-
PDF
- Split View
-
Views
-
Cite
Cite
Sanjay Kumar Dwivedi, Naveen Chandra, Arun Pratap Mishra, Sonam Bahuguna, Aman Srivastava, Dhani Arya, Gajendra Singh, Bojan Đurin, Nikola Kranjčić, Raj Singh, Assessing the role of Alnus nepalensis D. Don in stabilizing landslide and its potential distribution: a case study from Uttarakhand, Western Himalaya, Integrated Environmental Assessment and Management, Volume 21, Issue 1, January 2025, Pages 105–116, https://doi.org/10.1093/inteam/vjae002
- Share Icon Share
Abstract
The rising frequency and severity of landslides in the vulnerable Himalayan region of India threaten human settlements and critical infrastructure. This growing issue demands urgent action and innovative strategies to mitigate risks and bolster the resilience of affected communities and infrastructure in this fragile area. The research explores the use of Alnus nepalensis for slope stabilization, illustrated by a case study near Ukhimath, Uttarakhand, India, and elucidates the potential ecological niche of Alnus in the temperate region of Uttarakhand using well-dispersed species occurrence records along with environment. The study used Sentinel-2 (2021) data for land use and land cover (LULC) mapping and Landsat 4-5, Sentinel-2, and Google Earth imagery from 1998, 2004, 2015, 2019, and 2020 for landslide slope recovery assessment. The Ukhimath landslide, spanning 54.61 ha in August 1998, demonstrated a remarkable recovery, with 98.20% coverage of Alnus by 2020, showcasing the species efficacy in stabilizing slopes without human intervention. The research forecasts a total potential distribution area of Alnus in the temperate region (1,000–2,500 m·asl [above mean sea level]) of Uttarakhand as ∼7,833 km2, with 782.30 km2 highly suitable, 2,104.33 km2 moderately suitable, and the rest showing low suitability. These distribution insights provide a foundation for in situ planning to leverage Alnus-based bioengineering for early slope stabilization, which is especially relevant in landslide-prone areas like Uttarakhand. The study provides a comprehensive and scientifically rigorous strategy for achieving sustainable outcomes in landslide-prone areas, particularly in the lower temperate region of the Himalaya.
Introduction
Ecologists and researchers are inherently intrigued by the mechanisms governing the distribution of species in specific geographical areas and the factors that restrict their occurrence elsewhere (Bennet, 1872; Grinnell, 1917; Connell, 1961). To unravel this complexity, scientists delve into a species’ geographical range. This range is intricately shaped by the dynamic interplay between the inherent traits of the species and the multitude of environmental factors that exert influence. The geographic range serves as a window into the intricate relationships between the biological attributes of a species and the environmental conditions that either foster or hinder its proliferation (Brown et al., 1996; Qazi et al., 2022). Investigating these patterns provides valuable insights into the intricate ecological dynamics and evolutionary processes that govern the spatial distribution of life forms.
Alnus nepalensis (known as alder or locally as Utis or Utas in western Himalaya) is a species that attracts researchers due to its ability to thrive on eroded surfaces, promoting slope stability. This large deciduous tree species has silver-grey bark and is found across the Himalayan region between 600 and 3,000 m above mean sea level (Sharma, 2012; Joshi & Garkoti, 2021). The alder tree has a consistent shape with a gradual decrease in diameter and is predominantly monopodial with a few branched stems. It usually grows to a height of 35 m with a clear trunk. Leaves are 7–16 cm long and 5–10 cm wide, alternately having a simple shape with shallow teeth. Leaf veins are easily visible and parallel (Jackson et al., 1994; Sharma, 2012). The alder thrives well in extremely wet environments; however, deep volcanic loamy soil conditions are best for alder’s growth. It may also grow on clay, sand, and gravel. Alders grow luxuriantly near streams and sites where a permanent and continuous water supply is available, particularly in deeply shaded ravines. Alder occur in narrow strips throughout the areas, especially along small drainages with poor ground vegetation represented by sparse growth of some grasses and ferns. They form a pure forest that stands on old landslide areas. However, the alder is sometimes associated with other tree species, such as Quercus leucotrichophora, Lyonia ovalifolia, Pinus roxburghii, Engelhardia spicata, Populus ciliata, Aeaculus indica, Ulmus wallichiana, Coriaria nepalensis, Debregeasia salicifolia, and Myrica esculenta. In India, several researchers have underlined the role of vegetation in slope stability, such as Khair (Acacia catechu), Gainthi (Boehemeria rugulosa), Panger (Aesculus indica), Mandar (Erythrina suberosa), Shisham (Dalbergia sisso), Chilkiya (Wendlandia puberula), willow (Salix tetrasperma), and Semal (Bombax ceiba) pointing out their far-reaching effects on the stability of slopes (Mukherjee, 2019; Rohela et al., 2020); however, the alder is the pioneer species that grows well in full light but also tolerates shade. It is situated in moist areas at lower altitudes but can colonize rocky areas exposed by landslides, road construction areas, and fallow fields.
