-
PDF
- Split View
-
Views
-
Cite
Cite
Natalia A Platosz, Tina M Binz, Markus R Baumgartner, Elena Lendoiro, Ana de Castro, Marta Concheiro, Quantification of Classic, Prescription and Synthetic Opioids in Hair by LC–MS-MS, Journal of Analytical Toxicology, Volume 45, Issue 9, November 2021, Pages 943–949, https://doi.org/10.1093/jat/bkaa152
- Share Icon Share
Abstract
The current use and misuse of synthetic and prescription opioids in the USA has reached epidemic status. According to the US Department of Health and Human Services, every day more than 130 people in the USA die after overdosing on opioids, and 2.1 million had an opioid use disorder in 2018. Hair is becoming an alternative matrix of increasing interest in forensic toxicology to investigate drug use and abuse patterns due to its long window of detection. The focus of this project was to develop and validate a method that simultaneously detects and quantifies 27 classic, prescription and synthetic opioids in hair by liquid chromatography–tandem mass spectrometry (LC–MS-MS). Hair samples were decontaminated and pulverized in a bead mill. Twenty-five milligrams of hair powder were incubated in a buffer overnight. Mixed mode cation exchange solid phase extraction was carried out before undergoing reversed-phase chromatographic separation, successfully resolving isobaric opioids. We used two multiple reaction monitoring transitions in positive mode to identify each analyte. The linearity range was 1–500 pg/mg for fentanyl and synthetic opioids and 10–500 pg/mg for prescription and classic opioids. Imprecision was <17.5% and bias ranged from −13.6 to 12.0%. Majority of compounds showed extraction efficiency >50%, and ion suppression from −89.2 to −26.6% (CV < 19%, n = 10). This method was applied to 64 authentic cases, identifying 13 compounds from our panel. A sensitive and specific method was developed for the identification and quantification of 27 classic, prescription and synthetic opioids in hair by LC–MS-MS.
Introduction
Opioids are analgesic drugs that act on the opioid receptor (mu-receptor) reducing the intensity of pain, and they show a high addiction potential. These substances also induce a dose-dependent respiratory depression (1), which can be life-threatening. Opioid-related overdoses have exponentially increased in the USA since 2000 (2). In 2018, the Department of Health and Human Services reported that 47,600 people died from overdosing on opioids (synthetic, prescription and/or heroin), and 32,656 deaths were attributed to overdosing on synthetic opioids other than methadone; thus, over 130 people died every day from opioid-related drug overdoses (3).
Since 2013, synthetic opioid-related overdoses have increased quicker than any other documented drug class. The synthetic opioid group refers to fentanyl, its analogs (e.g., acetyl fentanyl, furanyl fentanyl) and the new generation synthetic opioids (e.g., U-47700, MT-45). Among them, the leading cause of synthetic opioid-related deaths was due to misuse of fentanyl. Fentanyl was first introduced in 1960 and was approved as an intravenous anesthetic in 1972 (4). Fentanyl can be prescribed as transdermal patches, lollipops, sublingual tablets, as well as other forms (5, 6). The rise of illicit use and abuse began in the mid-2000 when the drug was identified to be sold alone as a powder or pressed into tablets, and mixed with heroin and cocaine. Quickly after, many analogs of fentanyl were created to circumvent current regulations and evade analytical detection (4). Since fentanyl and their analogs are known for becoming quickly addictive, they are usually placed as a Schedule I or II drug. Schedule II drugs are known for having a medical use, while Schedule I drugs do not. Drugs such as fentanyl, alfentanil, sufentanil and carfentanil have been used in the medical field as opioid analgesics and anesthetics to assist with pain management during and after surgeries. On the other hand, analogs of fentanyl such as acetyl fentanyl and furanyl fentanyl were created in clandestine laboratories to avoid control and are not seen as medical aid, and therefore have been placed into a Schedule I category. According the Drug Enforcement Administration, the most commonly reported synthetic opioids in 2017 was fentanyl (73% cases), followed by carfentanil and furanyl fentanyl (7% each) (7).
