-
PDF
- Split View
-
Views
-
Cite
Cite
Aditya Upadrasta, Stephen Daniels, Thomas Philip Thompson, Brendan Gilmore, Hilary Humphreys, In situ generation of cold atmospheric plasma-activated mist and its biocidal activity against surrogate viruses for COVID-19, Journal of Applied Microbiology, Volume 134, Issue 8, August 2023, lxad181, https://doi.org/10.1093/jambio/lxad181
- Share Icon Share
Abstract
To provide an alternative to ultra violet light and vapourized hydrogen peroxide to enhance decontamination of surfaces as part of the response to the COVID-19 pandemic.
We developed an indirect method for in situ delivery of cold plasma and evaluated the anti-viral activity of plasma-activated mist (PAM) using bacteriophages phi6, MS2, and phiX174, surrogates for SARS-CoV-2. Exposure to ambient air atmospheric pressure derived PAM caused a 1.71 log10 PFU ml−1 reduction in phi6 titer within 5 min and a 7.4 log10 PFU ml−1 reduction after 10 min when the the PAM source was at 5 and 10 cm. With MS2 and phiX174, a 3.1 and 1.26 log10 PFU ml−1 reduction was achieved, respectively, after 30 min. The rate of killing was increased with longer exposure times but decreased when the PAM source was further away. Trace amounts of reactive species, hydrogen peroxide and nitrite were produced in the PAM, and the anti-viral activity was probably attributable to these and their secondary reactive species.
PAM exhibits virucidal activity against surrogate viruses for COVID-19, which is time and distance from the plasma source dependent.
Plasma-activated mist (PAM) has potential as a decontamination alternative to provide safer surfaces in built environments.
Introduction
The COVID-19 pandemic has presented challenges to healthcare institutions and all workplaces in trying to ensure that the physical environment is as safe as possible for essential and routine activities (Kurnitski et al. 2020). The severe acute respiratory syndrome coronavirus 2 (SARS-CoV-2), is more transmissible than previous coronaviruses (SARS-CoV and MERS-CoV), with rapid spread via contact and droplet routes (Li et al. 2020). In addition, there is a view that it is capable of being spread via aerosols, thus facilitating more widespread transmission over 50 metre distances (Morawska and Milton 2020, Nissen et al. 2020, Greenhalgh et al. 2021, Tang et al. 2021). The circumstances in which transmission is likely to be greatest require further investigation.
Infection prevention and control (IPC) measures in the community and workplaces have included vaccination, hand hygiene, social distancing, wearing masks in confined spaces, and environmental hygiene/decontamination. Conventional approaches to the cleaning of surfaces and equipment in general before COVID-19 concentrated on the use of liquid detergents with or without disinfectants. However, the efficacy of these is dependent on the rigour and thoroughness with which they are applied (Carling 2016). In recent years, the use of walk-away technologies (sometimes referred to as robots) in the healthcare environment has become commonplace, including ultra-violet light or vapourized hydrogen peroxide in addition to routine cleaning/decontamination approaches (Corrêa et al. 2021, Diab-El Schahawi et al. 2021). However, due to potential toxicities, their use requires the evacuation of patients and staff from the area to be decontaminated for a significant period before it is safe for them to return, reducing overall capacity in the system. It is also unclear if the addition of these approaches significantly reduces the acquisition of infections, and whether or not the cost incurred is justified (Diab-El Schahawi et al. 2021).
The spread of SARS-CoV-2 has resulted in considerable morbidity and mortality, especially those in clinically vulnerable categories (Lucey et al. 2021, Read et al. 2021, Waltenburg et al. 2021) and its nosocomial acquisition by healthcare professionals and patients has been disruptive to elective and conventional healthcare services within primary and secondary care. There is a need for additional IPC measures that can interrupt transmission and eradicate the virus from contaminated surfaces and any viral particles present in the air.
A promising disinfection approach, with potential application in hospital, emergency medicine settings and in built areas is the use of cold atmospheric pressure plasma (CAP). The antimicrobial potential of CAP has previously been demonstrated, with reported reductions in the bacterial bioburden on at least two common hospital surfaces (Cahill et al. 2017), inactivation of multi-drug resistant pathogens (Morfill et al. 2009, Cahill et al. 2014, Flynn et al. 2015, Cahill et al. 2017), and the removal of biofilms (Gilmore et al. 2018, Flynn et al. 2019, Alshraiedeh et al. 2020, Mai-Prochnow et al. 2021). Apart from biomedical applications, CAPs have also been used in thin film deposition and modification, plasma catalysis, production of enhanced nanomaterials, polymerization, xenobiotic degradation in wastewater, and air (Gorbanev et al. 2019), and the conversion of CO2 into value-added chemicals (Snoeckx and Bogaerts 2017).
