-
PDF
- Split View
-
Views
-
Cite
Cite
Erika Kertes, László Péczely, Tamás Ollmann, Kristóf László, Beáta Berta, Veronika Kállai, Olga Zagorácz, Anita Kovács, Ádám Szabó, Zoltán Karádi, László Lénárd, The role of pallidal substance P and neurokinin receptors in the consolidation of spatial memory of rats, International Journal of Neuropsychopharmacology, Volume 28, Issue 1, January 2025, pyaf002, https://doi.org/10.1093/ijnp/pyaf002
- Share Icon Share
Abstract
The tachykinin substance P (SP) facilitates learning and memory processes after its central administration. Activation of its different receptive sites, neurokinin-1 receptors (NK1Rs), as well as NK2Rs and NK3Rs, was shown to influence learning and memory. The basal ganglia have been confirmed to play an important role in the control of memory processes and spatial learning mechanisms, and as part of the basal ganglia, the globus pallidus (GP) may also be involved in this regulation. SP-immunoreactive fibers and terminals, as well as NK1Rs and NK3Rs, were shown to be present in the GP.
The present study aimed to examine whether the SP administered into the GP can influence spatial memory consolidation in the Morris water maze (MWM). Therefore, male Wistar rats received a post-trial microinjection of 0.4 µLf 10 ng SP, 100 ng SP, or vehicle solution. The possible involvement of pallidal NK1Rs and NK3Rs in the SP effects was also studied by applying WIN51708 for NK1R antagonism and SR142801 as a selective NK3R antagonist.
Our results showed that the lower dose of SP significantly decreased escape latency on the second day compared to control animals, while the higher dose was ineffective. Prior treatment with the NK1R antagonist WIN51708 could not block, while the NK3R antagonist SR142801 inhibited the effects of SP on memory consolidation in the MWM.
Our results are the first to demonstrate that SP improves consolidation of spatial memory in the GP, and this effect is mediated through NK3Rs but not NK1Rs.
This study investigated the role of a neuropeptide, substance P (SP) in spatial learning mechanisms when injected into the globus pallidus of rats. The involvement of the receptors of SP, namely, neurokinin-1 and neurokinin-3 receptors in this process was also examined. An important finding is that SP could enhance spatial memory consolidation, and this effect was blocked only with a neurokinin-3 receptor antagonist but not with a neurokinin-1 receptor antagonist. The effects of SP are mediated mainly via neurokinin-1 receptors, no data indicate a similar role for neurokinin-3 receptors so far. Our results, therefore, are new and unexpected findings and might contribute to a better understanding of the role of the neurokinin-3 receptor system in cognitive processes. The possible role of neurokinin-3 receptors in human intellectual decline is supposed; therefore, our results might contribute to finding a future treatment target for dementia.
INTRODUCTION
The undecapeptide substance P (SP), belonging to the tachykinin neuropeptide family, appears to function as a neurotransmitter and/or neuromodulator in the mammalian nervous system.1,2 Administration of SP could affect a wide range of behaviors including general activity, anxiety, and rewarding/reinforcing processes.3-7 Experimental data suggest an important role of SP in learning and memory as well, it can improve memory consolidation in different brain regions.3,8-10 Furthermore, systemic administration of SP could facilitate place learning of adult and aged rats, or rats with hippocampal lesions.11-13
The globus pallidus (GP), as an important structure of the basal ganglia, in addition to its motor functions, also plays a role in the central regulation of perceptual, motivational, as well as reward-related processes,14-16 and its role in learning and memory is also suggested by several data.17-20 Growing evidence indicates that, in addition to the hippocampus, the cortex-basal ganglia loop plays an important role in spatial learning,21-23 and the involvement of the GP in spatial learning mechanisms has also been supported by lesion studies.24,25 A number of studies have confirmed that the ventromedial and dorsolateral parts of the GP (vmGP and dlGP, respectively) are morphologically and functionally different. While the dlGP is involved mainly in action preparation and execution, the vmGP is supposed to play a role in motivational and learning processes.19,21-23,26-28
Within the mammalian central nervous system, the presence of SP in the pallidal complex has been proven, SP immunoreactivity was found in the GP in moderate to high concentrations.29,30 SP can bind to all 3 types of tachykinin receptive sites classified in mammalian species, namely to neurokinin-1, -2, and -3 receptors (NK1Rs, NK2Rs, and NK3Rs, respectively); however, its affinity to NK1Rs is much higher than to NK2Rs or NK3Rs.1,29,31,32 Rat GP contains NK1Rs in higher density, NK3Rs were also found but in a somewhat lower amount, while the presence of NK2Rs was not detected.32-34 According to previous findings, both NK1Rs and NK3Rs may play a role in processes related to learning and memory.8,10,35-39
Based on the aforementioned findings, we hypothesize that SP has a facilitating effect on spatial memory consolidation when injected into the vmGP. Thus, in the present experiments, the behavioral consequences of bilateral SP microinjections into the GP were examined in the Morris water maze (MWM) test. The effects of SP in various paradigms were dose-dependent3,4,6,10; therefore, SP was injected in 2 different doses. In most cases, SP acts through NK1Rs; however, the role of NK3Rs cannot be ruled out. To study the involvement of these receptors in the mediation of the effects of SP, we used non-peptide NK1R and NK3R antagonists, WIN51708, and SR142801, respectively, since these compounds are highly selective, and several reports have shown their effectiveness.6,10,37,40-44
MATERIALS AND METHODS
Subjects
One hundred twenty-four adult male Wistar rats weighing 280-320 g at the beginning of the experiments were housed individually and cared for in accordance with institutional (Animal Welfare Committee of Pécs University, Medical School; license No.: BA02/2000-61/2017), national (Law XXVIII, 1998, Government Decree, 40/2013. (II.14) ), and international standards (European Community Council Directive 86/609/EEC; 1986, 2006; European Directive 2010/63/EU of the European Parliament and the Council; National Institutes of Health Guidelines for Laboratory Animals, 1997). The minimal number of rats needed for the evaluation of the results was used in the experiments, and all efforts were made to provide conditions for the well-being and to minimize the distress and suffering of the animals, according to the ARRIVE guidelines. Rats were kept in a temperature-, humidity-, and light-controlled room (22 ± 2 °C; 55%-60%; 12:12-hour light-dark cycle with lights on at 7:00 AM) for 1 week before surgery. Standard laboratory chow pellet food (Szindbád Ltd) and tap water were available ad libitum. All behavioral tests were done during the rats’ daylight period, between 08:00 AM and 5:00 PM.
