-
PDF
- Split View
-
Views
-
Cite
Cite
Kelly A. Butts, Anthony G. Phillips, Glucocorticoid receptors in the prefrontal cortex regulate dopamine efflux to stress via descending glutamatergic feedback to the ventral tegmental area, International Journal of Neuropsychopharmacology, Volume 16, Issue 8, September 2013, Pages 1799–1807, https://doi.org/10.1017/S1461145713000187
- Share Icon Share
Abstract
Enhanced dopamine (DA) efflux in the medial prefrontal cortex (mPFC) is a well-documented response to acute stress. We have previously shown that glucocorticoid receptors in the mPFC regulate stress-evoked DA efflux but the underlying mechanism is unknown. DA neurons in the ventral tegmental area (VTA) receive excitatory input from and send reciprocal projections to the mPFC. We hypothesize that blockade of prefrontal glucocorticoid receptors can reduce activity of descending glutamatergic input to the VTA, thereby attenuating stress-evoked DA efflux in the mPFC. Using in vivo microdialysis, we demonstrate that acute tail-pinch stress leads to a significant increase in glutamate efflux in the VTA. Blockade of prefrontal glucocorticoid receptors with the selective antagonist CORT 108297 attenuates stress-evoked glutamate efflux in the VTA together with DA efflux in the mPFC. Furthermore, blockade of ionotrophic glutamate receptors in the VTA attenuates stress-evoked DA efflux in the mPFC. We also examine the possible role of glucocorticoid-induced synthesis and release of endocannabinoids acting presynaptically via cannabinoid CB1 receptors to inhibit GABA release onto prefrontal pyramidal cells, thus enhancing descending glutamatergic input to the VTA leading to an increase in mPFC DA efflux during stress. However, administration of the cannabinoid CB1 receptor antagonist into the mPFC does not attenuate stress-evoked DA efflux in the mPFC. Taken together, our data indicate that glucocorticoids act locally within the mPFC to modulate mesocortical DA efflux by potentiation of glutamatergic drive onto DA neurons in the VTA.
Introduction
Enhanced dopamine (DA) efflux in the medial prefrontal cortex (mPFC) is a well-documented response to acute stress. We have previously shown that administration of the glucocorticoid receptor (GR) antagonist, RU-38486, into the mPFC significantly attenuates DA efflux in the mPFC and working memory impairments induced by exposure to acute tail-pinch stress (Butts et al., 2011). However, the underlying mechanism(s) by which the GR regulates stress-evoked DA efflux in the mPFC remains to be determined.
DA neurons in the ventral tegmental area (VTA) receive excitatory input from and extend reciprocal projections to the mPFC (Carr and Sesack, 2000). Local application of ionotropic glutamate receptor antagonists or functional inactivation of the DA cell bodies with lidocaine in the VTA decreased stress-evoked DA efflux in the mPFC (Enrico et al., 1998; Butts et al., 2011) suggesting that glucocorticoids released after acute stress may enhance descending glutamatergic feedback to the VTA. This is supported by evidence from the drug addiction field showing that deletion of GR in post-synaptic neurons innervated by DA terminals, but not in DA-releasing neurons, was associated with a reduction in spontaneous neural activity, firing rate and frequency of burst events in DA neurons, together with decreased glucocorticoid-induced cocaine self-administration by mice (Ambroggi et al., 2009).
Enhanced glutamatergic transmission in mPFC pyramidal neurons following exposure to acute stressors occurs through a GR-dependent mechanism (Yuen et al., 2009). Emerging evidence suggests a possible role of the endocannabinoid system in mediating GR activation into changes in glutamatergic transmission in mPFC neurons. Under conditions of acute stress, glucocorticoids trigger the synthesis and release of the endocannabinoid ligands, N-arachidonylethanolamine and 2-arachidonylglycerol (Di et al., 2003; Tasker et al., 2006; Hill et al., 2010). Endocannabinoid release from the post-synaptic cell acts on cannabinoid receptor subtype 1 (CB1) receptors localized on pre-synaptic glutamatergic, or GABAergic terminals, to inhibit subsequent neurotransmitter release (Di et al., 2003; Tasker et al., 2006; Hill et al., 2010). Activation of GRs in the mPFC can trigger endocannabinoid release which functions pre-synaptically via cannabinoid CB1 receptors to inhibit GABA release onto prefrontal pyramidal cells. This in turn leads to increased activity in descending glutamatergic input to the hypothalamus, thereby inhibiting the hypothalamic–pituitary–adrenal axis (Hill et al., 2011). A similar mechanism within the mPFC may explain how stress-induced elevation of glucocorticoids may mediate indirect activation of glutamatergic afferents to the VTA leading subsequently to increased DA efflux in the mPFC. This hypothesis is tested directly by blockade of CB1 receptors in the mPFC during exposure to acute stress.