In recent years, plasma-activated liquids (PALs) have gained considerable attention due to their potential biocidal activity. Many studies have illustrated their antimicrobial efficacy against bacterial, fungal, and viral pathogens and further demonstrated their application in suppressing tumour growth in vitro (Rezaei et al. 2019). Despite having great biocidal potential, the stability of PALs is relatively poor and requires freezing for long-term storage to retain their biocidal ability (Tsoukou et al. 2020). PALs produced by CAP generally rely on the fact that reactive species are produced by the action of the plasma either within the water or at the plasma-water interface. Hence, in most cases, the plasma source electrode configuration is designed to ensure efficient interaction between the plasma and the fluid. Examples include plasma jets (Liu et al. 2020) dielectric barrier discharges (Subramanian et al. 2021), and gliding arc discharges (Wartel et al. 2021). The PAL is then used for the desired application such as sterilisation, wound healing, or seed germination.
Our primary interest is in environmental decontamination/hygiene, and hence we are interested in a design that is scalable and practical. To overcome such hurdles, an in-situ plasma-activated mist (PAM) approach is described. Previously, we developed a CAP jet prototype and evaluated it against bacteria commonly associated with hospital infections (Cahill et al. 2014, Cahill et al. 2017). The plasma jet has been modified to generate PAM that could be used to decontaminate surfaces and potentially also the air in workplaces and public indoor spaces, without requiring the evacuation of any individuals or equipment. In our approach, we first nebulized a water source, and subsequently transported this nebulized water through an active plasma region in order to activate the droplets. We used a sequence of plasma jets in series that interacted with the nebulized water droplets as they travelled through the system before exiting at a nozzle arrangement into the treatment area. This method delivers the plasma indirectly via mist and as any temperature increase is minimal, it can be safely used for biomedical applications, e.g. on thermosensitive materials, and burn wound infections.
To work with mammalian pathogenic viruses such as SARS-CoV, MERS-CoV, Influenza, and Ebola, etc. requires a biosafety level 4 laboratory with well-trained lab personnel to evaluate sampling efficiency, persistence and disinfective strategies. For safety concerns, non-pathogenic virus variant bacteriophages such as phi6 (Tseng and Li 2006, Adcock et al. 2009, Lin and Marr 2017, Fedorenko et al. 2020, Yang et al. 2022) MS2, phiX174 (Nastasi et al. 2022, Schupp et al. 2022, Shahin et al. 2022) and others have been used as acceptable surrogates to evaluate the anti-viral activities in many studies. Here, we describe the effect of the PAM on the viability of viruses commonly employed as conservative surrogates for SARS-CoV-2 and other non-eveloped enteric viruses (an outer lipid enveloped phage phi6 and two outer protein coat phages MS2 and phiX174, respectively) under a range of parameters, including the distance of the virus from mist generation and an initial assessment of the reactive species produced by the mist.
Materials and methods
Bacteriophages and host growth conditions
Bacteriophages phi6 (DSM-21518), phiX174 (DSM-4497), and MS2 (DSM-13767) and their bacterial hosts Pseudomonas syringae (DSM-21482), and Escherichia coli (DSM-13127 and DSM-5695) were purchased from the German Collection of Microorganisms and Cell Cultures DSMZ. Pseudomonas syringae was routinely cultured at 30°C in Tryptic Soy Broth (TSB, Merck) and E. coli (DSM-13127 and DSM-5695) was cultured at 37°C in Luria-Bertani broth (LB, Sigma–Aldrich). The bacteriophages phi6, MS2, and phiX174 were propagated using standard broth or solid agar overlays (Kropinski et al. 2009). The resultant phage propagation was filtered through a 0.22-µm syringe filter (GVS, Italy), and were stored at 4°C until use.
Atmospheric pressure plasma jet (APPJ)
The APPJs used in this study for plasma generation were configured and developed as previously described, using a single electrode operated with power supplied at ∼25 W (Cahill et al. 2014) (Fig. 1). The plasma jet was operated at atmospheric pressure, with room air supplied at 9 standard litres per minute as the plasma inducing gas.

Schematic of PAM set up with sequentially aligned two APPJs. The exposed surrogate viruses were placed on the Jiffy Jack Lab Stand (Z-stand) at distances 5–30 cm away.
Optical emission spectroscopy (OES) of air plasma plume
The optical emission spectra of air plasma plume was collected in open air using an Ocean Optics HR4000 (Ocean Insight) connected with F600 VIS-NIR fiber optic cable (StellarNet Inc, USA) of 600 µm diameter for a wavelength range of 400–2200 nm. The optical fiber was fixed at a distance of 5 mm perpendicular from the plasma plume axis and emission spectra were acquired through SpectraGryph software (Menges 2021).
Generation of mist and treatment with plasma
An ultrasonic cold mist maker (SMAUTOP Aluminium Mist maker) was placed in a water tank filled with deionized water and this was used for mist generation. The generated mist was channelized to a metal mixer with the aid of a 12 V DC centrifugal fan (ebmpapst, RV40-18/12H) and treated with two sequentially arranged APPJs to obtain PAM (Fig. 1).