Surgery
Stereotaxic operations were carried out under combined anesthesia induced by intraperitoneal injection of a mixture of ketamine and diazepam (Calypsol, Richter Gedeon, Hungary and Diazepeks, AS Grindeks) mixed in a ratio of 4:1 (ketamine: 80 mg/kg body weight, diazepam: 20 mg/kg body weight). During the operation, rats were implanted with 22-gauge guide cannulas bilaterally, directed toward and 1 mm above the target area in the ventromedial part of the GP, the coordinates were as follows: AP: −1.4 mm and ML: ± 3.4 mm, relative to Bregma, DV: −6.4 mm from the surface of dura mater, according to the rats’ stereotaxic atlas.45 Cannulas were fixed to the skull with 3 stainless steel screws and dental acrylic (Duracryl, SpofaDental a.s.). Guide cannulas were occluded with stainless steel obturators, 27 gauges in diameter, when not being used for injection. Animals were allowed a minimum of 6-day postoperative recovery before experiments commenced. During this period, rats were handled daily to get accustomed to hand-restraint thus reducing the stress of the injection procedure.
Drugs and Injection Procedure
Substance P (S6883, Sigma-Aldrich Co.) was injected bilaterally in 10 ng (7.42 pmol) or 100 ng (74.2 pmol) doses. Substance P was dissolved in 0.15 M sterile saline solution containing 0.01 M Na-acetate and 0.01 M phosphate-buffered saline (PBS, pH 7.4). Control animals received this solution as a vehicle (Veh1) in experiment 1. The NK1R antagonist WIN51708 (W103, Sigma-Aldrich Co.) or the NK3R antagonist SR142801 (SML0798, Sigma-Aldrich Co.) were applied directly into the GP in a near equimolar dose to SP, that is, in 5 ng (11.37 or 8.24 pmol, respectively). The NK1R and NK3R antagonists were diluted in 0.15 M saline solution containing 0.3% dimethyl-sulfoxide and 0.01 M PBS, and this vehicle solution (Veh2) was used for control injections in the experiments with the antagonists (ie, in experiments 2 and 3). In this report, all the doses mentioned are meant to be the dose per side values. Eppendorf tubes containing solutions were kept at + 4 °C before application.
Drugs or vehicles were microinjected through a 30-gauge stainless steel injection tube extending 1 mm below the tips of the implanted guide cannulas. The injection cannulas were attached to 10 μL Hamilton microsyringes (Hamilton Co.) via polyethylene (PE-10) tubing. All injections were delivered bilaterally at a steady speed by a syringe pump (Cole Parmer, IITC, Life Sci. Instruments) in the volume of 0.4 μL/side, over a 60-second interval. After the injection, the cannulas were left in place for an additional 60 seconds to allow the diffusion of solutions into the surrounding tissue. During the injections, rats were hand-restrained.
MWM Test
After the postoperative recovery period, behavioral testing was carried out in the MWM paradigm. As it was described in our previous papers,46-49 the apparatus was a circular pool, 150 cm in diameter with a 60 cm high wall. The pool was filled with tap water (temperature: 23 ± 1 °C) and was virtually divided into 4 quadrants. One of these quadrants (ie, the target quadrant) was chosen to place a square (10 cm × 10 cm) Plexiglas platform in it. The location of the platform was constant throughout the experiment, except for the habituation trial when the animals swam without the presence of the platform. The surface of the water was kept 2 cm above the platform, and the water was colored with odorless food dye to make the water opaque and the platform hidden from the animals. External visual cues in the surroundings helped the animals’ spatial orientation; these cues were kept in a constant position throughout the whole experiment. The room was dimly lit by four 40 W bulbs around the pool, providing approximately 60 lux illumination at the water surface.
The MWM procedure was carried out on 3 consecutive days. One day before the training, rats were habituated to the pool by allowing them to perform swimming for 90 seconds without the platform. During the experiments, rats were placed into the water facing the wall of the maze at randomly assigned, but predetermined locations to attenuate egocentric orientation. The task required rats to swim to the hidden platform guided by external spatial cues. After finding the platform, rats were allowed to remain there for 30 seconds. Rats that failed to find the platform in 180 seconds were placed on the platform by the experimenter and allowed to rest there for 30 seconds. During conditioning for spatial learning, rats were placed into the water maze for 2 days, for 2 trials per day, the 2 daily trials were separated by 60-second resting interval (inter-trial interval) on each day. On the first day, the second trial was immediately followed by the bilateral microinjections (see Figure 1) to test the effect of the drugs on the consolidation of the short-term memory trace formed as the result of the acquisition trials. Injections at that time point could not have nonspecific effects, which could have influenced the animals’ performance during the trials (eg, effect on motivation, attention, searching strategy, or motor abilities).
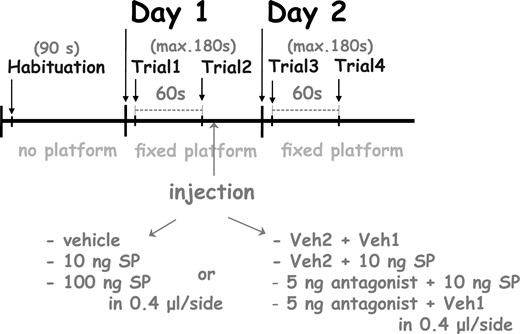
The experimental procedure of the Morris water maze test. For a detailed explanation, see the text.
