-
PDF
- Split View
-
Views
-
Cite
Cite
Meng-Chang Ko, Yu-Hui Hung, Pei-Yin Ho, Yi-Ling Yang, Kwok-Tung Lu, Neonatal glucocorticoid treatment increased depression-like behaviour in adult rats, International Journal of Neuropsychopharmacology, Volume 17, Issue 12, December 2014, Pages 1995–2004, https://doi.org/10.1017/S1461145714000868
- Share Icon Share
Abstract
Synthetic glucocorticoid dexamethasone (DEX) is frequently used as a therapeutic agent to lessen the morbidity of chronic lung disease in premature infants. Previous studies suggested that neonatal DEX treatment altered brain development and cognitive function. It has been recognized that the amygdala is involved in emotional processes and also a critical site of neuronal plasticity for fear conditioning. Little is known about the possible long-term adverse effect of neonatal DEX treatment on amygdala function. The present study was aimed to evaluate the possible effect of neonatal DEX treatment on the synaptic function of amygdala in adult rats. Newborn Wistar rats were subjected to subcutaneous tapering-dose injections of DEX (0.5, 0.3 and 0.1 mg/kg) from post-natal day one to three, PN1–PN3. Animals were then subjected to a forced swimming test (FST) and electrophysiological recording aged eight weeks. The results of the FST showed neonatal DEX treatment increased depression-like behaviour in adulthood. After acute stress evoking, the percentage of time spent free floating is significantly increased in the DEX treated group compared with the control animals. Furthermore, neonatal DEX treatment elevated long-term potentiation (LTP) response and the phosphorylation level of MAPK in the lateral nucleus of amygdala (LA). Intracerebroventricular infusion of the MAPK inhibitor, PD98059, showed significant rescue effects including reduced depression-like behaviour and restoration of LTP to within normal range. In conclusion, our results suggested that MAPK signalling cascade in the LA plays an important role in the adverse effect of neonatal DEX treatment on amygdala function, which may result in adverse consequences in adult age, such as the enhancement of susceptibility for a depressive disorder in later life.
Introduction
The experience in early life may have a major impact on later life (Lehmann et al., 2000; Kosten et al., 2007). Epidemiological studies on post-traumatic stress disorder (PTSD) suggest that early adversity, such as physical abuse or psychological maltreatment, is a major risk factor for developing PTSD in later periods of life. Psychoanalysts and child psychiatrists have reported that childhood abuse, the mother–child relationship and the fostering environment in early childhood strongly affects mental development and influences the prevalence rate of various psychiatric disorders including PTSD (Bifulco et al., 1991; McCauley et al., 1997; Young et al., 1997; Widom, 1999; Imanaka et al., 2006). Meaney and co-workers demonstrated that low maternal care resulted in decreased negative feedback on the hyperfunctioning of the hypothalamo-pituitary-adrenal (HPA) axis in response to stress through the increased methylation of the promoter of hippocampus glucocorticoid receptors (Meaney et al., 1996; Weaver et al., 2004; McGowan et al., 2009). It has been also reported that maternal separation or lack of maternal care results in increased corticotrophin releasing factor (CRF) mRNA expression (Aisa et al., 2009; Maken et al., 2010). Collectively, these results imply neonatal stress may modify the HPA axis and has long-lasting effects on stress responses in adolescence and adulthood (Aisa et al., 2007, 2009; Marais et al., 2008; McGowan et al., 2009).
In addition to neonatal adversity, some neonatal medications such as dexamethasone and L-carnitine are suspected to produce subtle changes in brain maturation, the impact of which may become apparent only in the later periods of life (Liu and van der Leij, 2011; Ter Wolbeek et al., 2013). Dexamethasone (DEX) is a synthetic glucocorticoid and is frequently used to lessen the progression of chronic lung disease in extremely low birth weight (ELBW) infants (LeFlore et al., 2002; Jefferies, 2012; Cheong et al., 2014). Even though clinical trials failed to consistently demonstrate improvement in mortality or length of hospitalization, exposures of up to 42 days were commonly used in many hospitals (For review Demauro, Dysart and Kirpalani, 2014). The time of DEX treatment for premature infants corresponds to the viable perinatal period from 24 to 40 weeks after conception. During this critical period for development, an infant's central nervous system undergoes profound structural and functional transformations, making it particularly vulnerable to external adverse stimulus. Common side effects of dexamethasone treatment in ELBW include hypertension (Fauser et al., 1993), bowel perforation, infection (Gordon, Young and Marshall, 2001), ventricular hypertrophy (Gill, Warner and Bull, 1996), catabolic changes (Verhaeghe et al., 2007) and the alteration of the limbic HPA axis (Aisa et al., 2007, 2009; Marais et al., 2008; McGowan et al., 2009). In animal studies, neonatal dexamethasone treatment in the rat leads to kidney damage in adulthood (de Vries et al., 2010). It also increases susceptibility to experimental autoimmune disease (Bakker et al., 2000) and the risk of pulmonary hypertension in adult rats (le Cras et al., 2000).