The antiviral efficacy of the PAM was later tested. Before application of PAM, the phage stocks were diluted in SM buffer (Russell and Sambrook 2001) and adjusted to obtain the desired intital phage concentration of ∼7.5–8 log10 plaque forming units, (PFU ml−1). The 0.1 ml−1 aliquot of diluted phage suspension was spotted as 20 × 5 µl−1 droplets on to a small 35 mm petri dish and exposed to PAM at the predetermined time intervals 3, 5, 10, 15, and 30 min. Furthermore, we also evaluated the spatial distribution of PAM using phage phi6 by placing the sample on a jiffy jack laboratory stand (Z-stand) at various heights to achieve distances of 5, 10, 20, and 30 cm from the PAM outlet. After exposure to PAM, the samples were recovered by adding 0.9 ml−1 of SM buffer and pipetted multiple times on all the spots by holding the petri plate in a slightly slanted position, and from that recovered samples of the serial dilutions were performed to estimate the viable log10 PFU ml−1 using double agar overlay assay. For every experiment, the diluted phage suspension was assayed to estimate the exact phage titer which served as a control.
The survival of exposed phages was determined using the standard double agar overlay method (Kropinski et al. 2009). Briefly, PAM exposed samples were serially diluted in SM buffer and an 0.1 ml−1 aliquot was mixed with exponentially grown bacterial host culture (∼0.3 OD600 nm) with 4 ml−1 of corresponding 0.45% (w/v) soft agar supplemented with 5 mm l−1 of CaCl2 and MgSO4.6H2O (Sigma), mixed by gentle vortexing and then poured over basal 1.5% agar plates. During phage viability estimation after treatment with PAM, a 0.1 ml−1 aliquot was plated in all dilutions, and the remaining (0.8 ml−1) of the lower dilution was also used for the overlay assay to ensure the presence of no viable phage. Phage survival kinetics are represented as log10 reduction in plaque forming units (PFU ml−1) with error bars representing standard deviation.
pH, ORP, and conductivity
The PAM condensate was collected for around 45 min on an ice bath in a glass bottle and later used to measure the aqueous chemistry. The pH, oxidative-reductive potential (mV) and conductivity (uS/cm) parameters were measured with a multichannel water analysis metre (Multi 3630 IDS WTW, Xylem Analytics, GmbH, Germany) using pH (SenTix 980), ORP (SenTix ORP-T 900) and conductivity (TetraCon 925) probes.
Quantitative measurement of nitrite, hydrogen peroxide, and dissolved ozone
To measure some of the reactive species, the PAM was collected in a 35 mm petri dish and placed on an ice bath at predetermined time intervals. The NO2− concentration was measured using Griess reagent colorimetric assay kit (Enzo Biosciences, Cat No: ADI-917–010). The kit involves the enzymatic conversion of nitrate to nitrite, by the enzyme nitrate reductase, followed by the colorimetric detection of nitrite as a coloured azo dye product of the Griess reaction. The absorbance was read at 540 nm using a compact microtiter plate reader. The hydrogen peroxide concentration was estimated using colorimetric kit (Enzo Biosciences, Cat No ADI-907–015), according to the manufacturer's instructions. This kit has a colour reagent that contains a dye, xylenol orange, which reacts with sorbitol and ammonium iron sulfate in acidic solution to produce a purple colour, proportional to the concentration of hydrogen peroxide. All samples were estimated by comparison with their corresponding standard curves.
To quench the reaction of nitrates with H2O2 at acidic pH (Lukes et al. 2014), the small petri dishes were pre-misted for 3 min using a handheld nebulizer (MicroAir mesh nebulizer U100, Omron Healthcare) with 10 mm l−1 sodium azide (SA) (Cat No: S2002, Sigma) solution prior to the collection of PAM. The collected samples at 3, 5, 10, 15, and 30 min were immediately kept on ice and analyzed within 30 min for hydrogen peroxide concentration.
Dissolved O3 concentration was estimated using spectrophotometric indigo method as described elsewhere (Bader and Hoigné 1981, Gordon et al. 2000, Gehr et al. 2003). The concentration of aqueous ozone was determined by the decolourization of indigo trisulfonate (absorbance at 600 nm). The change of absorbance vs ozone added was 2.0 ± 0.1 × 104 mol l−1 cm−1 and was independent of the concentration of aqueous ozone in the range of 0.005–30 mg l−1 (Bader and Hoigné 1981). Gaseous ozone in the PAM was measured using UV-106-M Ozone Analyzer (2B technologies, Gas Sensing, USA). All absorbance readings were measured using compact absorbance 96 plate reader (ENZ-INS-A96, Enzo Biosciences). All experiments were replicated twice with two duplicates.
