-
PDF
- Split View
-
Views
-
Cite
Cite
J. M. Neary, R. D. Brown, T. N. Holt, K. R. Stenmark, R. M. Enns, M. G. Thomas, F. B. Garry, Static and dynamic components of right ventricular afterload are negatively associated with calf survival at high altitude, Journal of Animal Science, Volume 94, Issue 10, October 2016, Pages 4172–4178, https://doi.org/10.2527/jas.2016-0652
- Share Icon Share
Abstract
The purposes of this study were to evaluate mean, systolic, and diastolic pulmonary arterial pressures; pulmonary arterial pulse pressures; and systemic oxygen extraction fraction as risk factors for the survival of suckling calves on one ranch located at an altitude of ≥ 2,730 m in Colorado, USA. A prospective cohort study of 58 calves was performed. Pulmonary arterial pressures and systemic oxygen extraction were measured when calves were approximately 3 mo (86 ± 7 d) and 7 mo (197 ± 6 d) of age. Seven of the 58 calves (12%), 4 steers and 3 heifers, were unaccounted for and presumed dead between 3 and 7 mo of age. Calves presumed to have died between 3 and 7 mo of age had significantly greater mean (P = 0.005) and systolic (P = 0.001) pulmonary arterial pressures and greater pulse pressures (P = 0.03) at 3 mo of age than calves that survived to 7 mo. Calves presumed to have died tended to have greater systemic oxygen extraction fractions at 3 mo of age than calves that survived (P = 0.13). Diastolic pressure was not associated with survival (P = 0.27). Mean pulmonary arterial pressure is predominantly determined by static resistance attributable to distal pulmonary arterial remodeling. Pulse pressure and systolic pulmonary arterial pressure represents the dynamic or oscillatory resistance and is determined by the characteristics of ventricular ejection and proximal arterial stiffness. These findings indicate that it may be beneficial to include pressure measurements indicative of both static and dynamic pulmonary arterial resistance in the selection of breeding stock at high altitude.
INTRODUCTION
Right-sided congestive heart failure (RHF) secondary to pulmonary hypertension, or cor pulmonale, is a leading cause of suckling calf death loss among ranches at altitudes over 2,400 m (Neary et al., 2013). The susceptibility of cattle (Bos taurus) to hypoxia-induced pulmonary hypertension has been attributed to their highly muscular small pulmonary arteries that vasoconstrict and remodel in response to hypoxia (Alexander and Jensen, 1963a,b; Tucker et al., 1975).
Because mean pulmonary arterial pressure (mPAP) is moderately heritable (Shirley et al., 2008; Newman et al., 2015), producers at high altitudes (> 2000 m) commonly screen their breeding stock to identify individuals with high mPAP—typically ≥ 45 mmHg—and remove them from the herd (Holt and Callan, 2007). This strategy is thought to reduce the incidence of RHF because cattle with mPAP ≥ 45 mmHg are considered to be at increased risk of RHF (Holt and Callan, 2007). Although the prognostic value of PAP measurement has been validated in other species (Ghio et al., 2001), it has not been determined in cattle. Further, to our knowledge, there has been no scientific validation that the identification and removal of individuals with mPAP ≥ 45 mmHg from the breeding stock will reduce the incidence of RHF in the calf crop. Therefore, it is important to define the utility of mPAP to predict calf survival.
To date, selection strategies to reduce calf death loss from RHF have focused on mPAP, reflecting the static resistance to ventricular afterload attributable to distal pulmonary arterial remodeling (Alexander and Jensen, 1963a,b; Holt and Callan, 2007). By contrast, the dynamic, pulsatile component of pulmonary arterial blood flow has largely been ignored; however, more recent evidence from human studies suggests that disturbed flow pulsatility arising from pulmonary arterial stiffening may contribute to the development of RHF (Schäfer et al., 2016). If so, indirect measures of pulmonary arterial stiffening, such as systolic pulmonary arterial pressure (sPAP) and pulmonary arterial pulse pressure, may also serve as valuable indicators of RHF susceptibility in cattle.
