-
PDF
- Split View
-
Views
-
Cite
Cite
Timo T. Schmidt, Ellis Rea, Julia Shababi-Klein, George Panagis, Christine Winter, Enhanced reward-facilitating effects of d-amphetamine in rats in the quinpirole model of obsessive–compulsive disorder, International Journal of Neuropsychopharmacology, Volume 16, Issue 5, June 2013, Pages 1083–1091, https://doi.org/10.1017/S1461145712000983
- Share Icon Share
Abstract
The underlying neurobiology of addictive or repetitive behaviours, such as obsessive–compulsive disorder (OCD), involves dopaminergic dysregulation. While addictive behaviour depends strongly on mesolimbocortical dopaminergic responses, repetitive behaviours have been associated with dopaminergic dysregulation in the basal ganglia–thalamo–cortical circuitry. The present study investigates differences in brain stimulation reward in rats with quinpirole-induced compulsive checking behaviour, in order to examine if deficits in reward processing are also relevant for OCD. Rats were tested in the intracranial self-stimulation (ICSS) paradigm, which targets reward-related responses. After phenotype induction, animals were implanted with a monopolar stimulation electrode in the left medial forebrain bundle and trained to press a lever to self-administer electric stimulation of varying frequency. The curve-shift method was used to assess the reward-facilitating effects of d-amphetamine and the reward-attenuating effects of haloperidol (a D2 antagonist). Thresholds for ICSS were estimated before and after drug/saline injection. The reward-facilitating effects of d-amphetamine were enhanced in quinpirole-treated rats in comparison to controls. This finding suggests that chronic quinpirole-treatment induces changes within the reward circuitry relevant for compulsive behaviour in the rat.
Introduction
Obsessive–compulsive disorder (OCD) is a highly afflicting psychiatric illness with a lifetime prevalence of 1–2% (Hollander, 1997; Rasmussen & Eisen, 1994; Weissman et al.1994). Patients suffering from OCD demonstrate a loss of control over habits and/or thoughts/impulsions leading to behaviours and thought patterns that can be extremely time-consuming and strongly interfere with everyday life. Current treatment options are limited and often ineffective, meaning that there is a demand for investigation of new therapeutic targets and strategies.
Although the aetiology of OCD is largely unknown, the dopaminergic and serotonergic systems have been implicated in its pathophysiology (Nikolaus et al.2010; Saxena et al.1998). Animal experimental data and human clinical studies converge to indicate that dopaminergic dysregulation within the basal ganglia–thalamo–cortical circuitry plays a crucial role in symptom manifestation (Lopes et al.2004; Mundt et al.2009). Moreover, clinical observations correlate OCD with disturbances of the brain reward system (Cohen et al.2012; Jung et al.2011; Palminteri et al.2012), i.e. behavioural aspects of OCD have been related to unadaptive perception of reward. Additionally, the high prevalence of OCD among substance abuse patients and the high co-morbidity of addictive behaviour in OCD patients indicate potential pathological changes within the reward system (Blom et al.2011).
Much of the current knowledge about the reward circuitry has been gained from experiments that specifically target different brain areas with electric stimulation. The findings of Olds & Milner (1954), that rats self-administer electric stimulation to brain areas such as the ventral tegmental area or the medial forebrain bundle (MFB), have been especially influential in the characterization of the mesolimbic dopamine system as the main reward pathway of the brain. Over several decades, the operant paradigm of intracranial self-stimulation (ICSS) has been used to examine and quantify changes in hedonic, anhedonic and motivational states in rodents. In ICSS experiments, rodents self-administer rewarding electrical stimulation (often referred to as brain stimulation reward) through electrodes implanted directly into the brain, typically into the MFB. The curve-shift variant of ICSS experiments allows quantification of the reward-facilitating or -attenuating effects of a drug treatment. Animal's self-stimulation thresholds for electric pulses of varying frequency are remarkably stable over weeks and months in well-trained animals. This allows quantifying drug related threshold shifts between a pre- and post-drug treatment measurement. A threshold decrease implies that the stimulation is more rewarding as a result of treatment (reflecting hyperfunction of brain reward systems), whereas a threshold increase implies that it is less rewarding (reflecting hypofunction of reward systems; Carlezon & Cartoff, 2007).