Previous studies also linked dexamethasone treatment to long-term neurological effects, including an increased incidence of cerebral palsy and decreased cerebral volume (Lee et al., 2008; Wilson-Costello et al., 2009). In addition, results also suggest neonatal dexamethasone treatment impaired brain development and cognitive function (Barrington, 2001; Neal et al., 2004). In addition, it has been proven that neonatal DEX treatment altered hippocampal synaptic plasticity and associative memory in rats (Kamphuis et al., 2003; Lin et al., 2006). Flagel and colleagues showed that DEX treated animals are less active in light and dark environments, while administering a blunted serum corticosterone response to a novel stress (Flagel et al., 2002; Claessens et al., 2011). These data suggest that neonatal DEX treatment permanently alters central nervous system function, particularly within the neuroendocrine stress axis. This may lead to an increase in risk of maladaptive responses to the environment.
We hypothesized that neonatal DEX treatment during early post-natal life might modify the developmental assembly of the amygdala, thereby providing a potential correlation for early experience-dependent effects on later responsiveness to emotionally evocative stimuli and, more generally, might enhance susceptibility for having depression disorder in later life. The present study was aimed to test this hypothesis by using behavioural and electrophysiological approaches.
Material and method
Subjects
Pregnant female Wister rats were obtained from the BioLASCO, Taiwan, Co., Ltd. Only pups born on day 22 or 23 of gestation were used. Within six to eight hours after delivery, female pups were removed from their nests and four to six male pups were placed back with each dam. Pups were weaned at twenty-one days old and remain group housed with littermates until experimentation at five to eight weeks old. They were housed in a temperature (24°C) controlled animal colony, with continuous access to food and water. Animals were maintained on a 12:12 light-dark cycle with lights on at 07:00. All behavioural procedures were taken place during the animal light cycle. All procedures were conducted in accordance with the National institutes of Health Guide for Care and Use of Laboratory Animals and the guidelines set forth by the local institutional animal care and use committee (IACUC) at the National Taiwan Normal University. All efforts were made to minimize animals suffering and to use the number of animals necessary to produce reliable data.
Dexamethasone administration protocol
During post-natal days one to three, pups were randomly assigned to two groups including the saline treated group (control group) and the dexamethasone treated group (DEX group). They were removed from their home cage and separated from their mother for drug treatment and body weight measurement. All processes were carried out between 10:00 and 14:00 and completed within 10 min. DEX group pups received a daily s.c. injection of DEX from P1 to P3. DEX was given in tapering doses of 0.5 mg/kg on P1, 0.3 mg/kg on P2, and 0.1 mg/kg on P3. Pups in the control group received equivalent volumes of subcutaneous injections of saline.
Locomotor activity
To determine the possible effect of neonatal DEX treatment on rats spontaneous activities, locomotor activity took place previous to other behaviour procedures. Rats were placed in the centre of the Plexiglas testing chamber facing away from the experimenter. The Plexiglas testing chamber was 42 cm wide and 36 cm in height. Horizontal distance was recorded by EthoVision video tracking system (EthoVision Noldus, NED). Tests lasting for 15 min were set up in three 5 min blocks (Zhang et al., 2001).
Rota-rod test
The animals’ motor coordination was evaluated by rota-rod treadmills. Rats were placed on the rota-rod for 1 min training with a constant speed rotation (4 rpm). In testing sessions, rats were placed on the rota-rod with an accelerated speed from 4 to 40 rpm. The retention time of each rat on the rota-rod was measured by timer, which stop recording when the rat fell down to the plate below. The latency to fall was recorded to a maximum of 600 s (Song et al., 2006).
Forced swimming tests
The forced swimming test method described by Porsolt et al. (1978) was carried out with some modification. The behavioural cylinder was 40 cm high and 18 cm in diameter. Before each session, the cylinder was filled with water to a height of 30 cm, so that rats could not touch the bottom with their paws or tail. The temperature of the water was adjusted to 25 ± 1°C. Rats were forced to swim for 15 min on the first experimental day. At the end of test session, rats were placed into a Plexiglas box under a 60 W bulb for 30 min to dry and then put back into their home cages. The next experimental session was followed 24 h later by a 5 min test session, and total immobility time was measured during this period. In the acute stress-evoking protocol, animals were placed on the elevated platform, 12 cm square and 95 cm from the ground prior to the test session. After the test session, the rats were decapitated and the brains were quickly removed from the skull. The amygdala was dissected out from the brain slices and subjected for Western blotting analysis.