Data analysis
All obtained data was analysed using Excel (Windows 10, Microsoft, USA) and GraphPad Prism version 9 (GraphPad, USA). The statistical significances of measured values at different time points were determined by one-way Analysis of Variance (ANOVA) using Tukey's multiple comparison test in GraphPad Prism version 9 (GraphPad, USA). Differences at P < 0.05 were considered significant.
Results
Physicochemical properties of water and mist
Deionized water (Barnstead II, Thermo Scientific) was used to generate the mist. The initial pH, oxidation-reduction potential (ORP, mV) and conductivity (uS/cm) measurements were assessed using PAM condensate collected for ∼45 min (Table 1). The initial pH recorded was 6.29 ± 0.41, which fell to 3.23 ± 0.11 in PAM, whereas the ORP and conductivity increased from 331.9 ± 4.39 to 667.43 ± 27.41 mV and 127.03 ± 98.74 to 500 ± 45.45 uS/cm, respectively, indicating the dissolution of reactive species in the PAM.
pH, ORP, and conductivity measurements of the PAM The values are an average of at least three individual measurements in duplicate with SD.
. | pH . | ORP (mV) . | Conductivity (uS) . |
---|---|---|---|
Deionized Water | 6.29 ± 0.41 | 331.9 ± 4.39 | 127.03 ± 98.74 |
PAM | 3.23 ± 0.11 | 667.43 ± 27.41 | 500 ± 45.45 |
. | pH . | ORP (mV) . | Conductivity (uS) . |
---|---|---|---|
Deionized Water | 6.29 ± 0.41 | 331.9 ± 4.39 | 127.03 ± 98.74 |
PAM | 3.23 ± 0.11 | 667.43 ± 27.41 | 500 ± 45.45 |
pH, ORP, and conductivity measurements of the PAM The values are an average of at least three individual measurements in duplicate with SD.
. | pH . | ORP (mV) . | Conductivity (uS) . |
---|---|---|---|
Deionized Water | 6.29 ± 0.41 | 331.9 ± 4.39 | 127.03 ± 98.74 |
PAM | 3.23 ± 0.11 | 667.43 ± 27.41 | 500 ± 45.45 |
. | pH . | ORP (mV) . | Conductivity (uS) . |
---|---|---|---|
Deionized Water | 6.29 ± 0.41 | 331.9 ± 4.39 | 127.03 ± 98.74 |
PAM | 3.23 ± 0.11 | 667.43 ± 27.41 | 500 ± 45.45 |
OES of atmospheric air plasma plume
The optical emission spectra of ambient air plasma are depicted in Fig. 2. The obtained spectrum was predominant with N2 series, with N2 second positive system at 315, 336, 357, and 380 nm, and N2 first negative system at 390 nm visible from air plasma discharge (Kovačević et al. 2017, Zhou et al. 2018, Rathore and Nema 2021). A weak emission line at 281 nm corresponds to nitrogen oxide (NO), as a result of the reactive collision between nitrogen and oxygen molecules (Rathore and Nema 2021), and atomic oxygen peaks at 777 and 844 nm were also identified (Kovačević et al. 2017).
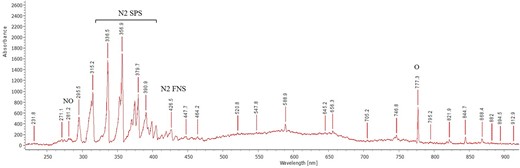
Antiviral activity of PAM
Figure 3a–c illustrate the impact of the PAM on the SARS-CoV-2 and influenza virus surrogates, phi6, phiX174, and MS2. For phi6, assays were carried out when the PAM source was 5, 10, 20, and 30 cm away from the surface contaminated with the surrogate virus suspensions. For phiX174 and MS2, the experiments were carried out at distances of 5 and 10 cm. The intial inocula (also serving as controls) for each three viruses were ∼6.5–7.5 log10 PFU ml−1. For phi6, a 7.4 log10 PFU ml−1 reduction was observed as early as 10 min after exposure when the mist source was 5 and 10 cm away from the virus sample; at 20 cm distance, an exposure time of 30 min led to a 7.4 log10 PFU ml−1 reduction. At 30 cm, a 30 min exposure led to a 1.16 log10 PFU ml−1 reduction in the viral titer. With MS2, when PAM was at a 5 cm, exposure times of 15 min, and 30 min led to a 1 and 3.1 log10 PFU ml−1 reduction, respectively. Furthermore, at 10 cm, a 1.64 log10 PFU ml−1 reduction was observed at 30 min. With phiX174, a distance of 5 and 10 cm, resulted in a 1.26 and 0.68 log10 PFU ml−1 reduction at 30 min, respectively. No significant reduction in phage titers observed when exposed for 30 min at 5 cm distance to control-mist (Fig. 3d, mist without plasma).