From a forensic standpoint, it is important to be able to identify these drugs in different biological matrices. Hair is a unique matrix in toxicology, which allows the detection of past drug exposure and the investigation of the chronological profile of that exposure. Hair is defined as a fully keratinized matrix that is composed of proteins, water, lipids and minerals. An advantage of hair over other matrices is that its collection is non-invasive and easily supervised, and has a larger window of detection (8). It is also easily transported and stored at room temperature and protected from light before analysis. Typically, hair is collected from the back of the head, the ‘vertex posterior’, where the growth rate is less influenced by sex and age. Head hair grows at an average of 1 cm per month, allowing for segmental analysis (9). Segmental analysis gives insight into past drug history on a month-to-month basis if 1 cm segments are used, which cannot be done with other matrices without multiple sampling and testing.
Although there is an increased necessity in monitoring and documenting different types of opioid exposure in clinical and forensic settings, limited methods have been developed for the determination of these compounds in hair (10–15). These methodologies focused on the determination of one type of opioids in hair (classic, prescription or synthetic opioids), and only Salomone et al. (12) included synthetic opioids and a limited number of prescription opioids. Considering that synthetic opioids are likely taken in combination with another opioid (16, 17), it is important to develop analytical tools that simultaneously detect different types of opioids. The method of multiple-opioids analysis saves time, costs and resources.
In this study, we developed a new analytical method by liquid chromatography–tandem mass spectrometry (LC–MS-MS) for the simultaneous determination and quantification of 27 classic, prescription and synthetic opioids in hair. The method was validated following guidelines published by the Scientific Working Group in Forensic Toxicology (18) and has been applied to 64 authentic samples.
Materials and Methods
Reagents and supplies
The following reference materials were purchased from Cerilliant (Round Rock, TX) at concentration between 0.05 and 1 mg/mL, (±)-cis-3-methylfentanyl, 4-ANPP (N-phenyl-1-(2-phenylethyl)-4-piperidinamine), 6-acetylmorphine, acetyl fentanyl, acrylfentanyl, alfentanil, butyryl fentanyl, carfentanil, cis-tramadol, codeine, EDPP (2-Ethylidene-1,5-dimethyl-3,3-diphenylpyrrolidine), fentanyl, furanyl fentanyl, hydrocodone, hydromorphone, methadone, morphine, norfentanyl, oxycodone, oxymorphone, para-fluorobutyryl fentanyl (PFBF), sufentanil and U-47700; and the internal standards 6-acetylmorphine-d3, codeine-d3, EDDP-d3, fentanyl-d5, hydrocodone-d6, hydromorphone-d3, methadone-d3, morphine-d3, norfentanyl-d5, oxycodone-d3, oxymorphone-d3, sufentanil-d5 and tramadol-13C-d3. The following reference materials were purchased from Cayman Chemical (Ann Arbor, MI) in powder form (5–10 mg), 4-methoxy-butyryl fentanyl, isobutyryl fentanyl, MT-45 (1-cyclohexyl-4-(1,2-diphenylethyl)-piperazine), isobutyryl fentanyl-d5 and valeryl fentanyl-d5, except valeryl fentanyl in methanol solution (1 mg/mL).
Methanol, acetonitrile, formic acid, isopropanol, dichloro-methane and ammonium hydroxide were purchased from Fisher Scientific (Fair Lawn, NJ). Two milliliter tubes with 2.4 mm metal beads purchased from Fisher Scientific were used to pulverize hair into a fine powder with a Bead Ruptor Elite Bead Mill Homogenizer (Omni International, Kennesaw, GA). Strata X-C 33 μm polymeric strong cation solid phase extraction (SPE) cartridges were purchased from Phenomenex (Torrence, CA). The SPE cartridges were assembled on a 24-port vacuum manifold from Fisher Scientific. Sarstedt Inc. 16 × 100 mm 10-mL screw cap tubes, FisherbrandTM 50 mL Easy ReaderTM plastic tubes and 350 μL fused insert vials were from Fisher Scientific.
Hair specimens
Drug-free (negative) hair samples were obtained from healthy volunteers and tested for the absence of drugs. Drug-free hair was used for the preparation of calibrators and quality control (QC) samples. De-identified authentic hair samples (n = 64) were collected at the Institute of Forensic Medicine in Zurich, Switzerland, and at the Institute of Forensic Sciences of the University of Santiago de Compostela, Spain. Most of these samples were from driving license granting cases. The samples were stored at room temperature in their original packing (aluminum foil or envelope) until analysis.