Stereotaxic surgery and drug microinjection
The rats were anesthetized with sodium pentobarbital (50 mg/kg) i.p. and positioned in a stereotaxic apparatus (David Kopf instruments, Tujunga, CA, USA). Stainless steel guide cannulas (25 gauge, 12 mm) were implanted in the bilateral ventricle. The stereotaxic coordinates for guide cannula placement in the bilateral ventricle were: anterior–posterior (AP)=−0.4 mm, dorsal–ventral (DV)=−4.2 mm, and lateral–medial (LM)=±1.5 mm from bregma. The guide cannulas were anchored to the skull with three steel screws and dental cement. A stainless wire was inserted into each cannula to protect it from clogging. The animals were given seven days for recovery prior to behavioural procedures.
The MEK inhibitor PD98059 was applied into the bilateral ventricles through a micro-syringe pump. The sub-threshold dosage of PD98059 was 1 μg in 5 μl, and the process rate was 0.5 μl/min (Murray et al., 1998; Schafe et al., 1999). The injection needles were left in the guide cannulas for 1 min following infusion to allow the drug to diffuse from the needle tip. Rats were placed back into their home cages after injection. The forced swimming test took place 30 min after drug infusion.
Brain slice extracellular recording
In a separate in vitro experiment, animals were decapitated and the brains were quickly removed from the skull. Coronal slices were cut 400 μm thick using the vibrotome. (MA752 Campden Instruments Ltd., UK) The appropriate slices were placed in a beaker of artificial cerebrospinal fluid (ACSF). The ACSF was bubbled continuously with 95%O2/5%CO2 to maintain the pH at 7.3–7.5. The ACSF consisted of 117 mm NaCl, 4.7 mm, KCl 2.5 mm, CaCl2, 1.2 mm MgCl2, 25 mm NaHCO3, 2 mm NaH2PO4 and 11 mm glucose. The slices were kept at room temperature for at least 1 h for stabilization before recording. A single slice was then transferred to the recording chamber where it was held submerged between two nylon nets and maintained at 32 ± l °C. The chamber consisted of a circular well of low volume (1–1.5 ml) and was perfused constantly at the rate of 3–4 ml/min. A bipolar stimulating electrode (SNE-2OOX, Kopf Instrument, USA) was used. Field excitatory post-synaptic potential (fEPSP) was recorded extra-cellularly using glass micro-electrodes fined with 3 m NaCl (3–8 mΩ). The stimulus electrode and recording electrode were placed in the internal capsule and the lateral nucleus of amygdala respectively. The evoked fEPSP signals were recorded using an Axoclamp-2B amplifier (Axon Instruments, USA). The responses were elicited by low square-wave pulses, delivered at 20 s interval and filtered at 1 kHz and digitized at 5 kHz (Digidata 1322A; Axon Instruments, USA). The stimulation voltage was adjusted individually for each experiment to produce fEPSP, which were 30–40% of the maximal response that could be evoked. The strength of synaptic transmission was quantified by measuring the initial slope of the fEPSP and analysed using pCLAMP software (Version 10.2; Axon Instruments, USA). The LTP was induced by high-frequency stimulation (HFS) at two-fold of test pulse intensity. (Two one-second trains of 100 Hz stimuli separated by an inter train interval of 20 s).
Western blot analysis
At the end of the behavioural session, animals were decapitated and the brains were quickly removed from the skull. The tissue of the amygdala region was dissected and homogenized using a T-PER tissue protein extraction reagent kit (Pierce Biotechnology Inc., USA). The samples were grinded on low power by a blender until fully homogenized. Samples were spun down at 13 200 g at 4 °C for 10 min. Total protein concentration was determined by Bio-Rad Bradford Protein Assay Kit (Bio-Rad, Hercules, USA). Each sample in equal 25 μg amounts of protein was subjected to sodium dodecyl sulphate polyacrylamide electrophoresis gel (SDS-PAGE). The protein was separated on the PVDF membrane (Merck Millipore, Germany). Blotting was blocked in PBS solution containing 5% non-fat milk and 0.05% Tween 20 for 1 h at room temperature. Two primary antibodies were used to detect the phosphorylated MAP kinase ERK1/2 (1:1000; Cell Signalling Tech. USA) and GAPDH (1:1000; Gene Tax, Inc. USA). The PVDP membranes were first incubated in a primary antibody PBS solution overnight at 4°C. They were then probed with HRP-conjugated secondary antibodies for 1 h at room temperature. The signals were detected using enhanced chemiluminescence. (Bioman Scientific Co. Ltd., Taiwan) and analysed by LAS3000 digital imaging system (Fujifilm, Japan). The phosphorylation degree of ERK1/2 was determined using the relative amount of the phosphorylation-independent antibody.