It has also been suggested that the small cardio-pulmonary system of domestic cattle relative to body size and oxygen requirements is a risk factor for RHF (Veit and Farrell, 1978). A reduction in the rate of oxygen delivery relative to metabolic demand would manifest physiologically as an increased systemic oxygen extraction fraction (sOEF), that is, the proportion of oxygen within arterial blood that is extracted by the peripheral tissues (McLellan and Walsh, 2004). If the rate of oxygen delivery is reduced, sOEF must increase to maintain aerobic metabolism at steady state. Consequently, calves that have a high sOEF (> 0.30) may be at greater risk of decompensated heart failure and death because they have less physiological reserve to respond to the increased cardiac workload associated with increasing mPAP.
The purposes of this study were to evaluate mean, systolic, diastolic pulmonary arterial pressures, pulmonary arterial pulse pressures, and sOEF as risk factors for the survival of suckling calves to 7 mo of age on 1 ranch located at an altitude of ≥ 2,730 m in Colorado, USA.
MATERIALS AND METHODS
Study Site and Cattle
The 58 calves (30 heifers and 28 steers) included in the study were a composite of Red Angus, Hereford, Gelbvieh, and Simmental breeds. Calves were born to primiparous heifers on a commercial cow-calf ranch at an altitude of 2,410 m. At 2 mo of age, they were moved to new pastures at an altitude of 2,730m. From 4 to 7 mo of age, calves had access to pastures ranging in altitude from 2,730 m to approximately 3,500 m. Calves were sampled twice at approximately 3 mo (86 ± 7 d) and 7 mo (197 ± 6 d) of age. Every second calf into the chute was sampled. Fifty-one of the 58 calves sampled at 3 mo of age survived to 7 mo of age and were resampled.
The dams were given prebreeding and precalving vaccinations offering protection against viral agents of respiratory disease. Calves were vaccinated against the same respiratory pathogens at 4 to 8 wk of age and 2 to 4 wk before weaning. All calves were sired by bulls with a mPAP < 42 mmHg at 2,440 m. A hormonal growth promotant (Synovex C; Zoetis, Madison, NJ) containing 100 mg progesterone and 10 mg estradiol benzoate was administered to all calves in the herd at 2,730 m when approximately 8 wk old. All calves were clinically healthy when sampled at 3 mo of age. The Colorado State University Animal Care and Use Committee approved the study before any animals were sampled (protocol 09–1524A).
Pulmonary Arterial Pressure (PAP) Testing
Holt and Callan (2007) provide a full description of the equipment, materials, and facilities required for PAP testing. In brief, saline-filled, polyethylene catheter tubing (external and internal diameter of 17 and 12 mm, respectively) was fed through a 12-gauge, 8.9-cm needle that was inserted into the jugular vein. The position of the catheter tip within the vascular system was determined from the pressure waveform on the oscilloscope (BM5Vet; Bionet America, Inc., Tustin, CA). The jugular vein, right atrium, right ventricle, and pulmonary artery have distinct pressure waveforms (Holt and Callan, 2007). Once inside the pulmonary artery, mean, systolic, and diastolic pressures were recorded after the pressure waveforms stabilized. Pulse pressure was calculated as the absolute difference between systolic and diastolic pressures. Calves were moved to a small pen enclosure for at least 20 min before sampling.
Blood-Gas Analyses
Approximately, 2.5 mL of blood aliquots of arterial and mixed venous blood were collected in 3-mL syringes. Arterial blood was collected from the coccygeal artery using a 22-gauge, 2.54-cm (1”) hypodermic needle. The bovine coccygeal artery is a suitable source for blood-gas analysis (Collie, 1991; Nagy et al., 2002). Mixed venous blood was collected via the catheter used for pulmonary arterial pressure measurement. Syringes used for arterial blood gas collection were heparinized with approximately 0.25 mL of sodium heparin (1,000 IU/ml). The plunger of each syringe was pulled back to the 3-mL mark coating the inner chamber surface with heparin. Heparin was then expelled so that only the needle hub contained heparin. The sample was discarded if during collection the flow of arterial blood was interrupted. Air bubbles within the blood were immediately expelled and the first several drops of blood discarded before analysis.
Blood-gas tensions and hemoglobin concentrations were measured using a handheld analyzer (VetScan i-STAT 1; Abaxis, Union City, CA). Oxygen tensions and oxyhemoglobin saturations of arterial and mixed venous blood were corrected to standard conditions (pH 7.4; 37° C) using equations developed specifically for bovine hemoglobin (Gustin et al., 1988; Clerbaux et al., 1993).