Preparation of stock solutions, calibrators, QC and internal standards
Working solutions containing all 27 analytes for calibrators and QCs were prepared at concentrations of 0.1, 0.01 and 0.001 μg/mL in methanol. A working solution of 15 internal standards was prepared at 0.05 μg/mL in methanol. All working solutions were stored in amber vials at –20°C. Calibrators were prepared at concentrations of 1, 5, 10, 25, 50, 100, 150, 250, 400 and 500 pg/mg, and QC samples were prepared at 3, 30 and 300 pg/mg, adding the adequate volume of the corresponding working solution. The internal standard concentration was 50 pg/mg.
Sample decontamination
Each sample was first placed into a clearly labeled 50 mL plastic tube. Next, 10 mL of deionized water (dH20) was added to the sample, capped and shaken for 2 minutes. The dH20 was decanted, replaced with 10 mL of methanol, capped and shaken for an additional 2 minutes. Finally, the methanol was decanted, replaced with 10 mL of dichloromethane, capped and shaken for 2 minutes. Finally, the dichloromethane was decanted, and the samples were dried overnight at room temperature.
Sample pulverization
Once samples were dried, they were cut into small pieces using scissors. The scissors were cleaned with methanol and dried between samples to avoid cross contamination. Next, the samples were placed into individual 2 mL tubes containing metal beads. The tubes were placed in the Omni International Bead Ruptor Elite Bead Mill Homogenizer and were pulverized at the following settings, speed 6 m/s, 3 cycles, for 50 seconds, dwell time of 3 minutes. Each sample was placed into its individual 10 mL tube with a goal weight of 25 ± 1 mg.
Sample digestion
After each samples’ weight was documented, 1.9 mL of mobile phase A (MPA) (0.01% formic acid + 2.5 mM ammonium formate in ultra-high purity, UHP water), 0.1 mL methanol and 25 μL of internal standard mixture at 0.05 μg/mL were added to each calibration point, QC and authentic sample. After all solutions were added, tubes were capped and vortexed. Each tube was incubated overnight at 37°C in a heating block. Once the incubation period was completed, samples were cooled to room temperature and centrifuged at 5,000 rpm for 10 minutes to allow all powdered hair to collect at the bottom of the tube.
Solid phase extraction
In order to extract desired analytes out of the incubation solution, a Phenomenex Strata X-C Polymeric Strong Cation exchange 3 mL 60 mg cartridge was employed. First, the cartridge was conditioned using 2 mL of methanol, followed by 2 mL of deionized water. Next, the sample was loaded into the cartridge and washed with 2 mL of 1% formic acid in deionized water, followed by 2 mL of methanol. The cartridges were dried for 10 minutes under vacuum. For elution, a 3 mL solution of dichloromethane:isopropanol:ammonium hydroxide (78:20:2, v:v:v) was added to each column. Eluates were evaporated at 50°C using a Biotage Turbovap LV (Charlotte, NC) under nitrogen flow. Dried extracts were reconstituted with 200 μL of MPA:acetonitrile (95:5). The solutions were transferred to injection vials containing micro-inserts, and 20 µL was injected into the LC–MS-MS.
Instrumentation
Samples were analyzed on a LC–MS 8050 instrumentation from Shimadzu (Columbia, MD). The Nexera high-performance liquid chromatography (HPLC) system consisted of a binary LC-20AD XR pump, an online degassing unit (DGU-20A 3R), a cooled autosampler (SIL-20A XR) and a column oven (CTO-20AC). A Kinetex polar C18, 1.7 µm particle size, 2.1 × 100 mm, 100 Å pore size, chromatographic column from Phenomenex was employed for this analysis. MPA was composed of 0.01% formic acid + 2.5 mM ammonium formate in UHP water, and MPB was composed of acetonitrile, with a flow rate of 0.3 mL/min. The column oven operated at 50°C. The gradient developed for this analysis increased from 5 to 20% of MPB at 0 to 3 min, 20 to 28% MPB at 3 to 14 min, 28 to 95% MPB at 14 to 24 min, held at 95% MPB from 24 to 26 min, and then decreased from 95 to 5% for one full minute, followed by 5 min of re-equilibration time. The total run time was 32 minutes.