Statistical analysis
Data are expressed as means±s.e.m. Body weight and per cent of fear potentiation scores (forced swimming test) were analysed by one-way analysis of variance (ANOVA). Fisher's post-hoc tests were performed to determine the source of detected significance in the ANOVA. LTP and PPF experiments statistical analysis were performed using the Mann–Whitney U test. Protein phosphatase activity data in Western blot were analysed using an unpaired Student's t-test. Probability levels of less than 0.05 (p < 0.05) were considered to be significant. All statistical analysis was performed using SPSS version 12.0 (SPSS, USA).
Results
Neonatal DEX treatment effects on somatic growth of pups
To determine whether neonatal DEX treatment influences somatic growth in later life, we compared the body weights of rats obtained from DEX- and SAL-treated groups at various post-natal ages including post-natal day-1, 2, 3, 28, 42 and 56. As shown in Fig. 1, neonatal DEX treatment significantly reduced body weight gain on P3 (p < 0.001), P28 (p < 0.001), and P42 (p < 0.05 compared with control animals) but by P56, there was no body weight difference between groups (p = 0.331 compared with control) (Fig. 1). These results suggested that neonatal DEX treatment affects somatic growth of pups in a temporary manner.
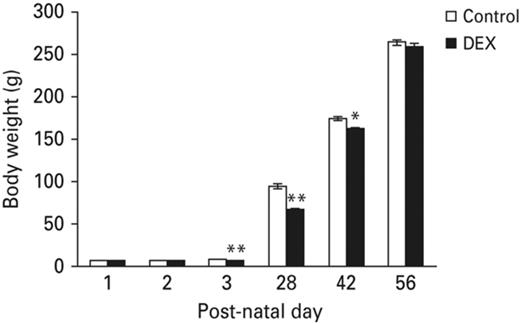
Neonatal DEX treatment effects on somatic growth. Neonatal DEX treatment resulted in decreasing body weight in the P3, P28 and P42 animals compared with the control animals, but by P56 there was no significant weight difference between groups. Each vertical bar represents the Mean±s.e.m. for each group (**p < 0.01, *p < 0.05).
Neonatal administration of DEX increased depression-like behaviour in rats measured by forced swim test
Previous studies have shown neonatal stress treatment impaired some amygdala-dependent emotional learning and memory tasks. We hypothesized that neonatal administration of dexamethasone mimicked neonatal stress events and increased depression-like behaviours. We tested this hypothesis by using the forced swim test (FST) paradigm. Forty animals were assigned to four different groups including the saline treated (SAL), saline+acute stress (SAL+AS) and dexamethasone treated (DEX) and dexamethasone+acute stress (DEX+AS) groups. Pups in the DEX and DEX+AS group received a daily i.p. injection of DEX between PN1 to PN3. DEX was given in tapering doses of 0.5 mg/kg on PN1, 0.3 mg/kg on PN2 and 0.1 mg/kg on PN3. Animals in the SAL group received equivalent volumes of i.p. injections of sterile saline as the pups in the DEX group. Animals were then subjected to FST tests at the age of eight weeks. Results showed that neonatal administration of dexamethasone enhanced depression-like behaviours after acute stress. In the FST test, one-way ANOVA revealed a significant effect of DEX+AS treatment on the percentage of immobility (% immobility) (Fig. 2a). No significant difference in percentage of immobility was observed in the DEX group compared to the SAL group.