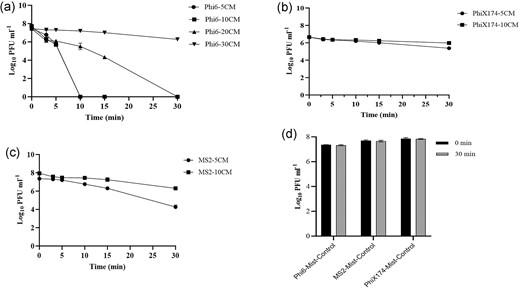
Inactivation kinetics of PAM against bacteriophages: (a) phi6 with PAM sourced at distances of 5, 10, 20, and 30 cm, (b) MS2 with PAM sourced at distances of 5 and 10 cm, (c) phiX174 with PAM sourced at distances of 5 and 10 cm, and (d) exposed to control mist at 5 cm for 30 min. The time 0 min serve as starting inoculum control before PAM treatment. The average reduction in log10 plaque forming units (PFU ml−1) with error bars representing SD were plotted.
Measurement of reactive oxygen and nitrogen species (RONS)
The dissolution of CAP-generated reactive species in water mist was quantified from the commencement of mist generation at various time intervals, i.e. 3, 5, 10, 15, and 30 min. The measured concentration of nitrites (NO2−) and hydrogen peroxide (H2O2) varied according to the time intervals (Fig. 4a–c). The mean concentration of nitrite (NO2−) was 1.61 mmol l−1 (Fig. 4a). A decay in H2O2 concentration was observed over time, with a decrease from 77.4 µmol l−1 at 3 min to 6.68 µmol l−1 at 30 min (P ≤ 0.01) (Fig. 4b). To investigate the possible interaction between nitrites and H2O2 at acidic pH, petri plates were pre-misted with NO2− scavenger SA, prior to PAM sample collection. With pre-misted SA, the H2O2 concentration was stable between 3 and 5 min, and significantly dropped from 3 to 30 min (P ≤ 0.01) (Fig. 4b), the mean concentration being 152 ± 4.07 µmol l−1. The mean concentration of dissolved ozone (O3) was 0.14 ± 0.01 mg l−1 (Fig. 4c) and dropped at 15 and 30 min (P ≤ 0.05).
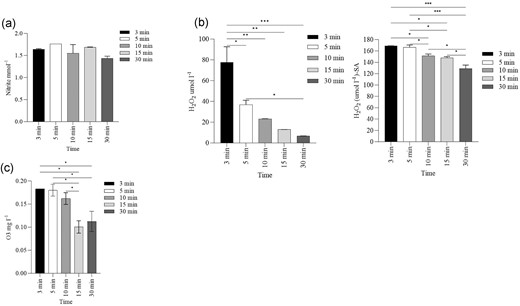
Measured concentrations of (a) nitrite (NO2− mmol l−1), (b) hydrogen peroxide (H2O2 µmol l−1) with and without the presence of SA, and (c) ozone (O3 mg l−1) over 3–30 min. The values are an average of two replicate experiments with two duplicates and error bars represents SD. The statistical significances of measured values at different time points were determined by one-way Analysis of Variance (ANOVA) using Tukey's multiple comparison test (* P ≤ 0.05, ** P ≤ 0.01, and *** P ≤ 0.001).
Discussion
The anti-viral efficacy of CAP and PAL varies greatly depending on jet types, electrode configuration, and the supplied gas (helium, argon, ambient air, and ad-mixtures of gases). For example, the applied voltage (kV), electrode system, and carrier gas do have a considerable effect and influence overall biocidal activity (Schutze et al. 1998, Weltmann et al. 2010). In this study, we used an indirect application of plasma to activate the mist using ambient air as a carrier gas to achieve proof of concept. The presence of PAM was confirmed with the observed decrease in pH from 6.3 to 3.2 and an increase in ORP (>600 mV) and conductivity (500 uS/cm−1) (Table 1), which suggests dissolution of plasma-generated reactive species (NO oxides into nitrous acid and nitric acid) from the gaseous phase into the liquid (Ikawa et al. 2010). ORP monitoring in water treatments provides a rapid single-value assessment of the disinfection potential. An ORP value between 600 and 700 mV showed highest killing activity against bacteria, yeasts and some-susceptible types of spore-forming fungal species (Suslow 2004). In general, PALs with higher ORP > 500 mV exhibited greater biocidal activity (Zhao et al. 2020), occuring as a result of the accumulation of more oxidizing species liberated from RONS formation. The formed oxidative species and active ions compromise the cell membrane potentia,l which leads to the damage and leakage of cell constituents, resulting in the inactivation and death of bacteria and viruses (Liao et al. 2007, Ikawa et al. 2016, Zhao et al. 2020). Similarly, PAW displays high electrical conductivity potential, due to the dissolution of RONS in the liquid-gas interphase, thereby increasing the concentration of active ions resulting in an increase in conductivity. The PALs with high ORP and conductivity displayed potential biocidal activity and can potentially be used as a disinfectant and to arrest the cancer cell growth etc. (Weltmann et al. 2010, Rezaei et al. 2019).