The triple-quadrupole mass spectrometer operated with an electrospray ionization source in positive mode. The following parameters were employed for the source: nebulizing gas flow at 2 L/min, heating gas flow at 10 L/min, drying gas flow at 10 L/min, interface temperature at 300°C, desolvation line temperature at 250°C and heating block temperature at 400°C. Each analyte was injected directly into the mass spectrometer (no column) and full scan and product ion scan experiments were performed to identify 2 transitions per analyte. Supplemental Table 1 shows the resulting multiple reaction monitoring (MRM) transitions and voltages for the drugs and internal standards. Two MRM transitions were selected per analyte (one quantifier and one qualifier) and one MRM transition per internal standard.
Method validation
Guidelines published by the Scientific Working Group for Forensic Toxicology (18) were used to appropriately validate the method. We evaluated the following parameters: linearity, limit of detection (LOD), limit of quantification (LOQ), bias, precision, matrix effect, extraction efficiency, process efficiency, carryover, interferences and autosampler stability.
The linearity for each analyte was investigated analyzing 1 curve per day for 6 days (n = 6), using 10 non-zero calibrators between 1 and 500 pg/mg. The calibration model was evaluated using a least-squares residual linear and quadratic model, forced or non-forced through origin, and different weighting factors (non-weighted, 1/x and 1/x2), to produce the simplest and most appropriate model per compound. Residuals comparison by Student t-test were employed to evaluate the significance of linear vs. quadratic, forced vs. non-forced, and weighting factors (non-weighted vs. 1/x, 1/x vs. 1/x2). All statistical tests were significant if P-value < 0.05.
The same concentration was chosen for LOD and LOQ, and was equal to the lowest non-zero calibrator. LOQ was examined in triplicate over 3 different days using a different donor source for each day (n = 9). The bias of the LOQ samples were required to be within ±20% of the established concentration and imprecision <20% in order to pass.
Imprecision and bias data were collected in triplicate each day over a 5-day span (n = 15) using QC samples at low QC (3 or 30 pg/mg, depending on the analyte) and at high QC (300 pg/mg). Bias was determined by measuring the percent error of QC samples (n = 15) and was determined to be acceptable if results were within ±20% of the target concentration. Imprecision was assessed by measuring the coefficient of variation (CV) between the samples and was considered acceptable if below 20%. Intra-day imprecision was calculated for each concentration separately for each of the five runs. The largest calculated intra-day CV for each concentration was used to assess intra-day imprecision acceptability. Inter-day imprecision was calculated for each concentration over the five runs, using the combined data from all replicates of each concentration.
Extraction efficiency, matrix effect and process efficiency were determined at low and high QC. For each of these concentrations, five ‘before’ samples from different donors followed the original protocol where hair, internal standard, appropriate amount of stock solution and digestion liquid were added to each tube, incubated and extracted as mentioned in the section ‘Solid phase extraction’. Ten ‘after’ samples were prepared from different donors and only contained hair and digestion liquid. Working solutions and internal standards were added directly to the eluates prior to evaporation. In addition to this, neat samples were also made in triplicate by adding internal standard and the desired amount of the working solution, evaporated and reconstituted in mobile phase. To determine extraction efficiency, results between the ‘before’ and ‘after’ samples were evaluated, (MeanBefore/MeanAfter) × 100. For matrix effect, results between the neat and ‘after’ samples were evaluated, (MeanAfter − MeanNeat/MeanNeat) × 100. Process efficiency was evaluated by comparing results between the neats and ‘before’ samples, (MeanBefore/MeanNeat) × 100. Matrix effect results were deemed acceptable if ion suppression/enhancement were within ±25%, and the CV among the different sources did not exceed 15%. Scientific Working Group for Forensic Toxicology guidelines (18) do not recommend any limits for extraction and process efficiencies.
To evaluate carryover, an injection at the upper limit of quantification (ULOQ, 500 pg/mg), was directly followed by the injection of a blank matrix sample (n = 5). Results were deemed acceptable if the blank sample had signals below the LOQ for each specific analyte.
Blank hair samples from 10 different sources (without the addition of internal standard) were evaluated for the absence of interferences produced from the matrix. Results were deemed acceptable if the sample had signals below the LOQ for each specific analyte. Exogenous interferences were evaluated by injecting solution containing amphetamine, methamphetamine, 3,4-methylenedioxyamphetamine (MDA), 3,4-methylenedioxymethamphetamine (MDMA), cocaine and benzoylecogonine at 500 pg/mg. Results were deemed acceptable if the sample had signals below the LOQ for each specific analyte. Autosampler stability was evaluated by reinjecting low and high QCs after being in the autosampler for 24 h at 10°C. The samples were considered stable if they quantified within ±20% of their original concentration.