We used in vivo microdialysis to investigate the role of the GR in mediating enhanced glutamatergic drive to the VTA. Using a selective GR antagonist, devoid of any progesterone activity, we demonstrate that local GR blockade in the mPFC attenuates stress-evoked glutamate efflux in the VTA and DA efflux in the mPFC. In contrast, blocking CB1 receptors in the mPFC failed to attenuate stress-evoked DA efflux. Antagonism of ionotropic glutamate receptor in the VTA attenuated DA efflux in the mPFC evoked by tail-pinch stress.
Materials and method
Animals
Male Sprague–Dawley rats (Charles River, Canada) were pair-housed. The colony was maintained at 21°C with a 12-h light/dark cycle (lights on 19:00 hours). Rats had free access to rat chow and water. All experimental protocols were approved by the Committee on Animal Care, University of British Columbia and conducted in compliance with guidelines provided by the Canadian Council of Animal Care.
Drugs
Stock solutions were made and dissolved in artificial cerebrospinal fluid (aCSF): RU-38486 (Tocris Biosciences, USA) was dissolved in ethanol. CORT 108297 (Corcept Therapeutics, USA) and CNQX, AP5, AM-251 and NIDA41020 (Tocris Bioscience) were dissolved in DMSO. Neither ethanol nor DMSO exceeded 0.1% when dissolved in aCSF.
In vivo microdialysis
Rats (∼280–310 g) were implanted with stainless steel guide cannulae (19 gauge × 15 mm) directly above the mPFC (3.0 mm AP, ± 0.6 mm ML from bregma, 4.6 mm DV from dura) and/or VTA (−5.8 mm AP, ± 0.6 mm ML, 8.0 mm DV). One week after surgery, microdialysis probes were implanted 14–16 h before experiments. Probes were concentric in design with silica inlet/outlet lines and a dialysis surface consisting of a semi-permeable membrane 1 or 2 mm in length (340 µm outer diameter; 65 000 molecular weight cut-off; Filtral 12; Hospal, UK). Typical in vitro probe (2 mm membrane) recovery of an external DA and glutamate standard is 18 ± 1% at room temperature. Microdialysis probes were connected to an Instech dual-channel liquid swivel and perfused continuously at 1.0 µl/min with aCSF [10 mm sodium phosphate, 1.2 mm CaCl2, 3.0 mm KCl, 1.0 mm MgCl2, 147.0 mm NaCl (pH 7.4)] using a 2.5-ml gas-tight syringe (Hamilton, UK) and a syringe pump (model 22; Harvard Apparatus, USA). Samples were collected at 15-min intervals. Following establishment of a stable baseline (four samples, <10% variability), the perfusion into the mPFC or VTA was switched to one containing RU-38486, CORT 108297, CNQX and AP5, AM-251 or NIDA41020, and DA or glutamate was continuously sampled from the mPFC and/or VTA. At 45 min after drug administration, animals were subjected to tail-pinch stress (clothes pin with Velcro covering on base of tail) for 15 min, and samples were continually collected for another 60–120 min.
HPLC
DA and glutamate in microdialysates were separated using HPLC with electrochemical detection.