The OES of air plasma, ranging from 200 to 900 nm, revealed more prominent N2 lines, dominated by strong single positive system N2 and atomic oxygen lines at 777 and 844 nm, demonstrating the formation of gaseous RONS and their successive dissolution into the aqueous phase. The spectra were obtained with ambient air as an inlet gas, with the N2 series being more prominent than the other reactive species.
We have demonstrated that PAM has anti-viral activity against SARS-CoV-2 and influenza virus surrogates, even if it was not possible for safety reasons to assess SARS-CoV-2 itself or to assess in a clinical environent. There was a difference in PAM virucidal activity among the tested bacteriophages; phi6 was more rapidly inactivated when compared to MS2 and phiX174. With phi6 there was a 7.4 log10 PFU ml−1 reduction achieved after 10 min when the mist source was at a distance of 5 cm from the substrate, as the distance of the source increased to 30 cm, a 1 log10 PFU ml−1 reduction was achieved (Fig. 3a). For MS2 and phiX174, the reductions were less when compared to phi6, e.g. just a 1 log10 PFU ml−1 reduction against phiX174 after 30 min of exposure to PAM. Regarding the biocidal activity of the PAM, the further the exposure distances (i.e. from 5 to 20 cm) of the surrogate viruses from the PAM source, the lower the log10 PFU ml−1 reductions observed. At greater distances, longer exposure times were required to achieve antiviral activity, probably due to the diffusion and quenching of short-lived reactive species in air before they get in contact with the actual biological target and the accumulation of more reactive species on the target surface leading to high viral inactivation.
Previous studies have also reported that a decrease in the density of reactive species with increasing distance from the nozzle (Van Gessel et al. 2013, Van Ham et al. 2014, Adhikari et al. 2019). Therefore, an enhanced virucidal activity could be achieved if there is sufficient contact time for the reactive species to interact with the viral particles. Moreover, PAM exerted similar inactivation dynamics to that at 5 cm, when the PAM was channelled through a closed pipe, 60 & 75 cm in length from the viral sample (data not shown). This observation indicates that PAM retains biocidal activity even after travelling ∼1 m from the outlet in a closed space, when compared to an open setting where there is more diffusion and quenching of reactive species into the outer atmosphere, eventually diminishing their effective concentration.
The difference in killing efficacy may be linked to the aggregation kinetics and overall surface charge among the protein-coated phages, hence, the variation in biocidal activity among the tested viruses. In general, enveloped viruses are more sensitive to biocidal species, probably due to the presence of an outer lipid membrane, which is prone to lipid peroxidation and compromising the membrane potential (Filipić et al. 2020). In contrast, non-eveloped viruses such as MS2 and phiX174 exhibit hydrophobic nature (Heldt et al. 2017) and are more resistant to disinfectants and needed longer treatment times. It has been shown that the lipophilic phi6 was more susceptible to disinfection than MS2 against different tested germicides (Woolwine and Gerberding 1995). Moreover, 3% (v/v) hydrogen peroxide had the least virucidal activity; <1 log10 PFU ml−1 reduction only was observed against phi6 and MS2 phages (Woolwine and Gerberding 1995). This observation also indicates that the inactivation of viruses obtained with PAM and PALs is not solely linked to one particular reactive species, and can be attributed to the synergistic action of various reactive nitrogen and oxygen species and their highly volatile secondary intermediate species.
