydrated powders (n = 7) and concentrates (n = 2) were also identified. The volumes provided, or produced when rehydrated, ranged from 60 to 120 mL, and most products were priced between $25 and $50 (Q1−Q3, range $2.99 to $100). With respect to composition, several products claimed to contain urea and/or uric acid, and two products were marketed as authentic donor urine (S14 and S15).
Only three adulterants were identified, and one of them (A03) came with a warning stating that ‘…most testing facilities are detecting this product, …’. The adulterants were formulated as a single liquid (A01), two liquids to be mixed in the sample (A02) or as crystals to be dissolved in the sample (A03). Prices ranged from $15 for A03 to $125 for A01.
Based on the survey, 16 synthetic urines and all adulterants were procured. A mixture of pre-mixed, powdered and concentrated synthetic urines was selected, as seen in Table II. It was noted that for most products, the packaging and instructions were professional and similar to what can be expected from other consumer products.
Type . | ID numbers . |
---|---|
Pre-mixed | S02, S04, S07, S08, S10b, S12, S13b, S15c |
Concentrate | S03, S11 |
Powdered | S01a, S05, S06, S09, S14c |
Type . | ID numbers . |
---|---|
Pre-mixed | S02, S04, S07, S08, S10b, S12, S13b, S15c |
Concentrate | S03, S11 |
Powdered | S01a, S05, S06, S09, S14c |
Heat powder added.
Plastic bottles for S10 and S13 were identical and had identical lot numbers.
Producer claims this is authentic human urine.
Type . | ID numbers . |
---|---|
Pre-mixed | S02, S04, S07, S08, S10b, S12, S13b, S15c |
Concentrate | S03, S11 |
Powdered | S01a, S05, S06, S09, S14c |
Type . | ID numbers . |
---|---|
Pre-mixed | S02, S04, S07, S08, S10b, S12, S13b, S15c |
Concentrate | S03, S11 |
Powdered | S01a, S05, S06, S09, S14c |
Heat powder added.
Plastic bottles for S10 and S13 were identical and had identical lot numbers.
Producer claims this is authentic human urine.
Detection and quantification of drugs in adulterated samples
As seen in Supplementary Table S2, drug concentrations in the positive control urine were between 93% and 106% of the intended targets, making the concentrations between 1.89× and 2.20× the initial cutoffs used by the laboratories.
The screening results for the adulterated specimens are included in Table III. For samples adulterated by A01 and A03, all laboratories reported positive results for all drugs, indicating a limited effect, if any, of the adulterants. However, for A02, all laboratories using CEDIA assays for 6-acetylmorphine and methamphetamine reported interferences, which could be an important tool to identify this particular adulterant. In addition, two laboratories reported negative results for ∆9-THC-COOH in samples adulterated by A02, which is supported by 65% lower concentrations of THC-COOH in confirmation testing (Figure 1). It is also worth pointing out that both laboratories also reported interference in the CEDIA assay, allowing the sample to be identified as invalid.
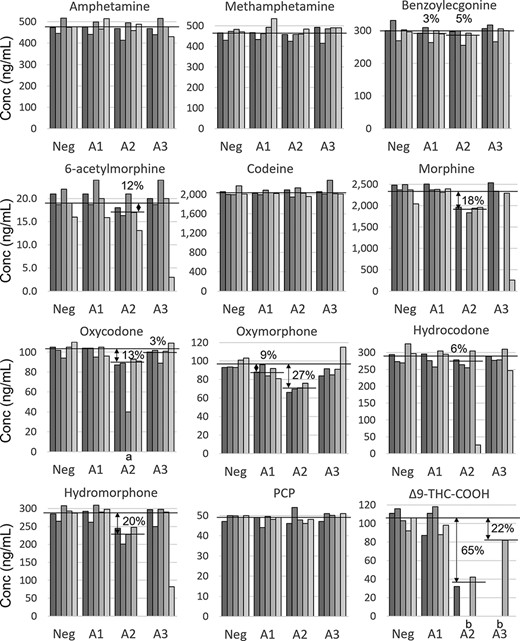
Confirmation results for adulterated samples. Each bar represents an individual result from a laboratory and shading from dark to light represents laboratories A−E. The horizontal lines represent mean concentrations in the unadulterated control (NEG), and the percentage loss is provided for significant losses compared to NEG (t-test). Missing bars represent interference. (a) Reported as negative by laboratory C, indicating a concentration <40 ng/mL. (b) For THC-COOH, there were not enough data points to perform t-test.