DA HPLC
DA in microdialysates was separated using HPLC with electrochemical detection. Four systems were used, each consisting of a LS 110 HPLC pump (Antec Leyden, Netherlands), a pulse damper (Scientific Systems Inc., USA), a Rheodyne Inert Manual Injector (model 7725i, 50-ml injection loop), a Tosoh Bioscience Super ODS TSK column (2 µm particle, 2 mm × 10 mm) an Antec Leyden Intro Electrochemical Detector and VT-03 flow cell with a Ag/AgCl reference electrode (Vapplied=+650 mV). The mobile phase [100 mm sodium acetate buffer, 40 mg/l EDTA and 4 mg/l SDS (variable between 3.5 and 4.5 mg/l), pH 4.1, 10% methanol] flowed through the system at 0.17 ml/min. EZChrome Elite software (Scientific Software, USA) was used to acquire and analyse chromatographic data. The average concentration of basal DA in dialysates from the mPFC uncorrected for probe recovery was 0.16 ± 0.01 nm.
Glutamate HPLC
Dialysate samples were subjected to precolumn derivatization with o-phthaldialdehyde (OPA) reagent before injection. 1 µl OPA reagent (37 mm OPA, 50 mm sodium sulfite, 90 mm boric acid, pH 10.4, 5% methanol) was mixed with 9 µl sample and allowed to react for 2 min before injection into the ALEXYS GABA and Glu Analyzer using an AS 110 autosampler with a UHPLC injector (Antec Leyden). Mobile phase (50 mm phosphoric acid, 50 mm citric acid, 0.1 mm EDTA, 0.5% acetonitril, pH 3.1), pumped at 200 µl/min by a LS 110S HPLC pump (AntecLeyden), delivered analytes onto an Acquity UPLC HSS T3 column (1.8 particle, 1.0 × 50 mm). After separation on the column, electrochemical detection was performed with Decade II Dual Cell Control (Antec Leyden). Amino acid derivatives were oxidized in a VT-03 flow cell with an Ag/AgCl reference electrode (Vapplied=+800 mV). Clarity software (Antec Leyden) was used to acquire and analyse chromatographic data. The average concentration of basal glutamate in dialysates from the mPFC and VTA uncorrected for probe recovery was 0.62 ± 0.16 and 0.76 ± 0.12 µm, respectively.
Histology
Following all experiments, rats were killed after being deeply anaesthetized with isoflurane. Brains were promptly removed and stored in 20% w/v sucrose and 4% v/v paraformaldehyde solution for a minimum of 1 wk. Brains were then sliced into 50 µm coronal sections, stained with Cresyl Violet and examined for verification of probe placement.
Results
Blockade of mPFC GRs with a selective antagonist attenuates stress-evoked DA efflux in the mPFC
These data confirmed our previous observation that tail-pinch stress leads to a rapid and transient increase in DA efflux in the mPFC, reaching maximum levels 15 min after the onset of stress and returning to basal values after 90 min (Fig. 1a). Importantly, this effect was attenuated by pretreatment with the selective GR antagonist CORT 108297, which unlike RU-38486, is devoid of antiprogesterone action (Asagami et al., 2011; Belanoff et al., 2011). Application of CORT 108297 (10 µm) into the mPFC via reverse microdialysis significantly attenuated stress-evoked DA efflux (n = 9 vehicle treatment group, n = 10 CORT 108297 treatment group; Fig. 1a). Comparison of area under the time–response curves (AUCs) over the initial 90 min after stress exposure, in the CORT 108297 group with vehicle controls, revealed that this selective GR antagonist significantly attenuated stress-induced DA efflux in the mPFC (p < 0.05; Fig. 1b).
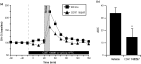
Selective glucocorticoid receptor antagonist attenuates stress-evoked dopamine (DA) efflux in the medial prefrontal cortex (mPFC). (a) Time course of the change of DA efflux from baseline measured within the mPFC while animals are subject to tail-pinch stress (15 min; grey-shaded box). CORT 108297 (horizontal black line) was delivered into the mPFC via reverse microdialysis. All values are expressed as percent change from baseline ± s.e.m. (n = 9, vehicle; n = 10, CORT 108297). (b) Histogram depicting mean area under curve (AUC; ± s.e.m.) for the change in DA efflux relative to baseline for 90 min after the initiation of stress (* p < 0.05).