The antiviral activity of CAP has been attributed to the plasma-generated reactive species, which damages surface coat proteins and cellular nucleic acids via oxidation and degradation mechanisms (Yasuda et al. 2010, Tanaka et al. 2013, Wu et al. 2015, Mizuno 2017, Guo et al. 2018). The surface chemistry of the virus also plays a role in response to changes in the environmental parameters, and these are mainly via pH and dissolved ionic substrates. A decrease in the surrounding pH can trigger viral aggregation (Floyd and Sharp 1978a, b; Langlet et al. 2007, Langlet et al. 2008a, Szermer-Olearnik et al. 2017, Pradhan et al. 2022), a known phenomenon that enhances their survival rate to combat adversity. Viruses obtain their net charge from different functional groups that constitute their phospholipid envelop or capsid proteins. In general, the isoelectric point of viruses ranges from 1.9 to 8.4 and they tend to aggregate in aqueous environments of pH near to their pI (Michen and Graule 2010). In the case of MS2, the aggregation occurs when the pH is near their isoelectric point (pI) i.e. 3.5–3.9, and this aggregation is independent of the ionic strength of the aqueous environement (Langlet et al. 2008a, Langlet et al. 2008b), which is close to the observed pH of the PAM (Table 1). Furthermore, MS2 possess high hydrophobic qualities when compared to the other viruses (Langlet et al. 2008a). The hydrophobic nature of phage MS2 would certainly aid the phage outer membrane to remain intact and a decrease in outer membrane pore size eventually minimizes the access to the generated primary and secondary reactive species. In the event of viral aggregation, there will be an electrostatic repulsive force among the capsid proteins resulting in their denaturation and therefore inducing a significant reduction in their outer capid pore size (Langlet et al. 2008b). This phenomenon of decreasing membrane pore size and aggregation of virus could be one of the possible reasons that such viruses required longer exposure and interaction times to disinfectants to achieve maximum inactivation efficacies. Moreover, severe aggregation of T4 bacteriophages has been reported when they were treated with PAW (Guo et al. 2018). Furthermore, a 6.0 log10 PFU ml−1 reduction in feline calicivirus was observed after two hours of exposure to plasma treatment (Aboubakr et al. 2015). In another study, the destruction of bacteriophage lambda coat protein was demonstrated as a result of cold plasma treatment, but the effect on bacteriophage DNA was minimal. However, inactivation is greater the longer the exposure to cold plasma (Yasuda et al. 2010). Moreover, higher viral inactivation rates were achieved with increased power levels while generating the plasma (Wu et al. 2015). Importantly, in many studies, either direct exposure of biological targets (bacteria or virus in liquid or dried formats) to dry plasma by placing the sample as close as possible to the electrode (0.2–0.5 cm away) and/or by treatment with PAL were used to evaluate biocidal activity. Of note, in the current PAM set-up, the travel distances of PAM from jets 1 and 2 to the actual PAM outlet were 46 and 34 cm, respectively, and the distance between jets 1 and 2 was 12 cm (Fig. 1). Therefore, the travel distance of PAM from the actual PAM source outlet should be considered. In total, 34 cm should be taken into account when considering the total distance travelled by PAM and its viral inactivation efficacy. However, the antiviral activity was tested at variable distances from the PAM outlet and was remained active throughout.
The NO2− concentration in the PAM condensate was relatively consistent over time (Fig. 4a); however, other studies have reported an increase in accumulated nitrites and H2O2 in PAL over time (Kovačević et al. 2017, Zhou et al. 2018, Wu et al. 2020). In such studies, either treating small volumes of water or using a batch reactor, the water undergoes plasma treatment for longer periods which eventually results in higher concentrations of dissolved reactive species. In contrast to such PAL methods, we nebulized the water from the reservoir continuously to generate a fine mist, and treated this mist with plasma to obtain the PAM. The persistence in NO2− concentration over time may be due to the very short interaction time between the plasma with the mist, as the dissolution of reactive species into the mist may be minimal given the exposure times described herein. The reactive species are formed in a continuum accumulated on the surface of the petri dish and were readily available to react with other co-formed reactive species. This will lead to the generation of short lifespan secondary reactive species such as peroxynitrite, peroxynitrous, and peroxone (Machala et al. 2013, Sysolyatina et al. 2020), which may balance the sum of their precursor species.
Concentrations of H2O2 in the PAM decayed rapidly from 3 to 30 min, however, when measured in the presence of nitrate quencher SA, but the concentrations of H2O2 was stable upto 10 min and gradual reduction was observed over 30 min (Fig. 4b). The possible interaction of peroxides with nitrites at lower pH to form intermediate species peroxynitrite (ONOO−) has been previously documented (Lukes et al. 2014). In an acidic environment, peroxynitrous acid can gain access to the lipid bilayer of the bacterial outer cell membrane in order to initiate lipid and protein peroxidation (Radi et al. 1991). Zhou et al., demonstrated the crucial role of ONOO− as a biocidal agent, and demonstrated a 4-log10 CFU ml−1 reduction of E. coli when exposed to PAW, while simulated reactive species in solution failed to exhibit similar anti-microbial activity (Zhou et al. 2018).
The concentration of dissolved O3 with a mean concentration of 0.14 mg l−1 was recorded, no significant difference in concentration was observed upto 5 min and the concentration was gradually reduced from 10 to 30 min. However, using a UV-based ozone monitor, 0.04 ppm of O3 was recorded in the PAM and 0.01–0.02 ppm in the gaseous phase of plasma. The solubility of gaseous ozone in liquid media depends on various factors such as discharge type, temperature and pH (Mouele et al. 2021). In a water medium, O3 may react with other dissolved species, or it may decompose to form hydroxyl radicals (·OH) as secondary oxidants (Staehelin and Hoigne 1985), and this may be one of the possible reason for the gradual reduction in concentrations of ozone we observed in PAM.
In the present study, where the viruses were indirectly exposed to plasma via plasma activated mist and where the inactivation efficiency is not a direct result of either UV irradiation or heat generated from the plasma as previously highlighted (Ikawa et al. 2010), the virucidal activity may be attributed to the reactive species formed at the plasma-mist interface.