The quinpirole (QNP) rat model of OCD was proposed by Szechtman (1998, 2001) and validated in pharmacological (Tizabi et al.2002), lesion (Dvorkin et al.2010) and deep brain stimulation (Djodari-Irani et al.2011; Mundt et al.2009; Winter et al.2008) studies. In the QNP model, rats are injected twice weekly with the D2/D3 agonist QNP and undergo training/testing on a modified open field after each injection. Over 5 wk a phenotype of compulsive checking behaviour develops and can be stably maintained by continuation of QNP treatment. The genesis of compulsive checking is not mere sensitization to QNP; instead, the combination of QNP application and exposure to an environment that evokes checking behaviour contributes to the development of the compulsive phenotype (Eilam & Golani, 1989; Eilam & Szechtman, 2005; Eilam et al.2011; Einat et al.1993, 1996; Szechtman et al.1998, 2001; Szumlinski et al.1997).
The present study addresses the question of whether rats exposed to chronic-intermittent QNP treatment – known to induce compulsive checking – show altered reward processing as tested in the curve-shift variant of the ICSS paradigm.
Materials and method
Animals
Twenty-six naive male Wistar rats (Harlan-Winkelmann, Germany; 160–180 g at the start of the experiment) were housed in a temperature and humidity controlled vivarium with a 12-h light–dark cycle (lights on 06:00 hours). Animals were housed in groups of six before electrode implantation and single housed afterwards. All experiments were performed during the day. Food and water were available ad libitum. The present study was carried out in accordance with the European Communities Council Directive of 24 November 1986 (86/609/EEC) for the care of laboratory animals and after approval of the Local Ethics Committee (Senate of Berlin). All efforts were made to reduce animal suffering and to reduce the number of animals used.
Design
The experiment consisted of three phases: (1) induction of the obsessive checking phenotype; (2) induction of self-stimulation and training in the ICSS procedure; (3) ICSS test sessions. Animals were injected twice weekly with QNP (QNP group: n = 13, included in the analysis n = 9) or saline (control group: n = 13, included in the analysis n = 9) shortly followed by an open field testing. This treatment was continuously performed over the course of the whole experiment, including all three experimental phases. Surgeries for electrode implantation were performed between the 7th and 9th injection [body weight QNP: mean = 322 g, s.d. = 14.8 (n = 9); control: mean = 313 g, s.d. = 21.2 (n = 9)]. ICSS training started after the 10th injection after confirmation of manifestation of compulsive checking. Whereas ICSS training was performed daily, ICSS test sessions were carried out on QNP-injection days, only with a time delay of at least 4 h after QNP administration. After the last ICSS test session, animals were administered with one last QNP injection (mean number of injections QNP: 23.11, s.d. = 3.98; control: 23.78, s.d. = 4.26) and were then killed (Fig. 1).
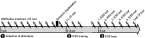
Experimental design. Animals received quinpirole (QNP) injections with consecutive open field (OF) tests twice per week over the course of the whole experiment (syringe symbols), including: (1) phenotype induction phase; (2) intracranial self-stimulation (ICSS) training phase; (3) ICSS test phase. After electrode implantation, animals were trained daily (on injection days and on no-injection days) in the ICSS procedure until stimulation thresholds stabilized. Five ICSS test sessions were carried out in a randomized order on injection days. To demonstrate that the compulsive phenotype was stable over the course of the study, open field data for the 11th and last open field test were assessed.
Phenotype induction and monitoring
Systemic drug administration
(−)-Quinpirole hydrochloride (Sigma, USA) was dissolved in 0.9% NaCl to a concentration of 0.5 mg/ml and injected subcutaneously under the nape of the neck at a dose of 0.5 mg/kg. Control animals received the same volume of saline.