In experiment 1, the effect of SP on spatial memory consolidation was tested. Rats were microinjected bilaterally with 10 ng or 100 ng SP in the volume of 0.4 μL/side immediately after the second trial on day 1. Doses were selected according to our previous experiments in the GP and the central nucleus of amygdala (CeA).6,10 Control animals received Veh1 in equal volume to that used for SP injections.
In experiment 2, we examined the role of NK1Rs of the GP in the consolidation of spatial memory. The behavioral procedure was the same as in experiment 1, but 4 groups were used: the antagonist-treated group (NK1Ant) received 5 ng (11.37 pmol) WIN51708, and then Veh1. The antagonist-pretreated SP-injected group (NK1Ant + SP) received 5 ng WIN51708 before being injected with 10 ng SP. The SP-treated group (SP) received Veh2 and then 10 ng SP. The control group (Control) received 2 different vehicle injections (Veh2 then Veh1). The vehicle solutions were administered in equal volume to that used for SP and antagonist injections. The antagonist or Veh2 solutions were applied 15 minutes prior to SP or Veh1 injections.
In experiment 3, the role of NK3Rs of the GP in spatial memory consolidation was examined. The behavioral procedure was the same as in experiment 1, but the following groups were used: the antagonist-treated group (NK3Ant) received 5 ng (8.24 pmol) SR142801, and then Veh1. The SP-injected group pretreated with the antagonist (NK3Ant + SP) received 5 ng SR142801 before being injected with 10 ng SP. The SP-treated group (SP) received Veh2 before the 10 ng SP injection. The control group (Control) received Veh2 and then Veh1 in equal volume to that used for SP and antagonist injections. Veh2 or antagonist injections were delivered 15 minutes before Veh1 or SP injection (Animal groups used in the experiments are shown in Table 1).
Experimental groups and treatments; for a detailed explanation, see the text.
Experiment 1 | ||
1. | Control | Veh1a |
2. | SP10 | 10 ng SP (dissolved in Veh1) |
3. | SP100 | 100 ng SP (dissolved in Veh1) |
Experiment 2 | ||
1. | Control | Veh2a pretreatment + Veh1 |
2. | SP | Veh2 pretreatment + 10 ng SP (in Veh1) |
3. | NK1Ant + SP | 5 ng WIN51708 (in Veh2) pretreatment + 10 ng SP (in Veh1) |
4. | NK1Ant | 5 ng WIN51708 (in Veh2) pretreatment + Veh1 |
Experiment 3 | ||
1. | Control | Veh2 pretreatment + Veh1 |
2. | SP | Veh2 pretreatment + 10 ng SP (in Veh1) |
3. | NK3Ant + SP | 5 ng SR142801 (in Veh2) pretreatment + 10 ng SP (in Veh1) |
4. | NK3Ant | 5 ng SR142801 (in Veh2) pretreatment + Veh1 |
Experiment 1 | ||
1. | Control | Veh1a |
2. | SP10 | 10 ng SP (dissolved in Veh1) |
3. | SP100 | 100 ng SP (dissolved in Veh1) |
Experiment 2 | ||
1. | Control | Veh2a pretreatment + Veh1 |
2. | SP | Veh2 pretreatment + 10 ng SP (in Veh1) |
3. | NK1Ant + SP | 5 ng WIN51708 (in Veh2) pretreatment + 10 ng SP (in Veh1) |
4. | NK1Ant | 5 ng WIN51708 (in Veh2) pretreatment + Veh1 |
Experiment 3 | ||
1. | Control | Veh2 pretreatment + Veh1 |
2. | SP | Veh2 pretreatment + 10 ng SP (in Veh1) |
3. | NK3Ant + SP | 5 ng SR142801 (in Veh2) pretreatment + 10 ng SP (in Veh1) |
4. | NK3Ant | 5 ng SR142801 (in Veh2) pretreatment + Veh1 |
NK1Ant: NK1-receptor antagonist.
NK3Ant: NK3-receptor antagonist.
aComposition of Veh1 and Veh2 is described in “Drugs and Injection Procedure.”
Abbreviations: NK1, neurokinin-1; NK3, neurokinin-3; SP, substance P; Veh1 and Veh2, vehicle solutions.
Experimental groups and treatments; for a detailed explanation, see the text.
Experiment 1 | ||
1. | Control | Veh1a |
2. | SP10 | 10 ng SP (dissolved in Veh1) |
3. | SP100 | 100 ng SP (dissolved in Veh1) |
Experiment 2 | ||
1. | Control | Veh2a pretreatment + Veh1 |
2. | SP | Veh2 pretreatment + 10 ng SP (in Veh1) |
3. | NK1Ant + SP | 5 ng WIN51708 (in Veh2) pretreatment + 10 ng SP (in Veh1) |
4. | NK1Ant | 5 ng WIN51708 (in Veh2) pretreatment + Veh1 |
Experiment 3 | ||
1. | Control | Veh2 pretreatment + Veh1 |
2. | SP | Veh2 pretreatment + 10 ng SP (in Veh1) |
3. | NK3Ant + SP | 5 ng SR142801 (in Veh2) pretreatment + 10 ng SP (in Veh1) |
4. | NK3Ant | 5 ng SR142801 (in Veh2) pretreatment + Veh1 |
Experiment 1 | ||
1. | Control | Veh1a |
2. | SP10 | 10 ng SP (dissolved in Veh1) |
3. | SP100 | 100 ng SP (dissolved in Veh1) |
Experiment 2 | ||
1. | Control | Veh2a pretreatment + Veh1 |
2. | SP | Veh2 pretreatment + 10 ng SP (in Veh1) |
3. | NK1Ant + SP | 5 ng WIN51708 (in Veh2) pretreatment + 10 ng SP (in Veh1) |
4. | NK1Ant | 5 ng WIN51708 (in Veh2) pretreatment + Veh1 |
Experiment 3 | ||
1. | Control | Veh2 pretreatment + Veh1 |
2. | SP | Veh2 pretreatment + 10 ng SP (in Veh1) |
3. | NK3Ant + SP | 5 ng SR142801 (in Veh2) pretreatment + 10 ng SP (in Veh1) |
4. | NK3Ant | 5 ng SR142801 (in Veh2) pretreatment + Veh1 |
NK1Ant: NK1-receptor antagonist.