. | ∆9-THC-COOH . | BZE . | MOR . | HYC . | OXYC . | 6AM . | PCP . | MAMP . |
---|---|---|---|---|---|---|---|---|
Ctrl Pos | Pos: All | Pos: All | Pos: All | Pos: All | Pos: All | Pos: all | Pos: All | Pos: All |
A1 Pos | Pos: All | Pos: All | Pos: All | Pos: All | Pos: All | Pos: all | Pos: All | Pos: All |
A2 Pos | Pos: A, C, E Neg: B, D | Pos: All | Pos: All | Pos: All | Pos: All | Pos: E Int: A, Ba, C, D | Pos: All | Pos: A, D, E Int: Ba, C |
A3 Pos | Pos: All | Pos: All | Pos: All | Pos: All | Pos: All | Pos: all | Pos: All | Pos: All |
. | ∆9-THC-COOH . | BZE . | MOR . | HYC . | OXYC . | 6AM . | PCP . | MAMP . |
---|---|---|---|---|---|---|---|---|
Ctrl Pos | Pos: All | Pos: All | Pos: All | Pos: All | Pos: All | Pos: all | Pos: All | Pos: All |
A1 Pos | Pos: All | Pos: All | Pos: All | Pos: All | Pos: All | Pos: all | Pos: All | Pos: All |
A2 Pos | Pos: A, C, E Neg: B, D | Pos: All | Pos: All | Pos: All | Pos: All | Pos: E Int: A, Ba, C, D | Pos: All | Pos: A, D, E Int: Ba, C |
A3 Pos | Pos: All | Pos: All | Pos: All | Pos: All | Pos: All | Pos: all | Pos: All | Pos: All |
Lab B reported only which screens were positive for this sample. For MAMP and 6AM, interference was inferred due to a marked lower signal than for other samples. 6AM, 6-acetylmorphine; ∆9-THC-COOH, ∆9-tetrahydrocannabinol-9-carboxylic acid; BZE, benzoylecgonine; HYC, hydrocodone; Int, interference; MAMP, methamphetamine; MOR, morphine; Neg, negative; OXYC, oxycodone; Pos, positive.
. | ∆9-THC-COOH . | BZE . | MOR . | HYC . | OXYC . | 6AM . | PCP . | MAMP . |
---|---|---|---|---|---|---|---|---|
Ctrl Pos | Pos: All | Pos: All | Pos: All | Pos: All | Pos: All | Pos: all | Pos: All | Pos: All |
A1 Pos | Pos: All | Pos: All | Pos: All | Pos: All | Pos: All | Pos: all | Pos: All | Pos: All |
A2 Pos | Pos: A, C, E Neg: B, D | Pos: All | Pos: All | Pos: All | Pos: All | Pos: E Int: A, Ba, C, D | Pos: All | Pos: A, D, E Int: Ba, C |
A3 Pos | Pos: All | Pos: All | Pos: All | Pos: All | Pos: All | Pos: all | Pos: All | Pos: All |
. | ∆9-THC-COOH . | BZE . | MOR . | HYC . | OXYC . | 6AM . | PCP . | MAMP . |
---|---|---|---|---|---|---|---|---|
Ctrl Pos | Pos: All | Pos: All | Pos: All | Pos: All | Pos: All | Pos: all | Pos: All | Pos: All |
A1 Pos | Pos: All | Pos: All | Pos: All | Pos: All | Pos: All | Pos: all | Pos: All | Pos: All |
A2 Pos | Pos: A, C, E Neg: B, D | Pos: All | Pos: All | Pos: All | Pos: All | Pos: E Int: A, Ba, C, D | Pos: All | Pos: A, D, E Int: Ba, C |
A3 Pos | Pos: All | Pos: All | Pos: All | Pos: All | Pos: All | Pos: all | Pos: All | Pos: All |
Lab B reported only which screens were positive for this sample. For MAMP and 6AM, interference was inferred due to a marked lower signal than for other samples. 6AM, 6-acetylmorphine; ∆9-THC-COOH, ∆9-tetrahydrocannabinol-9-carboxylic acid; BZE, benzoylecgonine; HYC, hydrocodone; Int, interference; MAMP, methamphetamine; MOR, morphine; Neg, negative; OXYC, oxycodone; Pos, positive.