Apparatus and behavioural procedure
Fifteen min after each QNP or saline administration, animals were placed in a modified open field for 30 min. The open field consisted of a glass table (140 × 140 cm and 20 cm high) with four Plexiglas boxes varying in shape and size at fixed locations. The platform was subdivided into 25 rectangles (locales). A computer, interfaced with a video recorder, was used to score locomotor behaviour during playback of video records (TSE VideoMot 2 system; Technical & Scientific Equipment, Germany). For each rat the total distance travelled and the frequency of stops at each open field locale was assessed, where stops/visits refer to periods of no locomotion (Szechtman et al.1998). For each rat, the locale with the highest total number of stops was defined as the home base (HB) (Eilam & Golani, 1989) and compulsive checking behaviour was analysed with reference to the HB. According to Szechtman et al. (2001), compulsive checking behaviour is present if a rat meets the following three criteria: (i) the rat returns to the HB excessively often; (ii) excessively rapidly; (iii) visits less places before returning to the HB in comparison to control rats. The following measures were therefore analysed for each rat and open field session: (i) the total number of visits to the HB; (ii) the mean time to return to the HB; (iii) the mean number of stops/visits before returning to the HB. Because checking behaviour requires locomotion, and repeated administration of QNP increases locomotion (Szechtman et al.1994; Szumlinski et al.1997), a calculation was applied to allow the assessment of altered checking behaviour independent of changes in locomotion: the ratio of observed to expected HB visits. This is a measure for the number of HB visits normalized regarding the total number of visits and the number of locales visited by the rat (Mundt et al.2009). Locomotor activity was recorded and analysed for the 11th and the last open field session before animals were killed. QNP-treated rats meet additional criteria for compulsive checking, i.e. ritual-like behaviour and context dependency, which have repeatedly been demonstrated to behave in a similar way to the parameters mentioned above (Szechtman et al.1998, 2001). These criteria were therefore not evaluated in this study.
Data analysis
Open field data were analysed using 2 × 2 mixed-design analyses of variance (ANOVAs) with the factor phenotype (QNP, control) as the between-subject variable and the time point of measurement (11th, last) as the within subject-variable. Multiple post-hoc t tests were Bonferroni's corrected. SPSS 19.0.0.1 Statistics (IBM Corporation, USA) was used for the analysis.
Intracranial self-stimulation
Surgery for electrode implantation
Stereotaxic implantations were performed between the 7th and 9th QNP injection under sodium pentobarbital anaesthesia (60 mg/kg i.p.). The incisor bar was set at 3.3 mm below the interaural line. A monopolar stainless steel stimulation electrode (MS303/1-AIU/SPC; Plastics One Inc., USA) was implanted into the MFB of the left hemisphere at the level of the lateral hypothalamus (coordinates anterior–posterior: −2.5 mm from bregma; mediolateral: 1.7 mm from midline; dorsoventral: −8.0 mm from the surface of the dura, according to Paxinos & Watson 1998). The anode was wrapped around the four fixation screws. The electrode assembly was fixed using dental cement (Technovit: Heraeus Kulzer GmbH, Germany).
Apparatus and procedures for self-stimulation
One week after surgery, the animals were tested for self-stimulation in an operant chamber 28 × 28 × 24 cm (TSE Systems, Germany) equipped with a plastic floor. A stainless-steel rodent lever protruded 4 cm from the wall at a height of 3 cm. Each lever press triggered a computer-interfaced constant current generator (STG4004; Multi Channel Systems GmbH, Germany) that delivered a 0.4 s train of rectangular cathodal pulses of 0.1 ms constant duration. Rats were connected to the current generator by cables with mesh or spring covering via a swivel (SL2C/SB; Plastics One Inc.). All experimental procedures were automatically executed and controlled by self-made MATLAB code (the MathWorks, USA). Response rates were assessed by the automatic counting function of the current generator.