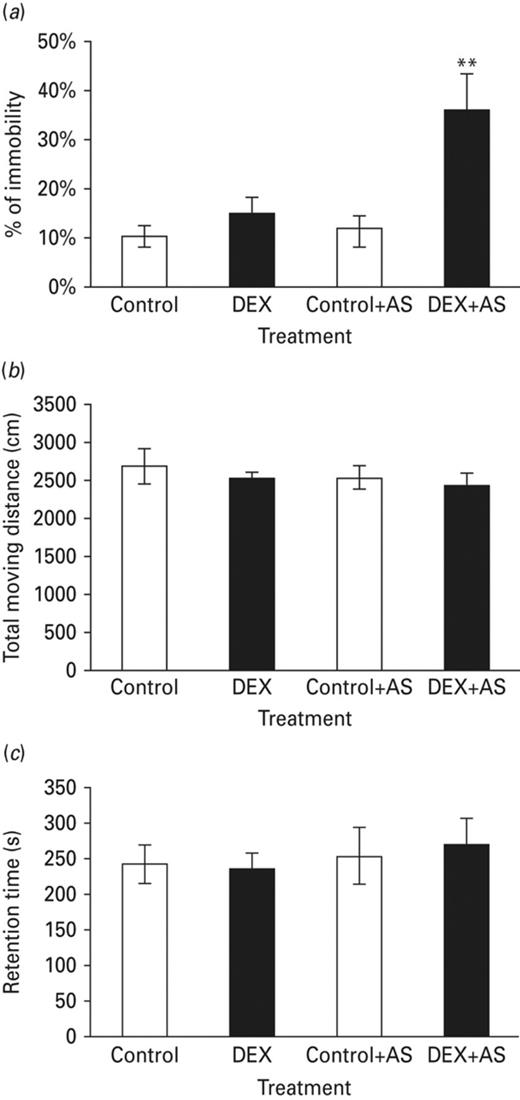
Neonatal DEX-treated animals significantly increased depression-like behaviour after exposure to an acute stress (flat-top exposure). (a) Without an acute stress (AS), no difference on the percentage of immobility among the DEX-treated and control groups. After flat top exposure, significant increase of immobility on the DEX-treated animals compared with control group. (b) Compared with the control group, neonatal DEX treatment results showed no significant different in locomotor activity between groups. (c) There was no significant different in retention on rota-rod task among each groups. Each vertical bar represents the Mean±sem for each group (**p < 0.01).
It is possible that neonatal DEX treatment may have a long-term adverse effect on motor function, resulting in misinterpretation of its increasing effects on depression-like behaviour. To test this possibility, all rats from experiment-1 were subjected to locomotor activity and rota-rod task. Results showed neither the total moving distance (Fig. 2b) nor retention time (Fig. 2c) were significantly different when compared with the control group. Thus, the increase of depression-like behaviour observed in the neonatal DEX treated animals appeared to result from a central effect rather than from a more permanent, perhaps toxic, action of DEX on motor function.
Neonatal DEX treatment enhanced the acute stress induced MAPK phosphorylation in amygdala
Previous studies have shown that enhanced MAPK phosphorylation level in amygdala is correlated to an increase of depression-like behaviour (Lin et al., 2006). We used Western blot to evaluate the amygdaloid MAPK phosphorylation level, testing whether the activation of the amygdaloid MAPK signal cascade is responsible for increasing depression-like behaviour in neonatal DEX-treated animals after acute stress exposure. Additional neonatal DEX-treated rats were used. Half of them received acute stress: exposure in a platform for 30 min before FST. Animals were killed by decapitation immediately after FST. The amygdala were collected and subjected for Western blot analysis. Without acute stress treatment, no significant difference on the MAPK phosphorylation level had been found between control and neonatal DEX-treated animals (Fig. 3a). With acute stress treatment, compared with the corresponding control group, MAPK phosphorylation was significantly elevated in amygdala after FST (Fig. 3b). These results raised the possibility that increase in depression-like behaviour in the neonatal DEX-treated group was mediated by the amygdaloid MAPK dependent signalling cascade.
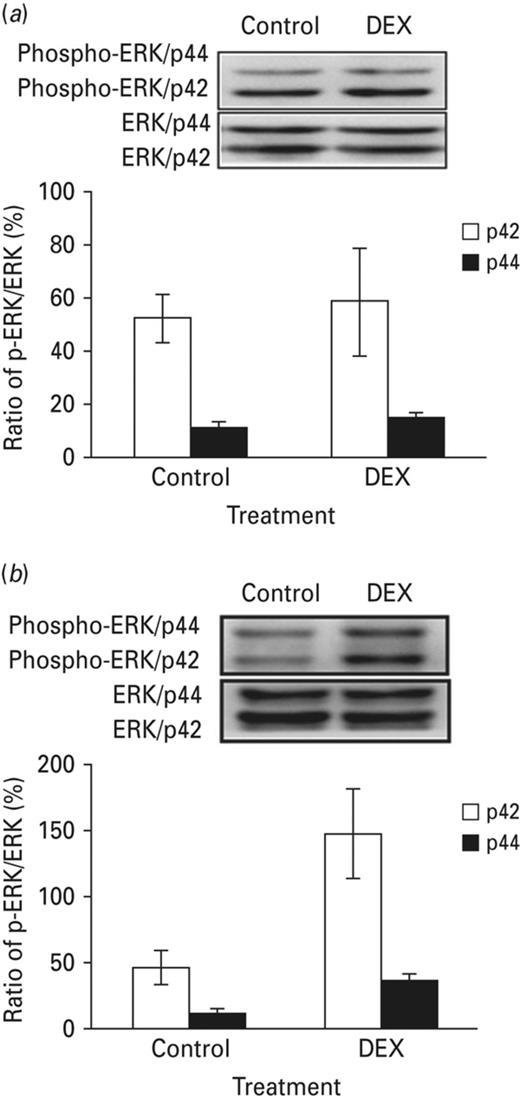
Amygdaloid ERK phosphorylation elevated after forced swim test on the DEX-treated combined acute stress animals. The figure depicts the differences in phosphorylation levels of amygdaloid MAPK across groups; the phosphorylation levels of were standardised to the total MAPK expression levels. (a) Without acute stress, no significant difference on the amygdaloid ERK phosphorylation level had been detected between DEX-treated and control group. (b) Compared with the corresponding control group, amygdaloid ERK phosphorylation level increased in the DEX-treated animals with acute stress. Each vertical bar represents the Mean±s.e.m. for each group.