Adulterant A01 had a limited effect on confirmatory testing, and the only observed effect was 3% and 9% reductions for benzoylecgonine and oxymorphone, respectively (Figure 1). Similar reductions were also observed for benzoylecgonine (5%) with A02 and for oxycodone (3%) with A03. The P values ranged between 0.02 and 0.05, indicating that they might be coincidences rather than effects of the adulterants. For adulterant A03, the only observed reduction was for THC-COOH (22%).
With adulterant A02, reductions in concentration (6–27%) were observed for six of the opioids (6-acetylmorphine, morphine, oxycodone, oxymorphone, hydrocodone and hydromorphone) as well as 65% reduction for THC-COOH (Figure 1). In addition, concentrations ranging from 9% to 29% of the group mean were observed for 6-acetylmorphine, morphine, hydrocodone and hydromorphone in single samples adulterated with A02 or A03 (Figure 1 and Supplementary Table S2). The reason for this is unknown.
In addition to concentration changes, interferences precluded quantification of some analytes in samples adulterated by A02 when analyzed by labs A-D (Figure 1). When specified, the interferences were mostly related to poor recovery of the internal standards.
SVT
Both adulterated samples and the synthetic urines were tested by standard SVT assays, including pH, specific gravity, oxidants/nitrites and, in one laboratory, iodate (Supplementary Table S3). While samples adulterated by A01 produced normal SVT results in all laboratories, no effects on drug screening or confirmation results were observed.
Adulterant A02 contained iodate, which was confirmed by ion chromatography. In addition, the adulterant also changed the color of the urine (Figure 2) and generated positive results in the Siemens general oxidant assay consistent with the cross-reactivity reported in the package insert (12). The corresponding Thermo Scientific assay did not produce positive results, nor did a nitrite confirmation assay based on ion chromatography.
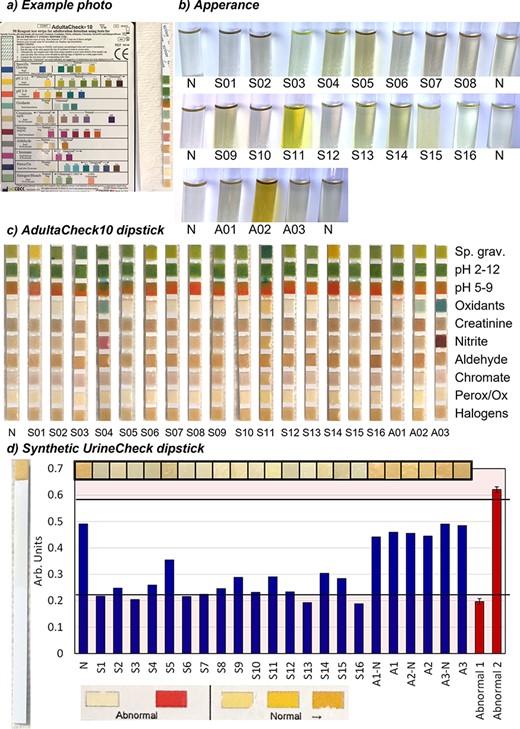
In-house specimen validity testing. (a) Example of dipstick photograph. (b) Appearance. N, control urine. (c) Adultacheck10 results. (d) Synthetic UrineCheck results. Bars represent color intensity, or saturation, as extracted from photographs of dipstick pads or manufacturer’s color guide. Black lines represent the 99.7% confidence interval for from bars representing abnormal results. Perox/Ox, peroxidase; Sp. Grav., specific gravity.