Systemic Oxygen Extraction Fraction (sOEF)
The sOEF is the systemic oxygen consumption expressed as a fraction of systemic oxygen deliver. Systemic OEF was calculated using the following formula:
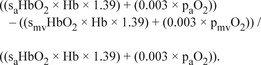
saHbO2 and smvHbO2 are arterial and mixed venous oxyhemoglobin saturation (%), respectively; Hb is hemoglobin concentration (g/L); and, paO2 and pmvO2 are arterial and mixed venous oxygen tensions (mm Hg).
Calf Mortality
A calf was considered dead if the dam was accounted for at the second test but the calf was not. The extensive and rugged mountainous terrain over which the calves were located requires travel by horseback; consequently, finding sick and deceased calves is extremely challenging and time consuming. Calf abandonment was unlikely to have occurred because mothering ability is a trait of high importance in the selection of range cattle. Grafted calves (n = 1) were excluded from the study because of the greater likelihood of calf abandonment.
Statistical Analyses
Descriptive statistics were used to summarize the data and graphically presented as scatterplots (STATA version 12; Stata Corporation, College Station, TX). Only 7 of the 58 calves in the cohort were presumed to have died; consequently, median values are provided as measures of central tendency. Further, Mann–Whitney U tests were used to determine if the pulmonary arterial pressures or sOEF of the 3 mo old calves differed according to 7 mo survival status. Pairwise correlation analyses were performed to determine if measurements obtained at 3 mo of age were predictive of measurements obtained at 7 mo of age.
RESULTS
Descriptive Summary
Seven of the 58 calves (12%), 4 steers and 3 heifers, were unaccounted for and presumed dead between 3 and 7 mo of age. Five of the 7 calves were included in the analysis. The catheter could not be advanced into the right atrium of 1 steer calf. This was most likely due to a high right atrial pressure. Another steer calf was excluded from the analysis because it had been grafted; consequently, calf abandonment was a likely confounding factor.
Evaluation of pairwise correlations between explanatory variables at 3 mo of age revealed that the following pairs of variables contained similar information: mPAP and sPAP (r = 0.82), mPAP and dPAP (r = 0.82), and sPAP and pulse pressure (r = 0.62). Moderate correlation was found between sPAP and dPAP (r = 0.50), mPAP and sOEF (r = 0.41), and dPAP and pulse pressure (r = −0.36). All other variable combinations were poorly correlated (r < |0.30|). The poor correlation between mPAP and pulse pressure is evident in Fig. 1A and 1D. For example, calf 5 had the second lowest mPAP (39 mm Hg) but the greatest pulse pressure (64 mm Hg). Consequently, although the right ventricle had a lower steady state resistance against which it had to work when compared to calves with greater mPAP, its external work was augmented to achieve highly pulsatile flow.
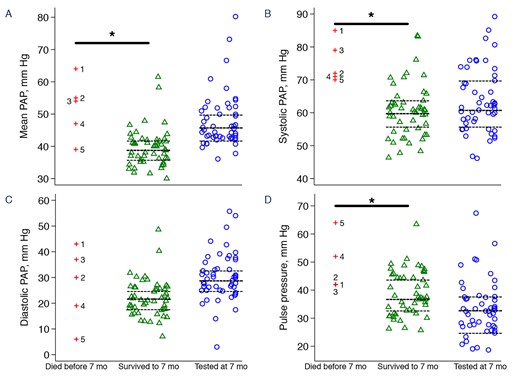
Scatter plot of (A) mean pulmonary arterial pressures; (B) systolic pulmonary arterial pressures; (C) diastolic pulmonary arterial pressures; and, (D) pulmonary arterial pulse pressures obtained from calves at 3 mo of age, according to survival status to 7 mo of age, and 7 mo of age. Measurements were taken at an altitude of 2,730 m. Calves presumed to have died before 7 mo of age are individually identified by a number. Median and quartiles are represented by dashed lines. * indicates P < 0.05 between calves presumed to have died and those that survived to 7 mo.