For initiation of self-stimulation (1–3 d), current intensity was set to 250 µA and the number of pulses per stimulation train (NP) was held constant at 50 NP. If animals failed to respond to stimulation or showed side-effects, the current intensity was adjusted (190–400 µA). After self-stimulation stabilized at rates >40 presses/min the individual current intensity was held constant for the rest of the experiment. During the ICSS training phase (2–3 wk) the animals were tested daily in rate-frequency (R/F) sessions until their stimulation threshold stabilized (<10% variation over three consecutive days). During the training phase the delay between QNP injection and ICSS training varied between 4–8 h (also within animal) on injection days. On the last training days (when thresholds were close to being stable), the animals were tested at the approximate time of their scheduled ICSS test session to ensure no time-dependent effects between ICSS test sessions. For each animal the time and the time delay between QNP injection and ICSS test was held as constant as possible over the course of its ICSS test sessions. Each R/F session lasted approximately 45 min. A session constituted 1 min trials, where lever pressing elicited stimulation. Each trial started with five priming stimulations and was followed by a 30 s time-out, where lever pressing did not lead to stimulation. The stimulation frequency was varied over trials in steps of 0.1 log units ranging between 15 and 200 Hz corresponding to 6–80 NP. The range of frequencies tested on each individual animal was adjusted to obtain trials at each end of the range, where stimulation was ineffective and where lever pressing had reached a maximum rate. The number of lever presses in each trial was recorded. Trials were presented as a series of descending and ascending frequencies with four trials per frequency (Panagis et al.2000). To obtain R/F functions, the four data points for each frequency were averaged. In ICSS test sessions the stimulation threshold and asymptote were first assessed in a baseline R/F function (pre-drug). After drug administration, a second R/F function (post-drug) was determined and compared to the pre-drug function to reveal drug-induced curve-shifts and corresponding alterations in threshold and asymptote.
Drugs and drug administration
In the ICSS test sessions, animals were challenged with haloperidol (Ratiopharm), d-amphetamine (A-5880; Sigma) or saline in five conditions: (1) 0.05 mg/kg haloperidol (highHalo); (2) 0.01 mg/kg haloperidol (lowHalo); (3) vehicle (saline); (4) 0.1 mg/kg d-amphetamine (lowAmph); (5) 0.5 mg/kg d-amphetamine (highAmph). All substances were dissolved in 0.9% NaCl and injected i.p. at a volume of 1.0 ml/kg. The sequence of test conditions was randomized.
Data analysis and statistical treatment
Data gathered from ICSS tests were curve-fitted and threshold and asymptote estimates were obtained. For fitting the R/F function, we used the Gompertz sigmoid model (Coulombe & Miliaressis, 1987), as previously described (Panagis et al.2000). The threshold represents the frequency producing 36.7% of the asymptotic response rate. Fitting of Gompertz sigmoid models to the ICSS data was performed by self-made MATLAB code using the statistics toolbox for the nonlinear regression. Single trials were excluded from the analysis, if an animal failed to respond in a particular trial, while substantially responding in the previous and following trial (Carlezon & Chartoff, 2007). Threshold and asymptote shifts were statistically evaluated with mixed-design ANOVAs with the group (QNP, control) as between-subject variable and the condition as within-subject variable. Multiple post-hoc t tests were Bonferroni corrected. Statistical analysis was performed using SPSS 19.0.0.1. The post-drug threshold and asymptote values were expressed as percentage of pre-drug values. Because animals presented individually characteristic curve-shifts in the saline condition, data were normalized for this shift and the threshold changes for highHalo, lowHalo, lowAmph, highAmph were calculated as the difference to the change in the saline condition.
Histology
After completion of the experimental procedure, the animals were given a lethal dose of chloral hydrate. Brains were removed from the skull and snap frozen. Then, 20 µm coronal sections were Nissl-stained for the reconstruction of the electrode localization by light microscopic inspection (data presented in Supplementary Fig. S1).
Results
Eighteen of the 26 animals were included in the data analysis (QNP: n = 9; control: n = 9). Six animals did not respond to the stimulation because of either electrode dysfunction or electrode dislocation. One animal was excluded due to a loss of the electrode assembly during the ICSS tests. One animal was excluded because it did not present a compulsive checking phenotype, despite QNP treatment.