Finally, adulterant A03 contained nitrite as confirmed by ion chromatography. The specimen also generated positive results in oxidant screening assays in all laboratories.
The synthetic urines generally performed well in the SVT assays and 8 out of 10 products sent to the laboratories generated results consistent with an authentic urine specimen (Figure 2 and Supplementary Table S3). For sample S01, which was a powdered urine, a high specific gravity was observed, and nitrite was confirmed by ion chromatography (general oxidant screens were negative). It is possible that the findings are connected to the heat-generating powder that was added to the urine to achieve body temperature. The powder was not added to the pre-mixed version of the same urine (S02) which had normal results. In addition, a low specific gravity and a slight green tint were observed for synthetic urine S11 (Figure 2b).
The AdultaCheck10 dipsticks allowed a quick screening for aldehydes, chromate and peroxidase (Figure 2a and c). The dipsticks correctly identified nitrite in A03 and detected an oxidant, but not halogens, in A03, which was adulterated with iodate. The results of the Synthetic UrineCheck dipsticks are shown in Figure 2d. While the pads from the synthetic urines were lighter in color than those from the authentic urine specimens (N and A01-03), the difference might not be substantial enough to allow analysts to make confident determinations. In addition, all authentic urine samples in this study were from the same source, and the variability in the population is not represented.
The results of alternative SVT assays (uric acid, magnesium and the biomarker panel) are summarized in Table IV. Uric acid was identified in all synthetic urine samples except two and magnesium in 7 out of 10. Among the other biomarkers, detection was sparse and limited to caffeine (6/10) and methylhistidine (3/10).
. | Ctrl . | S1 . | S2 . | S4 . | S5 . | S6 . | S7 . | S11 . | S12 . | S14 . | S15 . |
---|---|---|---|---|---|---|---|---|---|---|---|
Colorimetric assay | |||||||||||
Uric acid | 17.3 | 3.4 | 4.0 | <1 | 5.4 | 3.3 | 6.5 | <1 | 3.0 | 2.8 | 6.2 |
Magnesium | 6.3 | 1.8 | 1.3 | <0.5 | 0.7 | 8.5 | 5.8 | <0.5 | 1.1 | <0.5 | <0.5 |
LC–MS-MS biomarker panel | |||||||||||
Uric acid | ✓ | ✓ | ✓ | ✓ | ✓ | ✓ | ✓ | ✓ | ✓ | ||
Caffeine | ✓ | ✓ | ✓ | ✓ | ✓ | ✓ | ✓ | ||||
Methylhistidine | ✓ | ✓ | ✓ | ✓ | |||||||
Theophylline | ✓ | ||||||||||
Normetanephrine | ✓ | ||||||||||
Urobilin | ✓ | ||||||||||
Theobromine | ✓ |
. | Ctrl . | S1 . | S2 . | S4 . | S5 . | S6 . | S7 . | S11 . | S12 . | S14 . | S15 . |
---|---|---|---|---|---|---|---|---|---|---|---|
Colorimetric assay | |||||||||||
Uric acid | 17.3 | 3.4 | 4.0 | <1 | 5.4 | 3.3 | 6.5 | <1 | 3.0 | 2.8 | 6.2 |
Magnesium | 6.3 | 1.8 | 1.3 | <0.5 | 0.7 | 8.5 | 5.8 | <0.5 | 1.1 | <0.5 | <0.5 |
LC–MS-MS biomarker panel | |||||||||||
Uric acid | ✓ | ✓ | ✓ | ✓ | ✓ | ✓ | ✓ | ✓ | ✓ | ||
Caffeine | ✓ | ✓ | ✓ | ✓ | ✓ | ✓ | ✓ | ||||
Methylhistidine | ✓ | ✓ | ✓ | ✓ | |||||||
Theophylline | ✓ | ||||||||||
Normetanephrine | ✓ | ||||||||||
Urobilin | ✓ | ||||||||||
Theobromine | ✓ |
Biomarkers check-marked if present at expected concentrations.