Mean and diastolic PAP at 3 mo of age were somewhat predictive of the values obtained at 7 mo of age. All other variables obtained at 3 mo of age were poorly correlated with values obtained at 7 mo. Pairwise correlations between 3 mo and 7 mo of age were statistically significant for mPAP (r = 0.57, P < 0.001), dPAP (r = 0.52, P < 0.001), and sPAP (r = 0.28, P = 0.005), but not for sOEF (r = 0.25, P = 0.25) and pulse pressure (r = −0.03, P = 0.80).
Risk Factors for Nonsurvival
Both static and dynamic components of right ventricular afterload were risk factors for death. Calves that died between 3 and 7 mo of age had significantly greater mean (P = 0.005) and systolic (P = 0.001) pulmonary arterial pressures and greater pulse pressures (P = 0.03) than calves that survived to 7 mo (Fig. 1). Median mPAP, sPAP, and pulse pressures for calves presumed to have died and for those that survived to 7 mo were 54 mm Hg and 39 mm Hg, 72 mm Hg and 60 mm Hg, and 43 mm Hg and 37 mm Hg, respectively. Calves that died tended to have greater systemic oxygen extraction fractions (P = 0.13) than calves that survived (Fig. 2). Median sOER values for calves presumed to have died and for those that survived to 7 mo were 34% mm Hg and 24%, respectively. Diastolic pulmonary arterial pressures were not associated with risk of death (P = 0.27).
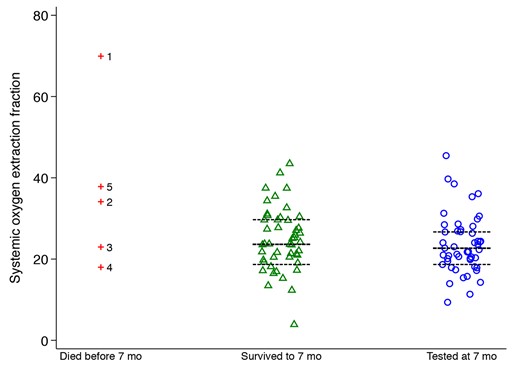
Scatter plot of systemic oxygen extraction fraction obtained from calves at 3 mo of age, according to survival status to 7 mo of age, and 7 mo of age. Measurements were taken at an altitude of 2,730 m. Calves presumed to have died before 7 mo of age are individually identified by a number. Median and quartiles are represented by dashed lines. Calves presumed dead by 7 mo of age tended to have greater systemic oxygen extraction fractions at 3 mo of age than calves that survived (P = 0.13).
DISCUSSION
The findings of this study indicate that both static and dynamic components of pulmonary arterial resistance were negatively associated with calf survival at high altitude. Calves presumed to have died prior to weaning at 7 mo of age had significantly greater mPAP, sPAP, and pulmonary arterial pulse pressures than calves that survived. Consequently, the genetic selection of breeding stock to reduce mPAP should reduce the risk of calf death loss given that mPAP has been estimated to be moderately heritable (Shirley et al., 2008; Newman et al., 2015). Traditionally, only mPAP, or steady state resistance, has been considered in the genetic selection of breeding stock at high altitude. Our findings indicate, however, that it may be beneficial to include pressure measurements reflective of dynamic resistance, alongside mPAP, in the selection of breeding stock at high altitude.
Determinants of pulse pressure and systolic arterial pressure include arterial compliance (∂V/∂P), or stiffening; stroke volume, the amount of blood ejected per beat from the right ventricle into the pulmonary artery; and, the ventricular ejection velocity of blood. Based on pulmonary pressures alone, we are not able to differentiate between these possible causes of elevated pulse pressure; however, the findings of other studies indicate that pulmonary arterial stiffening is a likely cause of the elevated pulse pressure. Human pulmonary hypertension (PH) patients and animal models of PH, such as hypoxic calves, rats, and mice, all show evidence of increased stiffness of the proximal pulmonary artery (Hunter et al., 2008; Stenmark et al., 2009; Hunter et al., 2011; Lammers et al., 2012; Stevens et al., 2012). Additionally, pulmonary arterial stiffness has been shown to have significant prognostic value in human pulmonary hypertension (Gan et al., 2007; Sanz et al., 2009; Hunter et al., 2011; Wang and Chesler, 2011; Stevens et al., 2012). Vessel stiffening not only increases ventricular workload, but the resultant increase in flow pulsatility induces inflammatory changes and cellular proliferation in the downstream pulmonary microcirculation (Li et al., 2009). These findings challenge the long-held belief that bovine pulmonary arterial hypertension is solely due to aberrant remodeling of the distal muscular pulmonary arterioles (Rhodes, 2005). To confirm that arterial stiffening is a cause of elevated pulse pressure in future studies, it will be necessary to rule out the other possible determinants by including measures of right ventricular output, flow velocity, and pulmonary arterial distension. These measurements were not feasible in our field studies, but will be accessible in a laboratory setting.