To confirm the presence of an analyte within a sample, the retention time had to be within 2% of the average retention time of the calibrators, the 2 MRM transitions had to be detected and show ion ratio qualifier transition/quantifier transition within 20% of average ion ratio of the calibrators (19).
Results and Discussion
Chromatographic separation
The LC–MS-MS method developed for the detection of classic, prescription and synthetic opioids in hair produced a good chromatographic separation for the 27 target analytes at a working linear range of 1–500 or 10–500 pg/mg, depending on the compound. A representative total ion chromatogram for each analyte of interest at 30 pg/mg in hair is shown in Figure 1. This method was capable of eluting all compounds between 1.5 (morphine) and 18 minutes (MT-45), and successfully resolved isobaric compounds, such as morphine and hydromorphone, codeine and hydrocodone, and the synthetic opioids 3-methylfentanyl, isobutyryl fentanyl and butyryl fentanyl (Figure 2).
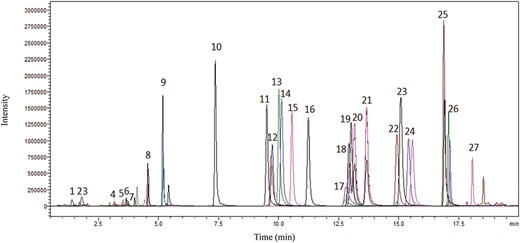
Total ion chromatogram (TIC) of all multiple reaction monitoring (MRM) transitions for analytes included in the study at 30 pg/mg in hair. 1, Morphine; 2, Oxymorphone; 3, Hydromorphone; 4, Codeine; 5, Oxycodone; 6, 6-AM; 7, Hydrocodone; 8, Norfentanyl; 9, Tramadol; 10, Acetyl Fentanyl; 11, Acryl Fentanyl; 12, U-4770; 13, 4-ANPP; 14, Fentanyl; 15, Alfentanyl; 16, Furanyl Fentanyl; 17, EDDP; 18, 3-Methylfentanyl; 19, Carfentanil; 20, Isobutyryl fentanyl; 21, Butyryl fentanyl; 22, PFBF; 23, 4-Methoxybutyrylfentanyl; 24, Sufentanil; 25, Methadone; 26, Valeryl Fentanyl; 27, MT-45.
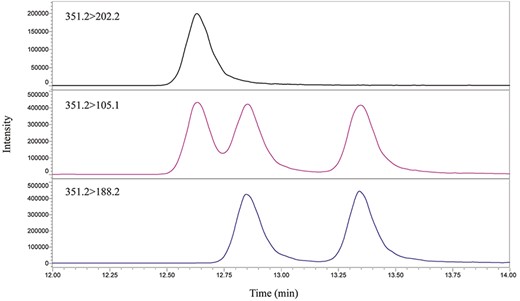
MRM chromatograms of the quantifier and qualifier transitions of three isobaric compounds, in elution order, 3-methylfentanyl (351.2 > 202.2, 351.2 > 105.1), isobutyryl fentanyl (351.2 > 188.2, 351.2 > 105.2) and butyryl fentanyl (351.2 > 188.2, 351.2 > 105.2), at 30 pg/mg in hair.
Method validation
The linearity for this method was 1–500 or 10–500 pg/mg depending on the target analyte. Method calibration was accomplished via a linear, non-forced and 1/x2 model for all analytes, except alfentanil, oxycodone and PFBF, which followed a linear, non-forced, 1/x model. All calibrators quantified within ±15% of target, except LOQ within ±20% (n = 6). The LOQ was either 1 or 10 pg/mg depending on the analyte (Supplemental Table 2). Bias results at the LOQ were within ±20%, and imprecision was <20%, except 4-ANPP intra-day imprecision (20.9%), and MT-45 (24.4%) and oxycodone (22.0%) inter-day imprecision. These values were slightly above the recommended limit of 20%, but all of them below 25%. Imprecision up to 25% should be considered for these compounds when analyzing authentic cases at this level of concentration. Bias and imprecision results at the LOQ are summarized in Supplemental Table 3.