NK3Ant: NK3-receptor antagonist.
aComposition of Veh1 and Veh2 is described in “Drugs and Injection Procedure.”
Abbreviations: NK1, neurokinin-1; NK3, neurokinin-3; SP, substance P; Veh1 and Veh2, vehicle solutions.
The behavior of the animals was recorded by a video camera and registered by a computer program (EthoVision; Noldus Information Technology). The latency to find the safe platform (ie, escape latency) was measured in each trial, and the average latency of the 2 trials on one day (ie, on day 1 or day 2, respectively) was calculated. During each trial, the distance moved and the mean velocities of the animals were also automatically measured.
Histology
At the end of the experiments, rats received an overdose of the ketamine-diazepam mixture and were transcardially perfused with isotonic saline followed by a 10% formalin solution. After 1 week of the post-fixation period, brains were frozen, cut into 40 μm serial sections, and stained with Cresyl-violet. Injection sites were reconstructed according to the stereotaxic atlas of the rat brain.45 Only data from rats with correctly placed cannulas were analyzed.
Statistical Analysis
Data distribution was examined with the Shapiro-Wilk test of normality. Because the distribution of escape latency data was not normal, nonparametric tests were used for statistical analysis. The escape latencies of the different groups on day 1 and day 2 were compared by means of Kruskal-Wallis analysis of variance (ANOVA) for independent groups, followed by the Wilcoxon Signed Rank test for within-group differences and the Mann-Whitney U test for between-group differences. Escape latencies on day 1 and day 2 are represented as median ± upper and lower interquartile ranges as it is the proper form of data presentation for nonparametric analyses. The distribution of swimming velocity data was normal, therefore, the parametric mixed ANOVA was used for the statistical analysis, followed by Bonferroni post hoc test. Swimming velocities during habituation and the average swimming velocities of the two trials on day 1 and day 2 are expressed as mean ± S.E.M. Statistical analyses were conducted using SPSS 20.0 for Windows, and P-values less than .05 were considered significant.
Results
Histology
Histological examination showed that in 110 cases of 124 animals, the target place of the cannulas was precisely and symmetrically tipped to the target area in the GP. The tracks of cannulas and tip positions were determined based on the existence of debris and moderate glial proliferation. A schematic illustration of correct cannula placements is shown in Figure 2A. Considering 14 rats, the reconstructed cannula placement was not correctly positioned in the target area, so these subjects were excluded from subsequent analysis; however, these few data from incorrect placements are not enough to draw far-reaching conclusions. A schematic illustration of incorrect cannula placements is shown in Figure 2B.
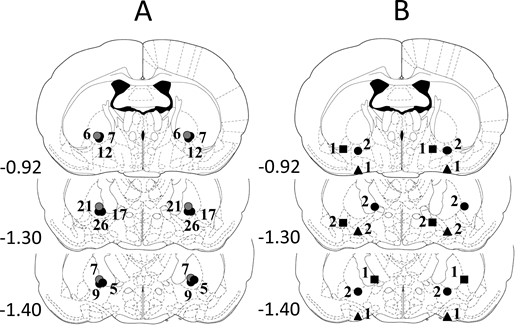
Illustration of reconstructed injection sites from the experiments. Correct bilateral injection placements are indicated as closed circles on panel A (n = 110). Incorrect injection placements are indicated on panel B (n = 14). Brain structure diagrams of coronal sections are adapted from the stereotaxic atlas of Paxinos and Watson,45 the numbers refer to the anterior-posterior distance from Bregma in mm. Identical symbols on panel B indicate coherent injection sites of bilateral injections. The numbers above symbols on panels A and B indicate the numbers of animals.
MWM Test
Experiment 1
Statistical evaluation of experiment 1 indicated that intra-pallidal microinjection of SP improves spatial memory consolidation in the MWM (Figure 3). All groups showed learning in the paradigm, the escape latencies on day 2 significantly differed from those on day 1 in all 3 groups (Wilcoxon Signed Rank test, SP10: P = .003, SP100: P = .010, Control: P = .028). Group medians did not differ on day 1 by Kruskal-Wallis ANOVA, while a significant difference was found on day 2 (P = .037). The low dose of SP (SP10, n = 11) facilitated the consolidation of memory, that is, escape latency was significantly lower in the SP10 group in comparison with that of the Control group (n = 12; Mann-Whitney U test, P = .032) and the high dose SP-treated group (SP100, n = 11; Mann-Whitney U test, P = .040). The facilitatory effect of SP was dose-dependent, as the higher dose of SP did not enhance performance over Controls; their escape latency did not differ (Mann-Whitney U test, P = .288).