High frequency induced LTP was increased in the amygdala of neonatal DEX treated animals
Lin et al. (2006) showed that neonatal DEX treatment in particular doses, altered synaptic plasticity in the hippocampus only in adolescence and did not persist into adulthood. We used extracellular recording to test the effects of neonatal administration of dexamethasone on the LTP formation in amygdala. Briefly, animals were subjected to in vitro extracellular recording, field excitatory post-synaptic potentials (fEPSPs) were recorded extracellularly by using glass microelectrodes with 4 m NaCl and placed into neurons of the amygdala (for detailed information please refer to the Materials and Method section). Neonatal application of dexamethasone caused an enhanced effect on LTP formation in the amygdala. The average magnitude of potentiation in the DEX-treated group measured 50 min after tetanus stimulus was 199 ± 11% (n = 6 p < 0.05) compared to the control group (166 ± 7%) (Fig. 4). These results suggested that neonatal DEX treatment has a long-lasting effect on the function of amygdala.
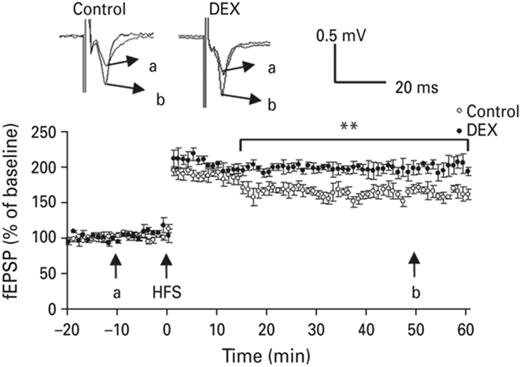
High frequency stimulation induced LTP in amygdala was increased in the neonatal DEX-treated animals compared with the control group. Typical traces were displayed in the upper panel, a & b represent 10 min before induction and 60 min after induction, respectively. Each vertical bar represents the Mean±sem. **p < 0.01 compared with control group.
Intracerebroventricular infusion of MAPK inhibitor PD98059 in a sub-threshold dose attenuates depression-like behaviour in neonatal DEX-treated animals
The above experiments showed that activation of the amygdaloid MAPK signal cascade is responsible for the increase in depression-like behaviour in neonatal DEX-treated animals. In this experiment, we examined whether the co-administration of sub-threshold doses of MAPK inhibitor PD98059 would provide a rescue effect and attenuate depression-like behaviour. Initially, 26 rats were used and received stereotaxic surgery. Cannulas were placed in the lateral ventricle, six of them were excluded for showing abnormal behaviour or cannula misplacement. The final twenty rats were then randomly assigned to two groups and received either vehicle or PD98059 infusion. Fifteen minutes later, they were then subjected to the FST, as described previously. Sub-threshold dose treatment of PD98059 significantly attenuated depression-like behaviour in neonatal DEX-treated animals (p < 0.01). In the control group, PD98059 in a sub-threshold dose did not have any effect on the percentage of immobility (Fig. 5a). To exclude the possible non-specific effect of PD98059 on animals behaviour, all animals in this experiment were subjected to locomotor activity monitoring and the rota-rod task. No significant difference was found between the PD98059-treated group and the vehicle control group (Fig. 5b, c). Thus, the rescue effect of a sub-threshold dose of PD98059 observed during the FST appeared to result from the acute drug effect rather than from non-specific effects of PD98059 on motor functions.

Pre-treated with a sub-threshold dose of MAPK inhibitor suppressed depression-like behaviour in neonatal DEX-treated animals. (a) In the given sub-threshold dose PD98059 did not alter the expression of depression-like behaviour in control group. The increased depression-like behaviour in DEX-treated animals was suppressed by the MAPK inhibitor. (b) Compared with the vehicle group (control), a sub-threshold dose of PD98059 the DEX group showed no significant different in locomotor activity. (c) There was no significant difference in retention of the rota-rod task among groups. Each vertical bar represents the Mean±s.e.m. for each group. **p < 0.01 compared with the corresponding group.