The bias and imprecision of 3 replicates over a 5-day period (n = 15) for each compound was monitored at 3 and 300 pg/mg QC for analytes that have a 1–500 pg/mg linearity, and at a 30 and 300 pg/mg QC for analytes that have a linearity of 10–500 pg/mg. Bias and imprecision at the 2 QCs demonstrated proficiency and were all within ±20% of the target concentration and <20%, respectively. Imprecision results were 3.9–17.5%, and bias results ranged from –13.6 to 12.0% (Supplemental Table 4).
All extraction efficiency, process efficiency and matrix effect data were calculated at 3 or 30 pg/mg (depending on the analyte), and 300 pg/mg QC using blank hair samples spiked with analytes and internal standards before or after extraction or as neats as previously described (Section ‘Method validation’ in ‘Materials and Methods’). For extraction efficiency at 3 or 30 pg/mg, it was identified that 16 opioids were above 80%, 10 opioids were between 50 and 80% and 1 opioid was below 50%. For 300 pg/mg, 3 opioids were above 80%, 19 opioids were between 50 and 80% and 5 opioids were below 50%. For process efficiency at 3 or 30 pg/mg, 7 opioids were above 50% and 20 opioids were below 50%. At 300 pg/mg QC, 14 analytes were above 50% and 13 opioids were under 50%. Overall, the majority of analytes had an extraction efficiency and process efficiency above 50%. All analytes showed ion suppression at their specified low QC varying between −89.2 and −28.3%, with a CV (n = 10) between 2.4 and 19%. At the high QC, 11 out of 27 analytes exhibited ion suppression varying between −72.4 and −26.6%, with a CV between 6.9 and 16.4%. Although there was ion suppression observed outside of the accepted 20%, the CV indicated that it was uniform throughout all samples. Also, deuterated analogs were employed as internal standards to compensate for these effects. A summary of these results is shown in Supplemental Table 5.
The carryover was evaluated by injecting a blank sample after the ULOQ at 500 pg/mg over a 5-day period. None of the blank samples calculated above the LOQ at 1 or 10 pg/mg; therefore, there was no evidence of carryover. To investigate the potential presence of endogenous interferences, blank hair samples from healthy donors (n = 10) were used. All 10 blank hair sources provided negative results for the entire panel of analytes in our method and therefore were evaluated to be free of any endogenous interferences. The exogenous interferences that were explored in this study were common drugs of abuse (amphetamine, methamphetamine, MDA, MDMA, cocaine and benzoylecogonine) injected at a high concentration (500 pg/mg) into the LC–MS-MS There was no indication of any exogenous interferences. Autosampler stability was evaluated by reinjecting 3, 30 and 300 pg/mg QC samples after 24 h in the autosampler at 10°C. All analytes at the different QCs showed good stability (±20% of target concentration) after 24 h in the autosampler.
Analysis of authentic samples
Authentic samples (n = 64) were donated by the Institute of Legal Medicine in Zurich, Switzerland, and the Institute of Forensic Sciences in Santiago de Compostela, Spain. Table I summarizes the results of 3–5 cm hair samples. The most detected substance from all tested samples was morphine. It occurred in 72% of the samples, followed by codeine (65.6%) and 6-acetylmorphine (56.3%). Methadone was present in 54.7% of cases, hydromorphone in 50%, hydrocodone in 31.3%, oxycodone in 20.3% and oxymorphone in 18.8%. The classic and prescription opioids concentrations ranged from 8 to >500 pg/mg. Synthetic opioids were detected in just 5 cases (4 cases fentanyl and 1 case furanyl fentanyl), with concentrations from 1 to 326.3 pg/mg. It has to be kept in mind that all the authentic samples were collected in European countries, Switzerland and Spain, where the presence of synthetic opioids is lower than in the USA (20). Salomone et al. (17) recently published synthetic opioid concentrations in hair samples collected in the USA between November 2016 and August 2018. The most common synthetic opioid detected was fentanyl (67.6% cases), alone or together with other derivatives, acetyl fentanyl (36.8% cases) or furanyl fentanyl (29.7% cases).