. | Ctrl . | S1 . | S2 . | S4 . | S5 . | S6 . | S7 . | S11 . | S12 . | S14 . | S15 . |
---|---|---|---|---|---|---|---|---|---|---|---|
Colorimetric assay | |||||||||||
Uric acid | 17.3 | 3.4 | 4.0 | <1 | 5.4 | 3.3 | 6.5 | <1 | 3.0 | 2.8 | 6.2 |
Magnesium | 6.3 | 1.8 | 1.3 | <0.5 | 0.7 | 8.5 | 5.8 | <0.5 | 1.1 | <0.5 | <0.5 |
LC–MS-MS biomarker panel | |||||||||||
Uric acid | ✓ | ✓ | ✓ | ✓ | ✓ | ✓ | ✓ | ✓ | ✓ | ||
Caffeine | ✓ | ✓ | ✓ | ✓ | ✓ | ✓ | ✓ | ||||
Methylhistidine | ✓ | ✓ | ✓ | ✓ | |||||||
Theophylline | ✓ | ||||||||||
Normetanephrine | ✓ | ||||||||||
Urobilin | ✓ | ||||||||||
Theobromine | ✓ |
. | Ctrl . | S1 . | S2 . | S4 . | S5 . | S6 . | S7 . | S11 . | S12 . | S14 . | S15 . |
---|---|---|---|---|---|---|---|---|---|---|---|
Colorimetric assay | |||||||||||
Uric acid | 17.3 | 3.4 | 4.0 | <1 | 5.4 | 3.3 | 6.5 | <1 | 3.0 | 2.8 | 6.2 |
Magnesium | 6.3 | 1.8 | 1.3 | <0.5 | 0.7 | 8.5 | 5.8 | <0.5 | 1.1 | <0.5 | <0.5 |
LC–MS-MS biomarker panel | |||||||||||
Uric acid | ✓ | ✓ | ✓ | ✓ | ✓ | ✓ | ✓ | ✓ | ✓ | ||
Caffeine | ✓ | ✓ | ✓ | ✓ | ✓ | ✓ | ✓ | ||||
Methylhistidine | ✓ | ✓ | ✓ | ✓ | |||||||
Theophylline | ✓ | ||||||||||
Normetanephrine | ✓ | ||||||||||
Urobilin | ✓ | ||||||||||
Theobromine | ✓ |
Biomarkers check-marked if present at expected concentrations.
Discussion
Three adulteration products were identified and tested. They did not appear effective in subverting drug testing as the effects on drug screening and confirmation were limited. In addition, the adulterated samples were readily identifiable. The two products that to some extent reduced drug concentrations contained either nitrite (A03) or iodate (A02), and both can be detected by either a general oxidation screen and/or interference in CEDIA immunoassays. It is unclear whether adulterant A01 contained an active ingredient at all, as the results of both drug screening and SVT assays were very similar to the unadulterated control urine.
Lower recovery of ∆9-THC-COOH and the corresponding internal standard after adulteration with pure potassium nitrate were reported by ElSohly et al. (13). The limited loss of ∆9-THC-COOH seen in this study with A03 might be connected to the pH (6.6) of the specimen, as increased losses have been observed at lower pH (14).