Prefrontal GR blockade attenuates stress-evoked glutamate efflux in the VTA
Tail-pinch stress induced a rapid and transient increase in glutamate efflux in the VTA reaching maximum values 15 min after the onset of stress, returning to basal values at 90 min (Fig. 2a). Infusion of RU-38486 (23 µm) or CORT 108297 (10 µm) into the mPFC via reverse microdialysis significantly attenuated stress-evoked glutamate efflux (Fig. 2a). Interestingly, we did not observe an increase in stress-induced efflux of glutamate in the mPFC (Fig. 2b). Comparison of AUCs for the initial 60 min after stress exposure revealed a significant reduction of glutamate efflux in the VTA after intra-mPFC injections of either RU-38486 or CORT 108297 (p < 0.05, two-tailed Student's t test; Fig. 2c). There was no significant difference between treatments on glutamate efflux in the mPFC (p > 0.05, two-tailed Student's t test; Fig. 2d).
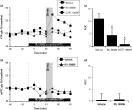
Glucocorticoid receptors in the medial prefrontal cortex (mPFC) regulate stress-evoked glutamate (glu) efflux in the ventral tegmental area (VTA). (a, b) Time course of the change from baseline of glutamate efflux in the VTA (a) and mPFC (b) of animals subjected to tail-pinch stress (15 min; grey-shaded box). CORT 108297 or RU-38486 (horizontal black line) was delivered via reverse microdialysis into the mPFC (n = 10, vehicle; n = 9, RU-38486) and/or VTA (n = 10, vehicle; n = 10, RU-38486; n = 6, CORT 108297). All values are expressed as percent change from baseline ± s.e.m. (c,d) Histograms depicting mean area under curve (AUC; ± s.e.m.) for the change in glu efflux relative to baseline for 60 min after the initiation of stress (* p < 0.05).
Blockade of ionotropic glutamate receptors in the VTA reduces stress-evoked DA efflux in the mPFC
We next perfused the AMPA receptor antagonist, CNQX (50 µm) and the NMDA receptor antagonist, AP5 (200 µm), into the VTA via reverse microdialysis while measuring DA efflux in the mPFC during tail-pinch stress. Local perfusion of CNQX and AP5 into the VTA has been previously shown to decrease DA efflux in the mPFC during handling stress (Enrico et al., 1998). Blockade of ionotropic glutamate receptors in the VTA significantly decreased stress-evoked DA efflux in the mPFC (n = 8 vehicle treatment group, n = 6 CNQX and AP5 treatment group; Fig 3a), measured by AUC (mean ± s.e.m.) over 90 min post-stress (p < 0.05; Fig. 3b).
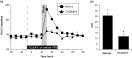
Ionotrophic glutamate receptors in the ventral tegmental area (VTA) mediate stress-evoked dopamine (DA) efflux in the medial prefrontal cortex (mPFC). (a) Time course of the change from baseline of DA efflux in the mPFC measured from while animals were subject to tail-pinch stress (15 min; grey-shaded box). CNQX or AP5 (horizontal black line) were perfused into the VTA via reverse microdialysis. All values are expressed as percent change from baseline ± s.e.m. (n = 8, vehicle; n = 6, CNQX and AP5). (b) Histogram depicting mean area under curve (AUC; ± s.e.m.) for the change in DA efflux relative to baseline for 90 min after the initiation of stress (* p < 0.05).
Cannabinoid CB1 receptors do not modulate stress-evoked DA efflux in the mPFC
We also examined the possible role of glucocorticoid-induced synthesis and release of endocannabinoids which may act presynaptically via cannabinoid CB1 receptors to inhibit GABA release onto prefrontal pyramidal cells, thus enhancing descending glutamatergic input to the VTA during stress leading to increased DA efflux in the mPFC (Hill et al., 2011). When administered locally into the mPFC, neither the CB1 receptor antagonist AM-251 (10 µm) nor NIDA41020 (10 µm) had a significant effect on stress-induced DA efflux in the mPFC (n = 8 vehicle, n = 9 AM-251, n = 8 NIDA41020; Fig. 4a) as measured by comparison of AUCs (p > 0.05, two-tailed Student's t test; Fig. 4b).
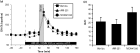
Stress-evoked dopamine (DA) efflux in the medial prefrontal cortex (mPFC) is not mediated by cannabinoid CB1 receptors. (a) Time course of the change from baseline of DA efflux in the mPFC in rats subjected to tail-pinch stress (15 min; grey-shaded box). AM-251 or NIDA41020 (horizontal black line) was perfused into the mPFC via reverse microdialysis. All values are expressed as percent change from baseline ± s.e.m. (n = 8, vehicle; n = 7, AM-251; n = 8, NIDA41020). (b) Histogram depicting mean area under curve (AUC; ± s.e.m.) for the change in DA efflux relative to baseline for 90 min after the initiation of stress.