Number of Positive Cases and Range of Concentrations for Each Target Analyte Detected in Authentic Cases (N = 64)
Analyte . | # Positive cases . | Range of concentrations (pg/mg) . |
---|---|---|
Morphine | 47 | 8 – >500 |
6-AM | 36 | 13.8 – >500 |
Codeine | 43 | 8.1 – >500 |
Hydromorphone | 34 | 8 – >500 |
Hydrocodone | 20 | 8.1 – >500 |
Oxycodone | 13 | 12.3 – >500 |
Oxymorphone | 12 | 11.8 – 507 |
Tramadol | 31 | 8.4 – >500 |
Methadone | 37 | 9.8 – >500 |
EDDP | 30 | 8.1 – >500 |
Fentanyl | 4 | 1.7–326.3 |
Norfentanyl | 1 | 20.3 |
Furanyl fentanyl | 1 | 1 |
Analyte . | # Positive cases . | Range of concentrations (pg/mg) . |
---|---|---|
Morphine | 47 | 8 – >500 |
6-AM | 36 | 13.8 – >500 |
Codeine | 43 | 8.1 – >500 |
Hydromorphone | 34 | 8 – >500 |
Hydrocodone | 20 | 8.1 – >500 |
Oxycodone | 13 | 12.3 – >500 |
Oxymorphone | 12 | 11.8 – 507 |
Tramadol | 31 | 8.4 – >500 |
Methadone | 37 | 9.8 – >500 |
EDDP | 30 | 8.1 – >500 |
Fentanyl | 4 | 1.7–326.3 |
Norfentanyl | 1 | 20.3 |
Furanyl fentanyl | 1 | 1 |
Number of Positive Cases and Range of Concentrations for Each Target Analyte Detected in Authentic Cases (N = 64)
Analyte . | # Positive cases . | Range of concentrations (pg/mg) . |
---|---|---|
Morphine | 47 | 8 – >500 |
6-AM | 36 | 13.8 – >500 |
Codeine | 43 | 8.1 – >500 |
Hydromorphone | 34 | 8 – >500 |
Hydrocodone | 20 | 8.1 – >500 |
Oxycodone | 13 | 12.3 – >500 |
Oxymorphone | 12 | 11.8 – 507 |
Tramadol | 31 | 8.4 – >500 |
Methadone | 37 | 9.8 – >500 |
EDDP | 30 | 8.1 – >500 |
Fentanyl | 4 | 1.7–326.3 |
Norfentanyl | 1 | 20.3 |
Furanyl fentanyl | 1 | 1 |
Analyte . | # Positive cases . | Range of concentrations (pg/mg) . |
---|---|---|
Morphine | 47 | 8 – >500 |
6-AM | 36 | 13.8 – >500 |
Codeine | 43 | 8.1 – >500 |
Hydromorphone | 34 | 8 – >500 |
Hydrocodone | 20 | 8.1 – >500 |
Oxycodone | 13 | 12.3 – >500 |
Oxymorphone | 12 | 11.8 – 507 |
Tramadol | 31 | 8.4 – >500 |
Methadone | 37 | 9.8 – >500 |
EDDP | 30 | 8.1 – >500 |
Fentanyl | 4 | 1.7–326.3 |
Norfentanyl | 1 | 20.3 |
Furanyl fentanyl | 1 | 1 |
Among the cases positive for the prescription opioids, 6 cases were positive for both oxycodone and oxymorphone with an oxymorphone/oxycodone ratio from 0.04 to 1.01, and 14 cases for both hydromorphone and hydrocodone, with hydromorphone/hydrocodone ratios ranging from 0.04 to 7.7. If present as metabolites, oxymorphone and hydromorphone hair concentrations should be less than 10% of the parent drug (oxycodone and hydrocodone, respectively) concentrations (21, 22). If hydromorphone and oxymorphone are present at higher concentrations, this is an indication that both opioids (oxymorphone and oxycodone, hydromorphone and hydrocodone) were consumed. Bearing this in mind, among the authentic cases analyzed, most of them indicated a concomitant use of both opioids, 4 cases out of 6 oxymorphone and oxycodone, and 12 cases out of 14 hydrocodone and hydromorphone use. Regarding other metabolites included in this method, EDDP presented concentrations of about 10% of methadone concentrations, in accordance with previous publications (23, 24). If methadone concentrations were <29 pg/mg, EDDP was not detected. Fentanyl was detected in four cases (1.7–326.3 pg/mg), but its metabolite norfentanyl was detected only in one sample (norfentanyl 20.3 pg/mg, fentanyl 326.3 pg/mg). If fentanyl concentrations were low (1.7–6.4 pg/mg), its metabolite norfentanyl was not detected. Salomone et al. (17) also reported that with low fentanyl hair concentrations (below 13 pg/mg), norfentanyl could not be detected.