Landslides are among the most common damaging natural geologic hazards in the hilly Himalayan landscape. The study of landslides has drawn global attention mainly due to expanding concern about the socioeconomic impacts of landslides (Kuniyal et al., 2021). High relief, weak tectonized, and heavily worn rocks, glacial debris, and anthropogenic activities like road building and step agriculture all contribute to frequent landslides in the Himalaya. Landslides are typically triggered by rain that is either lengthy or of high intensity. In the Himalaya region annually, landslides result in about 200 fatalities and losses of approximately $77 million U.S. (Chaturvedi et al., 2017). About 51% of the Uttarakhand region is located in areas with a high or very high risk of landslides (Gupta et al., 2022), resulting in several landslides in the recent past with a heavy loss of lives and infrastructure. Most of them have been rainfall-triggered landslides. Some significant tragic events are the Nainital city landslide in 1880, the Ukhimath landslides in 1979 and 1998 (former Chamoli, now Rudraprayag), and the Malpa landslide in 1998 in Pithoragarh, Uttarakhand (Rautela & Pande, 2005).
Several mechanical and traditional methods are used for restoring landslide sites. These include revegetation, retaining walls, terracing, drainage systems, slope grading, rock bolting, using geo-grids and geo-textiles, and bioengineering. Revegetation involves planting vegetation on the landslide site. Plants help stabilize the soil, prevent erosion, and reduce surface water runoff. The root systems of plants bind soil particles together, providing stability to the slope (Scotton & Andreatta, 2021). Construction of retaining walls involves erecting a structure to support and restrain soil on a slope. This prevents soil movement and reduces the risk of further landslides. The walls can be made from concrete, wood, or gabions (wire mesh containers filled with rocks) (Liu et al., 2023). There is also terracing, which involves creating a series of steps or platforms on a slope. This technique reduces the slope angle, minimizing the gravitational force acting on the soil. Terracing prevents soil erosion and controls water runoff (Mauri et al., 2022). Also, installing drainage systems helps manage water on the slope. Excess water can increase the weight of the soil, making it more prone to landslides. Proper drainage channels or pipes redirect water away from the slope, reducing the risk of instability (Srivastava & Chinnasamy, 2021; Zheng et al., 2021). In addition, the slope-grading method involves reshaping the slope by adjusting its gradient. The objective is to create a stable slope angle that minimizes the risk of landslides. Slope grading may include removing excess soil or redistributing it to achieve a safer configuration (Siddique et al., 2020). Rock bolts may also be used; rock bolts are inserted into the slope to reinforce and stabilize it. This technique is commonly used in rocky terrains. The bolts anchor unstable rock masses, preventing them from collapsing and triggering landslides (Pradhan & Siddique, 2020; Srivastava & Chinnasamy, 2023). Geogrids and geotextiles are synthetic materials placed within the soil to enhance its stability. Geogrids reinforce by improving soil structure, whereas geotextiles control erosion. Both contribute to landslide prevention by strengthening the soil (Kumar & Roy, 2022). Bioengineering involves using living plants and vegetation to stabilize slopes. Plant roots bind the soil, reducing erosion and adding cohesion. Bioengineering methods, such as planting specific species with robust root systems, contribute to long-term slope stability (Miele et al., 2021).
The primary objective of this work focuses on the Himalayan river valleys, characterized by unstable slopes and high precipitation, as potential habitats for Alnus nepalensis, a species recognized for its pioneer role in stabilizing landslide-prone slopes. Alnus is a nonleguminous nitrogen-fixing plant and has the remarkable ability to rapidly establish root systems that effectively bind soil, fast growth, and proficiency in nitrogen fixation that enriches the soil and promotes the growth of other vegetation. Its tolerance to a wide range of environmental conditions makes it an ideal pioneer species for natural recovery and long-term ecological stability. This study aims to evaluate the potential of A. nepalensis for slope stabilization and estimate its overall distribution within the region. This nuanced approach provides a comprehensive understanding of how this plant species relates to and potentially influences slope stability in areas susceptible to landslides. The work explicitly aims to bridge existing gaps in knowledge by offering management insights. There are some innovative aspects that significantly contribute to the understanding and application of A. nepalensis in slope stabilization and ecological restoration. First, the study provides a systematic evaluation of the species’ effectiveness in preventing landslides through root reinforcement and soil stabilization, offering empirical evidence of its capability to enhance slope stability in the fragile Himalayan region. Second, the study utilizes advanced geospatial techniques, including remote sensing and the MaxEnt machine learning algorithm, to predict the potential distribution of A. nepalensis across the region. By integrating field data with sophisticated analytical methods, the research identifies areas of high suitability for the species, guiding targeted restoration efforts and informing policy decisions. Additionally, this work addresses a significant gap in the literature by providing comprehensive estimates of the potential distribution of A. nepalensis, highlighting its ecological and socioeconomic benefits for the study area.