Administration of a sub-threshold dose of PD98059 suppressed amygdaloid MAPK phosphorylation and restored LTP to normal range
According to the results of the above experiments, neonatal DEX treatment enhanced the acute stress effect on amygdaloid MAPK phosphorylation (Fig. 6, lane 2). The MAPK inhibitor PD98059 blocked the enhanced effect of neonatal DEX treatment on amygdaloid MAPK phosphorylation (Fig. 6, lane 4). In addition, we measured the expression of MAPK in amygdala. No significant difference was found. In addition, after being co-treated with PD98059, the enhanced MAPK phosphorylation was suppressed and returned to the normal range. The average magnitude of potentiation of the DEX-treated group, measured 50 min after tetanus stimulus, was 155 ± 7% (n = 6, p = 0.33) compared to the control group (147 ± 6%) and SAL group (Fig. 7).
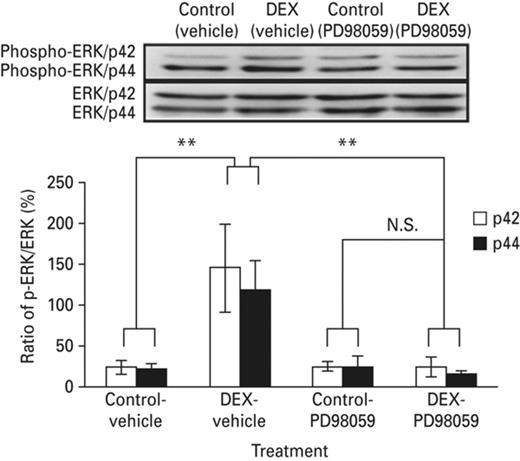
I.C.V. infusion of MAPK inhibitor PD98059 suppressed the increment of MAPK phosphorylation in the amygdala of the neonatal DEX-treated animals. The figure showed the differences in phosphorylation levels of amygdaloid MAPK among groups, the phosphorylation levels of were standardized to the total MAPK expression levels. The amygdaloid MAPK phosphorylation elevated in the neonatal DEX-treated group. The amygdaloid ERK phosphorylation level attenuated in the DEX-PD98059 treated group. Each vertical bar represents the Mean±s.e.m. for each group. Each vertical bar represents the Mean±s.e.m. **p < 0.01 compared with the corresponding group.
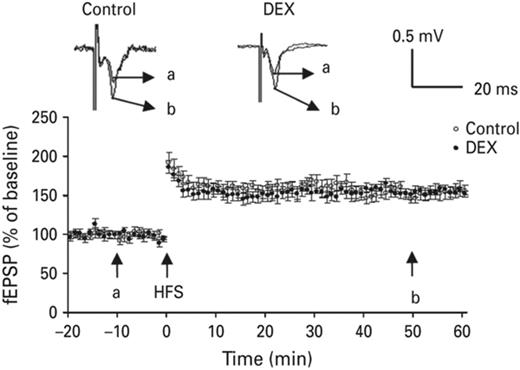
Amygdaloid LTP magnitude of neonatal DEX treated animals returned to normal range after treated with subthreshold dose of PD98059. Typical traces were displayed in the upper panel, a & b represent 10 min before induction and 60 min after induction, respectively. Each vertical bar represents the Mean±s.e.m.
Discussion
In the present study, we found that the depression-like behaviour was increased in neonatal DEX-treated animals after acute stress evoking. Compared with the control animals, the percentage of time spent in free floating is significantly increased in the neonatal DEX-treated group. Furthermore, neonatal DEX treatment elevated LTP formation and the phosphorylation level of MAPK in the lateral nucleus of amygdala (LA). Both of them can be restored to normal range after i.p. infusion and/or suprafusion of MAPK inhibitor PD98059. These results implied MAPK signalling cascade in LA plays an important role in the adverse effect of neonatal DEX treatment on amygdala function.
Previous studies demonstrated that dexamethasone treatment had long-term neurological effects, including an increased incidence of cerebral palsy and decreased cerebral volume (Lee et al., 2008; Wilson-Costello et al., 2009). In addition, results also suggest neonatal dexamethasone treatment impaired brain development and cognitive function (for review Barrington, 2001; Neal et al., 2004). Recent studies have shown that neonatal DEX treatment altered hippocampal synaptic plasticity and associative memory in rats (Kamphuis et al., 2003; Lin et al., 2006). Flagel and colleagues showed that DEX-treated animals are less active in light and dark environments, while administering a blunted serum corticosterone response to a novel stress (Flagel et al., 2002; Claessens et al., 2011). These data suggest that neonatal DEX treatment permanently alters central nervous system function, particularly within the neuroendocrine stress axis. This may lead to an increased risk of maladaptive responses to the environment. Some limbic structures including the hippocampus and amygdala were proven important to the feedback regulation on the HPA axis (Herman and Cullinan, 1997; Herman et al., 2005). Our results implied neonatal DEX treatment during early post-natal life might modify the developmental assembly of the amygdala, thereby providing a potential structural correlation for early experience-dependent effects on later responsiveness to emotionally evocative stimuli.