QNP-induced compulsive checking behaviour
Chronic QNP treatment induced compulsive checking behaviour as demonstrated with four performance measures of compulsive checking previously introduced by Szechtman et al. (1998) . This phenotype was stable by the 10th injection and remained so over the course of the experiment. QNP-treated rats visited their HB more often than controls (F1,16 = 274.24; p < 0.001). No significant differences were found in the within-subject contrasts (F1,16 = 3.05; p = 0.100) or the interaction of between- and within-subject contrasts (F1,16 = 3.18; p = 0.094), showing that the phenotype was stable over the course of the experiment (Fig. 2a). QNP-treated rats had a higher ratio of observed to expected HB visits than controls (F1,16 = 194.65; p < 0.001), which was stable for the duration of the study (within-subject: F1,16 = 0.142; p = 0.711; interaction: F1,16 = 0.578; p = 0.458; Fig. 2b). QNP-treated rats returned faster to their HB than controls (F1,16 = 76.25; p < 0.001), which was stable for the duration of the study (within-subject: F1,16 = 0.004; p = 0.951; interaction: F1,16 = 0.525; p = 0.479; Fig. 2c). QNP-treated animals made fewer stops before returning to their HB compared to controls (F1,16 = 13.103; p = 0.002), which was stable for the duration of the study (within-subject: F1,16 = 0.26; p = 0.617; interaction: F1,16 = 0.72; p = 0.409; Fig. 2d). In addition, QNP treatment was found to increase locomotion (F1,16 = 205.45; p < 0.001; within-subject: F1,16 = 11.27; p = 0.004; interaction: F1,16 = 11.07; p = 0.004). Although locomotion of controls did not change over the course of the experiment, total locomotion of QNP-treated rats further increased from the 11th to the last open field test (t16 = 3.446; p < 0.01; Fig. 2e).
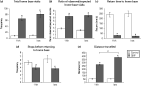
Quinpirole (QNP)-induced compulsive checking behaviour. Compulsive checking behaviour was analysed with reference to the home base, recognized as the locale with the highest frequency of stops. Data are presented for the 11th and last open field session and demonstrate a stable phenotype of compulsive checking behaviour over the course of the whole intracranial self-stimulation test procedure. QNP-treated animals met compulsive checking criteria: (a) more frequent returns to the home base; (b) a higher than expected rate of returning to the home base; (c) reduced return time to home base; (d) fewer visits to other places before revisiting home base compared to saline-treated controls. Additionally, QNP-treated rats displayed increased locomotion as measured by the total distance travelled over the 30 min observation period (e). *p < 0.05, t test. Error bars represent s.e.m.
Intracranial self-stimulation
Figure 3 presents the R/F functions of two representative animals; one from the QNP-treated group and one control. Animals demonstrate a shift of their R/F function to the left after highAmph treatment and to the right after highHalo treatment. The application of haloperidol or d-amphetamine at a low dose had no significant effects on stimulation thresholds compared to saline treatment. However, the animals demonstrated increases in ICSS thresholds after saline treatment (QNP: mean = 19.53%, s.d. = 7.72; control: mean = 9.58%, s.d. = 7.33), which were higher in QNP-treated animals (t16 = − 2.915, p = 0.010). To correct for this drug-unrelated bias in threshold changes, data were normalized to the change in the saline condition for each individual animal. The changes in ICSS threshold after each drug injection for the QNP and control group are presented in Fig. 4. A 2 × 4 mixed-design ANOVA was performed for group (QNP, control) and conditions (highHalo, lowHalo, lowAmph, highAmph). The within-subject contrasts were significant (F3,16 = 103.706; p < 0.001), demonstrating an effect of drug administration on threshold changes. The between-subject contrasts did not show significance (F1,16 = 0.475; p = 0.501) but the group × condition interaction was significant (F1,16 = 7.283; p = 0.016). Bonferroni's corrected post-hoc two-tailed t tests revealed no significant differences between the lowHalo and lowAmph conditions. Although no difference between the QNP and control group was detected for the highHalo condition, there was a significant difference between the groups in the highAmph condition (t16 = 4.602, p < 0.001), showing that in QNP-treated rats the reward-facilitating effects of d-amphetamine were enhanced in comparison to control rats.
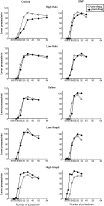
Rate-frequency (R/F) functions taken from two representative animals. R/F functions for the five intracranial self-stimulation tests from one control and one quinpirole (QNP)-treated animal. Each plot represents data from one test session under pre-drug (open circles) and post-drug (filled circles) condition. R/F functions were obtained by logarithmically changing the frequency of the stimulation pulses in 0.4 s stimulation train from a value that sustained maximum lever pressing to one that failed to sustain lever pressing. Each data point represents the mean of four trials. Both animals demonstrate a curve-shift to the left after high amphetamine (highAmph) treatment and to the right after an effective dose of haloperidol (highHalo). The application of haloperidol or d-amphetamine at a low dose had only minor effects on stimulation thresholds.