Histology
Histological analysis confirmed the location of all microdialysis probes from all experiments to be localized in either the prelimbic or infralimbic areas of the mPFC and the VTA (Fig. 5).
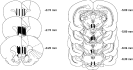
Placements of microdialysis probes. Each vertical black bar represents the active membrane surface location of a microdialysis probe in the medial prefrontal cortex or ventral tegmental area. Not all probes are shown, because of overlapping placements.
Discussion
The present study provides the novel observation that glutamate efflux in the VTA is increased in a phasic manner after exposure to tail-pinch stress that can be attenuated by reverse microdialysis of the selective GR antagonist CORT 108297 into the mPFC. Furthermore, blockade of ionotropic glutamate receptors in the VTA leads to a significant reduction in stress-evoked DA efflux in the mPFC. These data support the hypothesis that glucocorticoids act locally within the mPFC to modulate mesocortical DA efflux by potentiation of glutamatergic drive onto DA neurons in the VTA. As similar effects were obtained in a recent study with the progesterone and GR antagonist RU-38486 (Butts et al. 2011), using the selective GR antagonist CORT 108297 (Asagami et al., 2011; Belanoff et al., 2011), the present data suggest that the observed attenuation of stress-induced DA efflux is mediated by a glucocorticoid and not a progesterone receptor. These results also support our contention that the mPFC is the primary site of glucocorticoid regulation of mesocortical DA neurons during stress.
Although we observed a significant increase in stress-induced glutamate efflux in the VTA, a similar increase in glutamate efflux in the mPFC was not seen in the same animals. Previous studies examining the effects of handling stress on glutamate efflux also failed to detect an increase in the mPFC (Timmerman et al., 1999; Del Arco and Mora, 2001). However, these negative findings differ from previous observations demonstrating an increase in glutamate release in the mPFC of rats exposed to acute restraint or tail-pinch stress using brain microdialysis (Moghaddam, 1993; Bagley and Moghaddam, 1997). Furthermore, Hascup and colleagues employed enzyme-based microelectrode arrays to measure an increase in neuronally derived glutamate in the mPFC after exposure to both tail-pinch and restraint stress (Hascup et al., 2011, 2012). One possible explanation for our failure to observe a significant increase in mPFC glutamate efflux with a tail-pinch stressor may reflect the relatively mild nature of this stimulus. Alternatively, the detection limit of the analytical system used in the present study (0.05 µm) may not have had sufficient sensitivity.
Previous studies examining the mechanisms by which acute stress or corticosterone administration regulates glutamatergic transmission have focused on identifying the transcriptional targets of GR activation. Yuen and colleagues demonstrated that the stress-induced delayed and sustained potentiation of NMDAR- and AMPAR-mediated synaptic currents in layer V mPFC pyramidal cells mediated by GR activation occurs through the induction of serum- and glucocorticoid-inducible kinases and the activation of Rab4 that regulates receptor trafficking and function of NMDARs and AMPARs (Yuen et al., 2009, 2011). Revest and colleagues demonstrated that activation of GRs leads to an increase in the expression of the transcription factor early gene response-1 in the hippocampus (Revest et al., 2005, 2010). This, in turn, can increase expression of synapsin-Ia/Ib, the most highly expressed hippocampal presynaptic vesicle-associated phosphoproteins (Revest et al., 2005, 2010), which exert direct control over the release of neurotransmitters, such as glutamate (Nichols et al., 1992; Jovanovic et al., 2000). In contrast, in the present study, the increase in stress-evoked glutamate efflux in the VTA was observed within 15 min. As this short time-frame of action is too rapid to be mediated by means of the classical genomic GR pathway as described above, the immediate effects of acute stress may instead be regulated by a non-genomic membrane receptor-mediated mechanism (Prager and Johnson, 2009; Groeneweg et al., 2011).