Although several studies implied that the long-term effects of neonatal DEX treatment on neurodevelopment and cognitive function do not persist into adulthood (Kamphuis et al., 2003; Lin et al., 2006), the behaviour testing in these studies were carried out without advance acute stress exposure. In the present study, neither the control nor the DEX-treated group showed a difference in the retention time and total moving distance of the rota-rod task and locomotor activity, respectively. These results indicated that the increasing effect of neonatal DEX treatment on depression-like behaviour was not the result of dysfunction of locomotor function or a decrease in spontaneous behaviour.
In summary, neonatal DEX injection significantly enhanced depression-like behaviours in adult rats. But the immobility time will remarkably increase in DEX-treated rats in the presence of flat top stress expose prior to FST. This result indicates that neonatal DEX-treated rats have no distinguishable differences in behaviour performance under normal conditions. However, neonatal DEX treatment influences distress tolerance throughout the rat's life span, which is more sensitive to acute stress and contributes to emotional or behavioural disorders.
Our results also demonstrated that MAPK phosphorylation in amygdala of neonatal DEX-treated rats elevated rapidly after FST with acute stress treatment compared with the control animals. Intracerebroventricular administration of PD98059 suppressed the hypothalamus and amygdala phosphorylation levels returned to basal level in DEX-treated rats. These results indicated that amygdaloid MAPK phosphorylation plays an important role in the regulation of immobility behaviour and the increment of MAPK activation is one of the major factors in prolonging immobility time in neonatal DEX-treated rats. We noted that a similar phenomenon was also observed in a maternal deprivation model (Huang and Lin, 2006), which found that the immobility time of rats that were subjected to maternal deprivation in early life was longer than that of control rats. Western blot analysis also showed that the phosphorylation level of amygdaloid MAPK in maternally deprived rats was almost two times higher than in control rats. In addition, intra-amygdala infusion of PD098059 or U0126, MEK inhibitors, suppressed immobility behaviour during the forced swim test in both groups. These results suggest that although various kinds of early life adverse and traumatic experiences could increase susceptibility to mood disorders in adulthood, similar signalling pathways might involve stress response modulation. Dysfunction between the limbic system and HPA axis interaction are considered to be the key features of affective disorder (Lupien et al., 2009; DeRijk et al., 2011; Flandreau et al., 2012).
Evidence suggests that the projection neurons in the LA, which receive thalamic sensory afferents are essential for learned fear (Sigurdsson et al., 2007; Pape and Pare, 2010; Meis et al., 2012). MAPK phosphorylation is required for both fear memory consolidation and LTP formation in LA (Schafe et al., 2000, 2008). Previous studies using fluoro-gold as a tracer showed that the c-fos positive neurons in the circuit from the hypothalamus to the amygdala greatly increased after the forced swimming test. These results indicate that the activation of this circuit participated in the process, which is induced by acute stress (Zhu et al., 2011). The electrophysiological recording analysis in our studies provides evidence that HFS results in hyperactive response to the DEX-treated rats’ neurocircuit projection from the paraventricular nucleus to the LA. The hyperactivity of hypothalamic to LA circuit LTP implies that limbic-HPA axis dysfunction is one of the major causes of depression-like behaviour in DEX-treated rats. In the present study, the increment of MAPK phosphorylation in the LA was confirmed in the brain tissue of neonatal DEX-treated rats, which dissected out immediately after FST. Suprafusion with a sub-threshold dosage of PD98059 reduced the hyperactivity response followed by HFS in the brain slices of neonatal DEX-treated rats, which did not have a non-specific effect on the basal fEPSP intensity. These results indicated that hyperactivity of the neurocircuit projects from hypothalamus to the LA rendered the animal hypersensitive to acute stressful events resulting in affective disorder. PD98059 suppressed MAPK phosphorylation after FST and effectively decreased DEX-treated rats’ immobility in FST without affecting those in the control group.
In conclusion, our data suggest that neonatal DEX treatment causesa long-lasting hyperactivation effect on the neurocircuit of the amygdala. Thus it can sensitize responses to acute stress in later life and is associated with the development of mood disorder in adulthood.
Acknowledgment
This study is supported by the National Science Council, Taiwan. Our gratitude also goes to the Academic Paper Editing Clinic, National Taiwan Normal University (APEC of NTNU).
References
Author notes
MCK and YHH contributed equally.