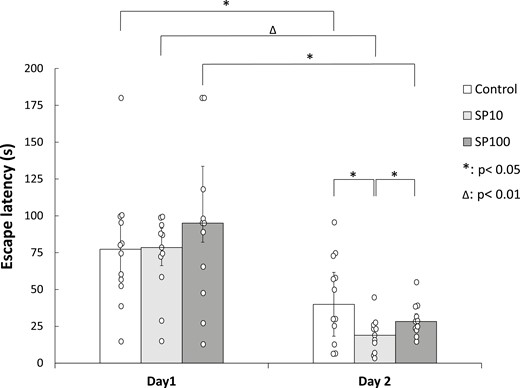
Spatial memory consolidation in the Morris water maze test after post-training bilateral SP microinjections into the GP. Columns represent medians ± interquartile ranges of escape latencies on day 1 and day 2 of the water maze test, respectively. Control: rats microinjected with Veh1 (n = 12). SP10: animals microinjected with 10 ng SP (n = 11). SP100: animals microinjected with 100 ng SP (n = 11). *: P < .05, Δ: P < .01. For more explanation, see the text. GP, globus pallidus; SP, substance P
Experiment 2
In the second experiment, we examined the role of NK1Rs in the mediation of the effect of SP on spatial memory consolidation (Figure 4). Tests were made only with 10 ng SP because this dose was effective in the first experiment. Within-group comparisons by the Wilcoxon Signed Rank test showed a significant learning effect in the case of SP-treated animals (SP, n = 9), as the escape latency decreased significantly from day 1 to day 2 (P = .008). In rats pretreated with NK1R antagonist before SP injection (NK1Ant + SP, n = 9), a similar learning effect was found (P = .008). In the case of animals treated with the antagonist alone (NK1Ant, n = 9) and in the case of Control rats (n = 8), there was only a tendency for escape latency decrease on day 2 compared to day 1 (P = .066 or.050, respectively). Likewise in experiment 1, a significant difference among group medians was found only on day 2 by Kruskal-Wallis ANOVA (P < .05). Comparisons of group medians by Mann-Whitney U test showed that SP treatment could enhance performance over Controls, that is, escape latency was reduced compared to that of the Control group (P = .036). Administration of the NK1R antagonist WIN51708 prior to the intra-pallidal injection of SP could not block the memory consolidation-improving effect of SP. Similarly to the SP-treated group, the escape latency of the NK1Ant + SP group was significantly lower than that of the Controls (P = .015). Moreover, NK1R antagonist pretreatment showed a tendency to enhance the effect of SP since the escape latency was somewhat lower compared to the SP-injected group; however, it did not reach statistical significance (P = .050). When the NK1 antagonist was administered alone, it showed only a tendency to decrease escape latency compared to Controls (P = .074), but there was no significant difference in escape latencies compared to the SP and the NK1Ant + SP groups (P = .489 and P = .796, respectively).
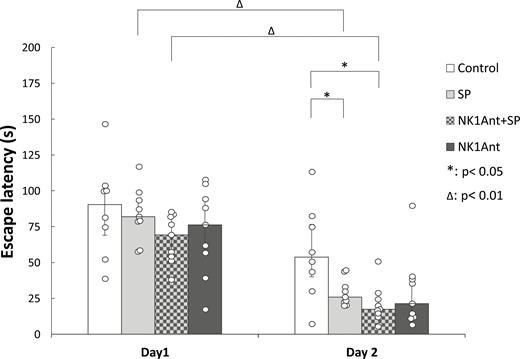
Effects of the NK1 receptor antagonist WIN51708 in the GP on spatial memory consolidation in the Morris water maze paradigm. Ordinate: median ± interquartile ranges of escape latencies on day 1 and day 2, respectively. Control: vehicle-treated rats (n = 8). SP: animals microinjected with 10 ng SP (n = 9). NK1Ant + SP: animals injected with 10 ng SP pretreated with 5 ng of the NK1 receptor antagonist WIN51708 (n = 9). NK1Ant: rats treated with 5 ng NK1 receptor antagonist (n = 9). *: P < .05, Δ: P < .01. For more explanation, see the text. GP, globus pallidus; NK1, neurokinin-1; SP, substance P.
Experiment 3
The role of NK3Rs in the mediation of memory consolidation enhancement by SP injection was examined in the third experiment, and the results are shown in Figure 5. Tests were made only with the effective dose of SP of the first experiment (ie, with 10 ng). In the third experiment, significant learning was found in SP-treated animals, as their escape latency significantly decreased from day 1 to day 2 (SP, n = 10; Wilcoxon Signed Rank test, P = .005). A similar learning effect was found also in the SR142801-treated group (NK3Ant, n = 10; Wilcoxon Signed Rank test, P = .047) and in animals that were pretreated with the NK3R antagonist prior to SP injection (NK3Ant + SP, n = 11; Wilcoxon Signed Rank test, P = .041). In the case of Controls (n = 10), there was only a tendency for escape latency decrease from day 1 to day 2 (Wilcoxon Signed Rank test, P = .093). Kruskal-Wallis ANOVA showed a significant difference among group medians on day 2 (P = .044) but not on day 1. SP treatment improved memory consolidation, as escape latency on day 2 was significantly lower compared to the Control group (Mann-Whitney U test, P = .035). Administration of the NK3R antagonist SR142801 could block the improvement by SP, as the escape latency of the NK3Ant + SP group on day 2 was significantly higher than that of the SP-treated group (Mann-Whitney U test, P = .043). SP improved performance compared to animals treated with the NK3R antagonist alone as well; specifically, SP decreased the escape latency compared to the antagonist-injected group (Mann-Whitney U test, P = .004). Antagonist treatment alone or before SP treatment did not have any effect compared to Controls, escape latencies were not different in the 3 groups.
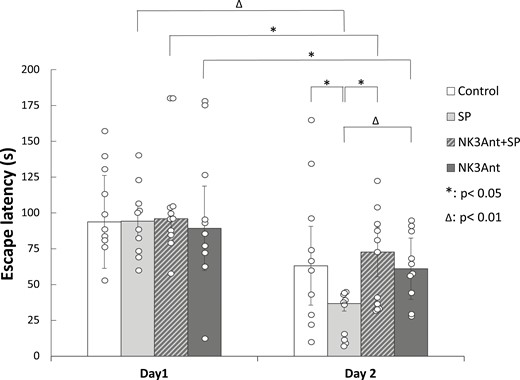
Effects of the NK3 receptor antagonist SR142801 in the GP on spatial memory consolidation in the Morris water maze paradigm. Columns represent medians ± interquartile ranges of escape latencies on day 1 or day 2, respectively. Control: vehicle-treated rats (n = 10). SP: animals microinjected with 10 ng SP (n = 10). NK3Ant + SP: animals injected with 10 ng SP pretreated with 5 ng NK3 receptor antagonist SR142801 (n = 11). NK3Ant: rats microinjected with 5 ng NK3 receptor antagonist (n = 10). *: P < .05, Δ: P < .01. For more explanation, see the text. GP, globus pallidus; NK3, neurokinin-3; SP, substance P.