In samples adulterated with iodate (A02), reduced concentrations of both opioids and ∆9-THC-COOH were observed. While the exact mechanism is not known, most affected analytes have free hydroxyl functional groups. For the opioids, free hydroxyl groups in the 3rd or 14th position appear to be most important. The largest reduction was seen for oxymorphone, which has hydroxyl groups in both positions. In addition, analytes with a free hydroxyl group in either position (6-acetylmorphine, hydromorphone, morphine and oxycodone) all showed reductions in concentration when adulterated by iodate. Of interest, hydroxyl groups in the 6th position (codeine and morphine) did not seem to be affected by iodate. While ∆9-THC-COOH also has a free hydroxyl group, it is difficult to assess the importance for adulteration given the drastically different structure.
In contrast, the synthetic urines proved difficult to detect using standard SVT assays, which stresses the importance of detecting the use of these products at the collection site, and the need for future developments of SVT methodology. Urine is a complex matrix, and the results of the biomarker panel (Table IV) clearly show that there are many markers that can be used to distinguish synthetic and authentic urine samples. While the biomarker panel would work well as a confirmation assay, it is too expensive to be used as a screening assay. Two potential screening assays for synthetic urines were evaluated in this study: the Synthetic UrineCheck dipstick and a laboratory-based assay for uric acid.
While careful color analysis showed a difference between synthetic and authentic urines, we found the Synthetic UrineCheck dipsticks difficult to interpret, generating unclear color changes (Figure 2). Our results were different from a similar experiment carried out by Kim et al. (10), who reported good performance from the dipsticks. Potential explanations include either that the composition of synthetic urines has changed over the last few years or that analyst training can enable accurate reading of the dipsticks. Further investigations of the reagent are warranted to determine variability in authentic urine specimens and the ability of automated analyzers to identify synthetic urines.
The uric acid testing revealed that 8 out of 10 synthetic urine products contain uric acid (Table IV), illustrating the speed with which the manufacturers adapt to new testing protocols. To overcome this hurdle, future SVT biomarkers could be chosen to ensure that adding them to synthetic urine is either expensive and/or difficult. Of interest, novel immunoassay reagents targeting urinary tract proteins have been introduced (15), which may pose a bigger challenge to synthetic urine manufacturers than uric acid. To further reduce the impact from synthetic urines and adulterants on workplace drug testing programs, oral fluid was recently introduced as an additional matrix in regulated testing (16). Oral fluid collections are directly observed, making both substitution and adulteration more difficult for the donor.
Conclusions
A market search revealed 3 adulterants and 32 synthetic urine products. Testing of the adulterants identified products containing iodate or nitrite, both of which are readily detectable through SVT assays and/or CEDIA immunoassay interference. Negative (THC-COOH) and invalid (6AM, MAMP) immunoassay results were observed when the iodate containing adulterant was present. It also reduced the concentrations of opioids (6–27%) and THC-COOH (65%). The adulterant containing nitrite did not affect immunoassay results and only reduced the concentration of THC-COOH (22%) in confirmatory testing.
The synthetic urines were difficult to identify using traditional SVT assays, and alternative SVT methods revealed the presence of uric acid, caffeine and magnesium in most synthetic urines. Using a biomarker assay measuring both endogenous and exogenous analytes, synthetic urine could be clearly identified. The assay also provides several potential targets for future screening assays.
Supplementary Data
Supplementary Data is available at Journal of Analytical Toxicology online.
Acknowledgments
The authors acknowledge the support of the participating laboratories.
Funding
This work was funded by Division of Workplace Programs, Center for Substance Abuse Prevention, Substance Abuse and Mental Health Services Administration (SAMHSA) through the National Laboratory Certification Program contract (HHSS277201800001C).
Data Availability
The data underlying this article are available in the article and in its online supplementary material. The identity of the tested products will be disclosed upon a reasonable request to the corresponding author.
References
Department of Health and Human Services, Substance Abuse and Mental Health Services Administration. (
Department of Health and Human Services, Substance Abuse and Mental Health Services Administration. (