Changes in intracranial self-stimulation (ICSS) threshold for quinpirole (QNP)-treated rats and controls. Changes in ICSS thresholds (expressed as percentage of pre-drug values) following the injection of 0.05 mg/kg haloperidol (highHalo), 0.01 mg/kg haloperidol (lowHalo), saline, 0.1 mg/kg d-amphetamine (lowAmph) or 0.5 mg/kg d-amphetamine (highAmph). (a) Animals from both groups (QNP: n = 9; control: n = 9) demonstrate slight increases of stimulation thresholds after lowHalo, saline and even lowAmph treatment, which is most probably due to high activity in the time preceding the ICSS tests. Consequently, threshold shifts were normalized for each rat's individual shift in the saline condition. (b) QNP-treated animals demonstrate a stronger response to an effective dose of d-amphetamine than controls. Error bars represent s.e.m.
Testing for effects of drug administration on the asymptotes of the R/F functions revealed significance in the within-subject contrasts (F4,16 = 7.630; p = 0.014) and the between-subject contrasts (F1,16 = 8150.192; p < 0.001). However, Bonferroni's corrected post-hoc t tests failed to show significant differences in any of the pairwise comparisons. When asymptote changes were corrected for the individual saline condition (as done for the threshold shifts) a 2 × 4 mixed-design ANOVA revealed significance in the within-subject contrasts (F3,16 = 8.193; p = 0.011) while Bonferroni's corrected t tests did not reveal any significant effects.
Discussion
This study was designed to investigate the involvement of dopaminergic dysregulation in the reward circuitry of rats in the QNP model of OCD. Animals were acutely challenged in an ICSS experiment with d-amphetamine and haloperidol, i.e. drugs affecting dopaminergic neurotransmission. Our major finding is that the reward-facilitating effects of an effective dose of d-amphetamine (0.5 mg/kg) were enhanced in QNP-treated rats compared to controls. This suggests that the chronic QNP treatment induces changes within the reward circuitry. To the best of our knowledge, this is an observation that has not previously been reported.
Phenotype induction and data analysis
As has previously been shown, chronic-intermittent administration of QNP led to the emergence of compulsive checking in rats. QNP-treated rats revisited one locale on a modified open field excessively often and rapidly compared to saline-treated controls. Compulsive checking was stable after the 10th injection of QNP until the end of the experimental procedure. In contrast, QNP-induced locomotor sensitization further increased with continued QNP administration.
When tested in the ICSS procedure, animals demonstrated an unexpected rightward shift of their R/F function after injection of saline and even after a low dose of d-amphetamine. This shift was slightly higher in QNP-treated animals than in controls (see Fig. 4). Although self-stimulation is relatively unaffected by external confounders, our observations and other reports (Carlezon & Chartoff, 2007) confirm that fatigued animals occasionally do not respond to normally efficacious frequencies. In our design, the ICSS tests were performed 4 h after the QNP/saline injection and the open field test. QNP-treated animals were significantly more active during this time, as demonstrated by their increased locomotion. Therefore, it is possible that the observed shift is due to the fatiguing activity before the ICSS test. We believe that this is a confounder intrinsic to the design of the study. Consequently, the threshold changes for d-amphetamine and haloperidol were normalized for the changes in the saline condition. Due to a relatively long half-life time of QNP we cannot exclude having tested under declining QNP plasma levels. However, baseline thresholds were not affected by the QNP injection, making residual QNP unlikely to majorly affect the reported findings.
Enhanced response to d-amphetamine
We tested two dosages (high, low) of d-amphetamine and haloperidol. The higher dosage was chosen based on its reported effectiveness to shift the ICSS R/F function; the lower dosage was added in order to detect potential hypersensitivity to the drugs (Grilly & Loveland, 2001). Administration of the higher dosage of haloperidol (0.05 mg/kg) induced a substantial increase of ICSS thresholds in both animal groups, while asymptotes were not significantly affected. This rightward shift of the R/F function can be attributed to the well-known D2 antagonist properties of haloperidol, which have been related to the attenuation of brain reward and induction of anhedonia (Wise, 2008). No significant differences between the QNP group and the control group were detected for this drug treatment.