Other oxygen-related reactive species such as singlet oxygen and hydroxyl anion can also play a pivotal role in antimicrobial activity. This is due to the presence of more hydrogen molecules sourced from the mist and the mist-plasma interaction leading to the formation of singlet oxygen and hydroxyl radicals. Singlet oxygen oxidizes outer membranes in bacteria/viruses made up of either proteins or lipids (Maisch et al. 2007) leading to their death. Furthermore, it was shown that bacteriophage MS2 was inactivated by singlet oxygen generated with UV-irradiated fullerol, resulting in significant oxidative modification to the capsid proteins (Hotze et al. 2009). The reaction between the peroxynitrite anion and hydrogen peroxide also leads to the release of singlet oxygen (Di Mascio et al. 1994). The generation of singlet oxygen in the gaseous plasma phase has been reported, where argon was used as a carrier gas with a 1% admixture of molecular oxygen (Jablonowski et al. 2018). In our study, the carrier gas was atmospheric air and the photolysis of oxygen molecules may release singlet oxygen in the gaseous plasma phase. Furthermore, the OES of the gaseous phase plasma had a peak at 777 and 844 nm (Fig. 2), i.e. belonging to atomic oxygen and thus demonstrating the existence of excited oxygen series, which may generate the oxygen-mediated free radicals.
However, the complexity associated with the APPJs, and their sensitivity to subtle variations in configuration and operational parameters can undoubtedly produce variable results, and this may explain different results in other studies. Therefore, comparisons between studies are fraught with challenges and at this point in time, it may be impractical to draw specific conclusions as to their relative effectiveness.
A feature of the COVID-19 pandemic has been the high transmissibility of the virus, with some evidence that there may be airborne spread as well as spread by contact and by droplets (Morawska and Milton 2020, Meyerowitz et al. 2021, Paton et al. 2021). While simple measures may be effective in reducing droplet spread, controlled air ventilation systems may be required for areas or units where aerosol spread may occur (Humphreys 2021). Surface spread is theoretically capable of being controlled by effective hospital hygiene/decontamination procedures, but these are variable. Recently, ‘robots’ or walk-away technology with ultra violet light or vapourized hydrogen peroxide have been promoted to enhance routine cleaning. However, these are not without their shortcomings including potential toxicities with both, and shadowing with ultraviolet light (Diab-El Schahawi et al. 2021).
Our PAM prototype was developed and designed to deliver a consistent PAM with anti-viral activity. Key features of our prototype include the absence of a requirement for defined storage conditions as it generates PAM in situ, and it can be re-engineered to maximize the RONS production for rapid biocidal action. Further modifications to the current set up, including to the jet parameters and the PAM interaction duration, will most likely improve the efficiency of this method for extended use. Of note, supplying controlled humidified air for generating plasma may also enhance the biocidal potential of the PAM as some studies have shown that humidification of dry air used for plasma generation enhanced the concentration of RONS (Chen et al. 2016).
Cold plasma-based disinfection shows promise and appears to be much safer than other walk away strategies, which are currently in practice. We showed that a PAM prototype is a potentially versatile tool with significant virucidal activity against phi6, MS2, and phiX174 bacteriophages. The indirect delivery of plasma via mist activation has great potential and with this approach, PAM can be delivered in situ, containing active RONS at non-toxic concentrations. However, further studies are warranted against a greater panel of pathogenic viruses and simulating aerosol experiments to demonstrate the true potential of PAM as a safer alternative tool for the decontamination of surfaces and potentially air. Then, it can be evaluated in a clinical or occupational setting to determine if it confirms its potential.
Acknowledgement
The authors are grateful to Dr. Lakshmi Prapurna Yallapragada, CSIR-IICT, Hyderabad, India, and Dr. Jim Conway, Dublin City University for their valuable insights and discussion.
Ethics declaration
No animals, patient samples or human tissue were used in this research. No patient data was collected or analysed. All research involved in vitro laboratory experiments.
Conflicts of interest
H.H. has been in receipt of research grants from Pfizer (Ireland) and Astellas (Ireland) in recent years, and has been paid a consultancy fee by Pfizer (Ireland). All other authors have no conflicts of interest to declare.
Funding
The Science Foundation Ireland funded this research through Grant No 20350A01.
Author contribution
Aditya Upadrasta (Investigation, Methodology, Writing – original draft, Writing – review & editing), Stephen Daniels (Conceptualization, Funding acquisition, Investigation, Resources, Supervision, Writing – review & editing), Thomas Philip Thompson (Supervision, Writing – review & editing), Brendan Gilmore (Supervision, Writing – review & editing), and Hilary Humphreys (Conceptualization, Funding acquisition, Investigation, Supervision, Writing – review & editing)
Data Availability
The authors confirm that the data supporting the findings of this study are available within the article.