The present method was capable of analyzing analytes that were both well above the ULOQ, as well as ones that were at the edge of the LOQ. For example, sample 34 (5 cm hair sample) contained 9 opioids from our panel; 6-acetylmorphine, morphine and codeine (Figure 3a–c) were well above the ULOQ and were indicators of a chronic heroin user. This sample also successfully quantified high concentration of hydromorphone, oxymorphone, hydrocodone, EDDP and methadone (Figure 3d–h). Interestingly, furanyl fentanyl was also present at 1 pg/mg, just at our LOQ (Figure 3i), suggesting exposure to this substance by synthetic opioid-laced heroin.
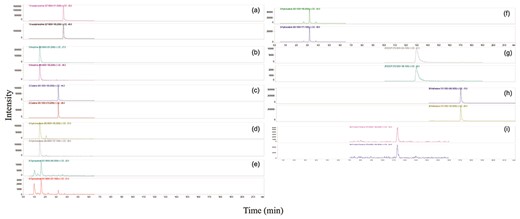
Quantifier and qualifier MRM transitions of sample 34 positive for (a) 6-acetylmorphine at >500 pg/mg, (b) morphine at >500 pg/mg, (c) codeine at >500 pg/mg, (d) hydromorphone at 514.5 pg/mg, (e) oxymorphone at 109 pg/mg, (f) hydrocodone at 67.1 pg/mg, (g) EDDP at 87.6 pg/mg, (h) methadone at 690.9 pg/mg and (i) furanyl fentanyl at 1 pg/mg.
Comparison to previously published methods
There have been several methods previously published on the identification and quantification of prescription, classic and/or synthetic opioids in hair (10–15). These methods were focused on one type of opioids (classic or prescription or synthetic), or slightly incorporated opioids from a different category (12). Considering different types of opioids (heroin, fentanyl analogs, prescription opioids) are likely taken in combination (16, 17), it is important to develop analytical tools that simultaneously detect these types of opioids. The present method is the first one that simultaneously detects 10 classic and prescription opioids and 17 synthetic opioids in hair samples with high specificity (2 MRM transitions per analytes) and sensitivity (LOQ 1–10 pg/mg). Salomone et al. (12) included tramadol, oxycodone, hydrocodone and 10 synthetic opioids. They monitored two MRM transitions for all compounds, except for tramadol, which only produced one transition. We were able to monitor two transitions to identify this compound, fulfilling the confirmation criteria for all the analytes in the method.
The chromatographic separations of the methodologies focused on synthetic opioids (10–12) were faster (6–10 min total run time) than the one in the current method (32 min total run time). The main reason for this observation was that in our method we were able to chromatographically resolve isobaric synthetic opioids (3-methyl fentanyl, isobutyryl fentanyl and butyryl fentanyl), which were not included in the analysis (10, 12) or dropped from the method (11) because they were not resolved.
The previously published methods employed 10 to 50 mg of hair and achieved limits of quantification from 0.5 to 50 pg/mg (11–14). This sensitivity is similar to the one in the present method, 25 mg LOQ 1–10 pg/mg. Bursardò et al. (10) developed the most sensitive method with LOQ from 0.011 to 0.021 pg/mg; however, only fentanyl and analogs were included in the analysis.
Previous studies digested the hair samples in methanol, making necessary an evaporation step before proceeding with the extraction procedure (11, 13, 15). In the present method, the samples were incubated in a buffer, which allowed proceeding directly to the loading step in the SPE. Cation exchange SPE was employed by Ramírez et al. (11), similarly than in the present procedure, and reversed phase SPE by Busardò et al. (10). Rosado et al. (13) employed microextraction by packed sorbent, and Salomone et al. (12) and Kim et al. (14) injected the methanol extract without clean-up procedure.
Conclusions
A sensitive and specific method was developed for simultaneous identification and quantification of 27 classic, prescription and synthetic opioids in hair by LC–MS-MS. A lower LOQ of 1 pg/mg for synthetic opioids and 10 pg/mg for classic and prescription opioids in 25 mg of hair were achieved. The method was applied to 64 authentic hair samples. The most detected substance from all tested samples was morphine (73% samples), followed by codeine (67%). Synthetic opioids were detected in five cases; fentanyl in four and furanyl fentanyl in one case.
Supplementary data
Supplementary data is available at Journal of Analytical Toxicology online.