Swimming velocity data were also analyzed to assess the motor ability of the rats (Table 2). In Experiment 1 the mixed ANOVA analysis showed a significant trial effect (F(2,53) = 10.250; p < 0.001), but the treatment effect (F(2,28) = 2.156; p = 0.134) and the treatment*trial interaction (F(4,53) = 0.200; p = 0.937) were not significant. The Bonferroni test showed a significant difference only in the case of SP100 group, their swimming velocity was lower on day 1 and day 2 compared to that during habituation (p = 0.003 and 0.020, resp.). However, there were no significant differences among any groups during habituation, day 1 or day 2. In Experiment 2 a significant trial effect (F(2,59) = 4.390; p = 0.017) was found with the mixed ANOVA analysis, while the treatment effect (F(3,32) = 1.072; p = 0.375) and the treatment*trial interaction (F(6,58) = 1.557; p = 0.176) were not significant. The difference was significant only in the case of SP treated group, they had higher swimming velocity on day 2 compared to day 1 (Bonferroni test, p = 0.038). Again, there were no significant differences among any groups during habituation, day 1 or day 2. In Experiment 3 the mixed ANOVA analysis revealed no significant trial effect (F(2,82) = 1.518; p = 0.225), treatment effect (F(3,41) = 1.539; p = 0.219) or treatment*trial interaction (F(6,82) = 0.546; p = 0.772). Since no significant differences were found among group means of swimming velocities in any of the experiments, we presume, that the significant differences in the escape latencies were not attributable to alterations in the motor abilities that could be elicited by the different treatments (Table 2).
Habituation . | Day 1 . | Day 2 . | |
---|---|---|---|
Experiment 1 | |||
Control | 38.01±4.61 | 33.73±3.82 | 35.02±3.38 |
SP10 | 36.99±3.48 | 32.15±2.02 | 34.60±3.98 |
SP100 | 37.34±3.35 | 25.77±1.02 | 27.84±1.70 |
Experiment 2 | |||
Control | 32.88±6.47 | 34.53±5.22 | 39.59±6.17 |
SP | 26.71±1.21 | 32.75±3.09 | 41.90±3.74 |
NK1Ant+SP | 28.13±0.79 | 35.23±1.84 | 31.99±1.78 |
NK1Ant | 27.57±1.14 | 33.00±2.05 | 34.79±2.13 |
Experiment 3 | |||
Control | 24.40±1.37 | 24.71±1.06 | 25.63±1.32 |
SP | 25.84±2.78 | 27.83±1.10 | 27.44±1.29 |
NK3Ant+SP | 25.65±0.97 | 25.16±0.65 | 25.97±1.49 |
NK3Ant | 26.97±0.91 | 27.24±1.62 | 27.48±1.42 |
Habituation . | Day 1 . | Day 2 . | |
---|---|---|---|
Experiment 1 | |||
Control | 38.01±4.61 | 33.73±3.82 | 35.02±3.38 |
SP10 | 36.99±3.48 | 32.15±2.02 | 34.60±3.98 |
SP100 | 37.34±3.35 | 25.77±1.02 | 27.84±1.70 |
Experiment 2 | |||
Control | 32.88±6.47 | 34.53±5.22 | 39.59±6.17 |
SP | 26.71±1.21 | 32.75±3.09 | 41.90±3.74 |
NK1Ant+SP | 28.13±0.79 | 35.23±1.84 | 31.99±1.78 |
NK1Ant | 27.57±1.14 | 33.00±2.05 | 34.79±2.13 |
Experiment 3 | |||
Control | 24.40±1.37 | 24.71±1.06 | 25.63±1.32 |
SP | 25.84±2.78 | 27.83±1.10 | 27.44±1.29 |
NK3Ant+SP | 25.65±0.97 | 25.16±0.65 | 25.97±1.49 |
NK3Ant | 26.97±0.91 | 27.24±1.62 | 27.48±1.42 |
Abbreviations: NK1Ant, neurokinin-1 receptor antagonist; NK3Ant, neurokinin-3 receptor antagonist; SP or SP10, 10 ng substance P; SP100, 100 ng substance P.
Habituation . | Day 1 . | Day 2 . | |
---|---|---|---|
Experiment 1 | |||
Control | 38.01±4.61 | 33.73±3.82 | 35.02±3.38 |
SP10 | 36.99±3.48 | 32.15±2.02 | 34.60±3.98 |
SP100 | 37.34±3.35 | 25.77±1.02 | 27.84±1.70 |
Experiment 2 | |||
Control | 32.88±6.47 | 34.53±5.22 | 39.59±6.17 |
SP | 26.71±1.21 | 32.75±3.09 | 41.90±3.74 |
NK1Ant+SP | 28.13±0.79 | 35.23±1.84 | 31.99±1.78 |
NK1Ant | 27.57±1.14 | 33.00±2.05 | 34.79±2.13 |
Experiment 3 | |||
Control | 24.40±1.37 | 24.71±1.06 | 25.63±1.32 |
SP | 25.84±2.78 | 27.83±1.10 | 27.44±1.29 |
NK3Ant+SP | 25.65±0.97 | 25.16±0.65 | 25.97±1.49 |
NK3Ant | 26.97±0.91 | 27.24±1.62 | 27.48±1.42 |
Habituation . | Day 1 . | Day 2 . | |
---|---|---|---|
Experiment 1 | |||
Control | 38.01±4.61 | 33.73±3.82 | 35.02±3.38 |
SP10 | 36.99±3.48 | 32.15±2.02 | 34.60±3.98 |
SP100 | 37.34±3.35 | 25.77±1.02 | 27.84±1.70 |
Experiment 2 | |||
Control | 32.88±6.47 | 34.53±5.22 | 39.59±6.17 |
SP | 26.71±1.21 | 32.75±3.09 | 41.90±3.74 |
NK1Ant+SP | 28.13±0.79 | 35.23±1.84 | 31.99±1.78 |
NK1Ant | 27.57±1.14 | 33.00±2.05 | 34.79±2.13 |
Experiment 3 | |||
Control | 24.40±1.37 | 24.71±1.06 | 25.63±1.32 |
SP | 25.84±2.78 | 27.83±1.10 | 27.44±1.29 |
NK3Ant+SP | 25.65±0.97 | 25.16±0.65 | 25.97±1.49 |
NK3Ant | 26.97±0.91 | 27.24±1.62 | 27.48±1.42 |
Abbreviations: NK1Ant, neurokinin-1 receptor antagonist; NK3Ant, neurokinin-3 receptor antagonist; SP or SP10, 10 ng substance P; SP100, 100 ng substance P.