Administration of the higher dosage of d-amphetamine (0.5 mg/kg) induced a leftward curve-shift in both groups, while maximal response rates were not significantly affected. The leftward shift of the R/F function can be attributed to the increased dopaminergic neurotransmission induced by d-amphetamine, which has been related to a facilitation of brain stimulation reward (Grilly, 2001; Wise, 2008). Interestingly, the threshold change was significantly enhanced in the QNP group compared to the control group. This effect indicates that QNP treatment induced chronic changes and possibly neuroadaptation in the reward circuitry. As we observed a reward facilitation, it remains a subject for future research to integrate this finding with the view that OCD involves reduced reward processes associated with goal attainment.
The reward systems and OCD
In this study we detected differences in dopaminergic reward processing in QNP-treated rats in an ICSS experiment. A single ICSS study cannot give conclusive answers with regard to the locus and mechanism underlying the observed behavioural measure as the effects of brain stimulation reward are trans-synaptically transmitted (Wise, 2002). However, neuroadaptation within the dopaminergic signalling pathways of the nucleus accumbens (NAcc) can be speculated as an underlying mechanism. The NAcc acts as the interface between motivational drive and subsequent motor acts. Repetitive behaviours – as seen in OCD – are typically complex behaviours and their development requires the translation of motivationally relevant stimuli into adaptive motor responses. Further, the summation of d-amphetamine reward with brain stimulation reward is thought to primarily occur in the NAcc (Wise, 1996, 2002; Yeomans et al.1985).
Intermittent QNP-treatment over a longer period was previously indicated to induce a D2-autoreceptor desensitization in dopaminergic terminals of the mesolimbic system (Cluver et al.2008; Lomanowska et al.2004; Muscat et al.1993). Our data suggest that pre- rather than post-synaptic D2 receptors mediate the observed effect of QNP treatment. As d-amphetamine acts as a dopamine releaser, it affects pre- and post-synaptic signalling, where desensitized presynaptic D2 autoreceptors might enhance dopamine release. Haloperidol in contrast only acts on the post-synaptic level, which might explain why the animal's response to haloperidol is not altered.
Interestingly, the hypothesis of a crucial involvement of the NAcc in the pathophysiology of OCD is also supported by clinical evidence (Cohen et al.2012; Jung et al.2011; Palminteri et al.2012). The NAcc has been repeatedly hypothesized to play a key role in repetitive and compulsive behaviour and has been used as a target for therapeutic deep brain stimulation in OCD and Tourette's syndrome patients (Brito, 1997; Denys et al.2004; Sturm et al.2003). A recent fMRI study by Figee et al. (2011), which reported differences in NAcc activity during reward processing in OCD patients, further supports this hypothesis.
In addition to these clinical findings, recent animal studies have also indicated the critical involvement of NAcc in compulsive checking in rats. Dvorkin et al. (2010) examined the effects of neurotoxic lesions to the NAcc core on compulsive checking in the QNP model. They found that lesions to the NAcc core increased checking behaviour in control animals to a level almost as high as that of QNP-treated rats. Mundt et al. (2009) assessed the effects of high-frequency stimulation of the NAcc shell and core in a study that did not find increased checking behaviour in control rats during high-frequency stimulation. Stimulation of the NAcc core and shell was even seen to reduce compulsive behaviour in QNP-treated animals. Such an anti-compulsive effect of NAcc high-frequency stimulation has also been reported for rats with schedule-induced polyipsia (van Kuyck et al.2008).
In summary, our data support the hypothesis that chronic QNP treatment of rats induces dysregulation within the reward system. These changes in reward processing of animals with compulsive checking behaviour may be related to NAcc function. Clinical evidence suggests that these changes in reward processing are not simply a by-product of the QNP treatment but rather a crucial factor for the emergence of OCD symptoms.
Supplementary material
For supplementary material accompanying this paper, visit http://dx.doi.org/10.1017/S1461145712000983
Supplementary information supplied by authors.
Acknowledgements
We thank R. Winter, J. Hellweg and C. Kölske for their technical assistance. The study was supported by Fresenius grant (Else Kröner-Fresenius Grant 2008_A132).
Statement of Interest
None.
References