We should also consider whether increased static or dynamic pulmonary resistances could have predisposed calves to other causes of mortality such as respiratory disease. The relationships between BRD and PH are complex and likely reciprocal. The available evidence indicates that respiratory disease causes a three-fold increase in the risk of RHF in feedlot cattle (Neary et al., 2016). Conversely, human patients with subclinical and nonsymptomatic PH accompanied by pro-inflammatory vascular remodeling are at risk for exaggerated and life-threatening complications from respiratory infections (Pullamsetti et al., 2011). Individuals with preexisting pulmonary hypertension are likely to be more susceptible to morbidity and mortality associated with a respiratory infection because they have less cardiopulmonary reserve, as indicated by increased sOEF.
In our study, the calves that were presumed to have died tended to have greater sOEF than calves that survived, indicating that systemic oxygen delivery was reduced relative to oxygen demand. Normal sOEF is approximately 0.2 (Dellinger, 2002; Leach and Treacher, 2002; McLellan and Walsh, 2004); that is, approximately 20% of total oxygen delivered to the systemic microcirculation is consumed by the tissues. A reduction in the rate of oxygen delivery relative to systemic oxygen extraction results in sOEF to exceed 0.3, or 30% (Rady et al., 1994; Olkowski et al., 2005). Hypoxemia and worsening pulmonary hypertension likely impaired cardiac function and, consequently, reduced oxygen delivery to peripheral tissues. The negative effect of hypobaric hypoxia on calf cardiac function has been previously reported (Ruiz et al., 1973; Will and Bisgard, 1975). It is also plausible, however, that a high metabolic oxygen demand is deleterious to calf survival at high altitude. Cardiac output increases in parallel with oxygen demand; consequently, calves with a high oxygen demand may be more susceptible to the adverse effects of further increases in cardiac workload caused by increasing pulmonary arterial pressures. If true, faster-growing calves may be at greater risk of RHF. Although we did not measure growth rate, we find supporting evidence that metabolic oxygen demand may be adversely associated with RHF in studies of broiler chickens, another food-producing species susceptible to pulmonary hypertension. Fast-growing broiler chickens (sOEF ≈ 0.4) and chickens with congestive heart failure (sOEF ≈ 0.56) had a significantly higher sOEF than slower-growing broiler chickens and Leghorns (sOEF ≈ 0.16; Olkowski et al., 2005). Furthermore, fast-growing broiler chickens were significantly more susceptible to RHF than slower-growing broiler chickens (Olkowski et al., 2005).
There are several limitations to our study. First, due to the extensive and rugged mountainous terrain over which the calves were located, the specific causes of the presumed calf mortalities were unknown. In a previous study conducted on the same ranch, however, we found that suckling calf death loss was equally attributable to respiratory disease and right-heart failure (Neary et al., 2013). Second, the results only apply to the ranch studied. The findings do, however, indicate that the screening of breeding stock to identify and remove those individuals with mPAP > 45 mm Hg has the potential to reduce the incidence of calf death loss. Moreover, the findings also suggest that sPAP and pulmonary arterial pulse pressure may be important variables to include, alongside mPAP, in the selective breeding of cattle at high altitude.
LITERATURE CITED
Footnotes
Funding for the study was provided by producer donations to the Integrated Livestock. Management program of the College of Veterinary and Biomedical Sciences, Colorado State University; the Department of Animal Science's John E. Rouse Endowment, Colorado State University; and, the National Institutes of Health (HL-014985, to KRS). The content is solely the responsibility of the authors. The authors have no conflicts of interest to declare.