DISCUSSION
The aims of the present study were to elucidate the role of pallidal SP in spatial learning mechanisms and to determine the type of NK receptors that mediate its actions. The results of our experiments confirm that SP microinjected into the GP improves consolidation of spatial memory in the MWM test, and this effect is mediated through NK3 but not NK1 receptors.
The MWM test is a widely used method to investigate spatial learning, where animals need to find a hidden platform in a pool filled with opaque water, according to allocentric or egocentric navigation.50,51 Since control animals can learn the place of the safe platform after only 4 to 6 trials,12,13,50 it is more appropriate to study the learning-enhancing effect of a substance in a schedule with a limited number of trials and/or days. MWM paradigm with only 2 trials per day can also be found in the literature,52 and previous results of our research group with other neuropeptides were obtained with the same MWM schedule.46-48 Even with this short method, memory consolidation can be examined. In the first trial, animals find the platform randomly, with a relatively long latency; however, during trial 2, the escape latency is shorter due to a short-term memory trace formed after trial 1.49 If this short-term memory trace is strengthened, animals can find the platform with a similarly short latency during the first trial on the next day, while the escape latency of those animals in which this consolidation did not occur will have longer latencies. Likewise in our previous experiment with quinpirole,49 the shorter escape latency on day 2 in the present experiment can be attributed to a memory consolidation-enhancing effect of SP. However, escape latencies on day 2 will decrease in the case of all animals, and a weak learning effect (or a learning tendency) can be seen in all groups.
A number of experiments have implicated SP in learning and memory in different paradigms, in several brain areas.3,8,9 In our previous experiments, SP improved memory consolidation and retention in the vmGP and the CeA in the step-through avoidance test as well.10 Peripheral SP treatment also enhanced place learning of adult and aged rats and improved spatial learning of rats with hippocampal lesions in the MWM test,11-13 therefore, our present results are consistent with the data above. Comparable effects were found after SP microinjection into the motor cortex of rats, SP improved skill acquisition and promoted the encoding of memory after the first injection of SP, while it influenced neither learning in the later phase nor retention.9
Our results are also in good accordance with several data in the literature confirming the role of the GP in learning and memory processes.15,17,18,20 There are some data regarding the effects of pallidal lesions on spatial learning as well, namely, lesions of the rostral GP led to a deficit in the milk maze performance of rats,24 while lesions of the lateral pallidum led to an increase in escape latencies compared to controls during the acquisition and retention of the MWM task.25 Several findings support the crucial role of the basal ganglia in spatial learning, and the different parts of the striatum were shown to be implicated in distinct aspects of it. Specifically, the posterior dorsomedial striatum seems to play a role in allocentric navigation by external cues.21-23 According to the distribution of peptides and projection targets, the vmGP and the dlGP are morphologically and functionally different,19,26,27 the medial tip of the GP mainly receives fibers from the associative dorsomedial striatal regions. Based on the above-cited findings, one might suppose the role of the vmGP in place learning, especially in allocentric navigation, but the exact mechanism needs further investigation.
SP immunoreactivity in the GP was shown to be confined to fibers and terminal processes that are in contact mainly with GABAergic neurons and might originate in the caudate-putamen complex.30,53 The presence of neurokinin B mRNA in striatopallidal projections was demonstrated as well.54 As neurokinin B (NKB) is the most potent natural agonist of NK3Rs, one might assume that in natural conditions NKB and not SP have a memory consolidation-enhancing effect similar to that observed in our experiments. Since SP can activate NK3Rs,55 and, on the other hand, in a newer study, it was found that NKB fibers of the dorsal striatum sparsely innervate the GP cells,56 we suppose that SP and not NKB might stimulate NK3Rs even in natural circumstances. However, volume transmission was shown in the case of SP,57 and we cannot exclude the possibility that, similarly to SP, NKB can exert effects through volume transmission.
NK1Rs are expressed in moderate to high densities in the GP,29,32,33 and NK3Rs were detected as well.34 The presence of NK1Rs and NK3Rs in this structure suggests that SP can exert its effect through either of these receptors. In vivo extracellular recordings demonstrated that the spontaneous firing rate of the GABAergic neurons of the GP is increased by NK1R stimulation.42,43 On the other hand, no information is available in the literature about the role of NK3R activation in the GP at present.