Emerging evidence suggests that corticosterone may rapidly affect neuronal signalling by binding to membrane-associated corticosterone receptors (Orchinik et al., 1991; Dallman, 2005; Haller et al., 2008; Prager and Johnson, 2009) and anatomical data confirm that GRs are localized to membranes and synapses (Liposits and Bohn, 1993; Johnson et al., 2005). These membrane receptors have been shown to rapidly change neuronal excitability through direct actions on ion channels or intracellular signalling messengers (ffrench-Mullen, 1995; Tasker et al., 2006; Prager and Johnson, 2009; Groeneweg et al., 2012). Relatively few studies have examined the possible role of rapid non-genomic mechanisms in mediating corticosterone signalling in the mPFC (Groeneweg et al., 2011). However, a recent behavioural study conducted by Barsegyan and colleagues demonstrated that administration of corticosterone conjugated to the membrane impermeable protein, bovine serum albumin, into the mPFC impaired performance on a working memory task (Barsegyan et al., 2010). This effect was prevented by co-administration of RU-38486 and discovered to be mediated by a membrane GR-induced facilitation of a noradrenergic signal transduction pathway within the mPFC (Barsegyan et al., 2010). Accordingly, we propose that corticosterone acts on a putative membrane GR of layer V pyramidal neurons in the mPFC (Fig. 6). Activation of layer V cortical neurons, the principal projection neurons of the mPFC, would, in turn, enhance glutamatergic drive onto DA neurons in the VTA, leading to an increase in DA efflux in the mPFC (Fig. 6; Carr and Sesack, 2000). As previously mentioned, the endocannabinoid signalling molecules are a well-studied retrograde messenger system regulated by membrane GRs (Tasker et al., 2006). Therefore, we investigated whether the present findings may be mediated by glucocorticoid-induced synthesis and release of endocannabinoids that would in turn act presynaptically via cannabinoid CB1 receptors to remove GABAergic inhibition of layer V pyramidal neurons in the mPFC (Hill et al., 2011) and thus enhancing DA efflux in the mPFC. However, blockade of CB1 receptors in the mPFC failed to attenuate stress-evoked DA efflux. Although the concentration of AM-251 and NIDA-41020 used in the present study was effective in previous behavioural and electrophysiological experiments (Di et al., 2003; Miller et al., 2007; Sousa et al., 2011), the possibility remains that a higher concentration of these drugs is required to produce positive effects. However, given this finding, it is noteworthy that glucocorticoid-induced synthesis and release of endocannabinoids acting presynaptically via cannabinoid CB1 receptors to inhibit GABA release in the mPFC was not observed until 1 h after stress exposure (Hill et al., 2011). Future studies examining other rapid GR-mediated non-genomic mechanisms regulating corticosterone signalling in mPFC projection neurons are warranted.

Schematic depiction of the anatomical connections between the medial prefrontal cortex (mPFC) and ventral tegmental area (VTA) proposed to mediate stress-evoked dopamine (DA) efflux in the medial mPFC. Specifically, we hypothesize that elevated circulating corticosterone during stress activates putative membrane glucocorticoid receptors (GR) on prefrontal glutamatergic projection neurons that terminate on DA neurons in the VTA. Increased activation of these projection neurons by corticosterone would subsequently increase VTA DA neuron cell firing thereby augmenting DA release in the mPFC (black line indicates the glutamatergic projection, the grey line depicts the mesocortical dopaminergic projection).
In summary, blockade of GRs within the mPFC with a selective antagonist attenuates stress-evoked DA and glutamate efflux in the mPFC and VTA, respectively. Blockade of ionotrophic glutamate receptors in the VTA also reduces stress-evoked DA efflux in the mPFC, thereby confirming an important contribution of mPFC GRs to the modulation of ‘top-down’ feedback control of mesocortical DA neurons (Phillips et al., 2008). Together, these findings may offer a partial explanation of stress-induced disruption and possibly facilitation of cognitive functions mediated by neural activity within the mPFC.
Acknowledgements
We thank Dr Joseph Belanoff for his generous donation of CORT 108297, Dr Soyon Ahn, Kitty So and Giada Vacca for technical assistance with the HPLC, and Dr Allan Young and Lasse Dissing-Olesen for helpful advice and feedback.
Statement of Interest
A.G.P. serves on the board of Allon Therapeutics Inc. and owns shares in the company.
References