There is a discrepancy regarding the role of NK1Rs in the control of learning and memory processes. In some studies, NK1R activation enhanced learning and memory, e.g., facilitated long term potentiation in the CA1 region of the hippocampus,35 and improved passive avoidance learning and retention in the CeA in our previous experiment,10 while knocking down NK1R activity in the striatal marginal division and hippocampus elicited learning and memory impairment in the Y-maze test.8 On the contrary, in other experiments NK1R activation seems to have the opposite effect, since learning and memory processes were enhanced by NK1R antagonism. Namely, systemic administration of a selective NK1R antagonist facilitated long-term memory formation in inhibitory avoidance and open-field habituation tests or could reinstate episodic-like memory of rats.58,59 These latter data are consistent with our present finding that NK1R antagonism in the GP tends to enhance memory consolidation over SP-treated and control animals. The role of NK3Rs in the regulation of learning and memory processes is also well established. NK3R stimulation enhanced fear memory consolidation in the CeA,38 facilitated the consolidation and expression of episodic-like memory37; furthermore, improved learning in the water maze in aged rats.36 NK3R knockout mice showed impairment in the retention of passive avoidance, and a delay in the acquisition of conditioned avoidance responding, as well as of the MWM escape task.39 Since SP can activate all 3 tachykinin receptive sites and shows poor selectivity,1,31 it may exert its effect on any NK receptor type; however, in most cases, in the mediation of SP effects, the role of NK1Rs was confirmed.6,10,40-43 There is sparse evidence in the literature for the involvement of NK2Rs,60,61 and, to the best of our knowledge, our present results are the first to demonstrate that NK3Rs might mediate the effects of SP.
In our previous experiments, the promnestic effect of SP in the CeA was blocked by the NK1R antagonist WIN51708 pretreatment,10 and the rewarding effect of SP in the GP could be inhibited by the same NK1R blocker.6 On the contrary, in this experiment, the effect of SP was not counteracted by the NK1R antagonist, while NK3R antagonism could inhibit it. It seems, therefore, that the 2 different receptor types contribute to different regulatory processes within the GP. Recently, it was shown by our research group that antagonism of D2-type dopamine receptors in the ventral pallidum exerts opposite effects on the consolidation of spatial memory and reward-related processes.62 Our present results show similar antagonistic effects of NK1R activation in the GP, namely it has a rewarding effect but tends to interfere with spatial memory consolidation. One possible explanation of this discrepancy is that through the activation of the different NK receptors, not the same second messenger pathways are activated in the cells, thus eliciting diverse effects. It was shown that NK1R activation might induce many different second messenger pathways through different G protein types,63 while NK3R was shown to be coupled mainly to the Gq signaling pathway55; however, NK3R might also be internalized into the nucleus, resulting in altered gene transcription.64 Another possibility is that the various cell types of the GP express NK1Rs and NK3Rs in different quantities. Supporting this hypothesis, NK1Rs were detected mainly on cholinergic cells,32 while NK3Rs were found primarily on GABAergic cells of the GP, and their colocalization was very rare.34 In this study, we cannot give the detailed molecular-receptorial mechanisms underlying our results; therefore, further experiments with selective agonists and antagonists of different NK receptors, and comprehensive examination with immunohistochemical and electrophysiological methods are necessary to cast light on the exact pathway through which SP can exert its effects on spatial learning mechanisms in the GP.
In conclusion, our results are the first to demonstrate that SP facilitates spatial memory consolidation in the MWM paradigm when injected into the vmGP. The effects of SP are dose-dependent since only the lower dose improved the consolidation of spatial memory, while the higher dose was ineffective. The effect of SP was not blocked by the prior application of a non-peptide NK1R antagonist, but NK3R antagonism could counteract the facilitating effect of SP. Present data show that SP plays a role in spatial learning processes related to the GP through the activation of NK3Rs; however, the exact mechanism of action remains to be elucidated. Recently, a study by de Souza Silva and coworkers proved a correlation between a single nucleotide polymorphism in the NK3R encoding gene and the performance of elderly patients having cognitive impairments in learning and memory tests, suggesting that NK3Rs might be a target for the treatment of cognitive impairments in the elderly.36 Our data might contribute to a better understanding of the role of the NK3R system in the basal ganglia in cognitive processes, and finding a future treatment target for dementia.
Acknowledgments
The authors would like to express their thanks to Irén Frumen, Erika Kvak, Erzsébet Korona, Erika Szabó, and Pálma Fogasi for their technical contribution to this work.
Author contributions
Erika Kertes (Conceptualization [equal], Data curation [supporting], Formal analysis [supporting], Investigation [supporting], Methodology [lead], Project administration [equal], Resources [supporting], Software [supporting], Validation [supporting], Visualization [lead], Writing—original draft [lead], Writing—review & editing [lead]), László Péczely (Conceptualization [supporting], Data curation [equal], Formal analysis [equal], Methodology [equal], Project administration [supporting], Resources [supporting], Writing—original draft [supporting], Writing—review & editing [supporting]), Tamás Ollmann (Data curation [equal], Methodology [supporting], Software [equal], Validation [supporting], Writing—review & editing [supporting]), Kristóf László (Conceptualization [supporting], Data curation [supporting], Funding acquisition [lead], Methodology [equal], Project administration [equal], Resources [supporting], Writing—review & editing [supporting]), Beáta Berta (Data curation [supporting], Formal analysis [supporting], Funding acquisition [supporting], Investigation [equal], Resources [equal], Writing—review & editing [supporting]), Veronika Kállai (Data curation [supporting], Formal analysis [supporting], Investigation [equal], Software [supporting], Visualization [supporting], Writing—review & editing [supporting]), Olga Zagorácz (Methodology [supporting], Validation [equal], Visualization [supporting], Writing—original draft [supporting], Writing—review & editing [supporting]), Anita Kovács (Formal analysis [equal], Investigation [supporting], Resources [equal], Validation [supporting], Writing—review & editing [supporting]), Ádám Szabó (Investigation [supporting], Software [equal], Validation [equal], Visualization [supporting], Writing—review & editing [supporting]), Zoltán Karádi (Conceptualization [supporting], Supervision [equal], Writing—review & editing [supporting]), and László Lénárd (Conceptualization [equal], Supervision [equal], Writing—review & editing [supporting])
Funding
Open-access funding was provided by University of Pécs, Medical School, Pécs, Hungary. This work was supported by University of Pécs, Medical School, Pécs, Hungary (PTE ÁOK KA-2020-06), and the New National Excellence Program of the Ministry for Innovation and Technology (ÚNKP-21-5-PTE-1333).
Conflicts of interest
The authors declare no conflict of interest.
Data availability
Datasets generated during the experiments are available from the corresponding author upon reasonable request.