By focusing on the utility of A. nepalensis in slope stabilization, especially in a real-world case study near the town of Ukhimath, the study strives to provide practical implications for authorities involved in hazard mitigation planning and management. Including a detailed case study in Ukhimath town adds a layer of practicality and applicability to the research. This on-the-ground validation assesses the feasibility and effectiveness of using A. nepalensis for slope stabilization in a real-world scenario. The investigation goes beyond mere distribution mapping by delving into the potential habitat suitability of A. nepalensis across the temperate region of Uttarakhand.
Study area
The geographical extent of the study stretches between 1,000 to 2,500 m above mean sea level and has a temperate climate zone (Figure 1). The study region, which consists of low- to medium-grade crystalline rocks with intrusive acidic and basic rocks, is located in the lesser Himalaya beneath the center of the Himalayan zone (Mukherjee, 2019). The rock types in this region include granite gneiss, migmatite, argentiferous mica schist, porphyritic gneiss, tremolite-actinolite schist, calc-sericite schist, schistose quartzite, marble, and amphibolite. The area’s topography and landform are controlled by two major factors: bedrock structure and climatic changes. Due to the continuous tectonic movement of the crystalline and active denudation processes, these mountain ranges have become unstable. The lower midelevation ranges (1,200–1,800 m) representing the outer (Lesser) Himalayas vary considerably in forest formations and general physiognomy. Major vegetation types at these altitudes include Chir pine on south-facing slopes often exposed to fire, broadleaf forests of oak–rhododendron (Quercus leucotrichophora–Rhododendron arboreum), hillside grasslands, and secondary scrub. The higher elevation ranges (1,800–2,500 m asl [above mean sea level]) support temperate broadleaf forests, both evergreen and deciduous types. Oaks and Lauraceous species dominate evergreen forests. Key species include Moru oak (Q. floribunda) and Kharsu oak (Q. semecarpifolia). Common tree species at these elevations are Aesculus indica, Alnus nepalensis, Betula alnoides, Acer caesium, A. cappadocicum, Carpinus viminea, Juglans regia, Fraxinus micrantha, Corylus jacquemontii, Cornus macrophylla, and Euonymus fimbriatus, to name a few.
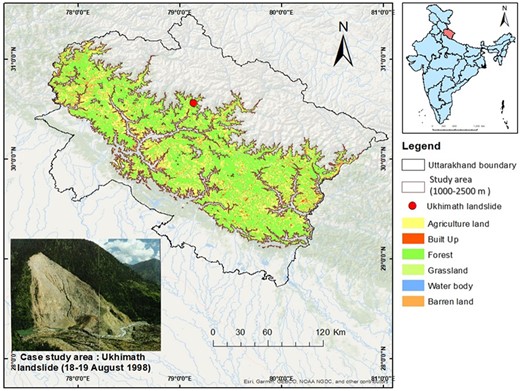
Map depicting the extent of the present study across Uttarakhand.
The landslide catastrophe near Ukhimath (Kothi-Benti) in 1998 was taken under investigation for a case study of Alnus nepalensis D. Don as a landslide stabilizer. The selection of this landslide catastrophe can be attributed to several key factors. A significant landslide catastrophe in 1998 suggests the site has a history of such events. The historical context provides valuable insights into the area’s vulnerability to landslides, making it an ideal case for studying landslide stabilization measures. Choosing a site with a documented history of landslides allows researchers to assess the real-world applicability and effectiveness of Alnus nepalensis in stabilizing landslide-prone slopes. The practical implications derived from a case study can offer valuable information for hazard mitigation planning. The two major thrusts, the Vaikrita Thrust and the Main Central Thrust, highlight the geological complexity of the region (Naithani et al., 2002). The interaction of geological factors is crucial in landslide occurrence, making this site geologically interesting for study. Ukhimath, situated in the Himalayan region, represents the challenging terrain characterized by steep slopes and landslide susceptibility. Findings from this case study can potentially be extrapolated to similar areas in the Himalaya, contributing to a broader understanding of landslide mitigation strategies.
Materials and methods
Data acquisition
Species presence data for A. nepalensis was obtained through an extensive survey conducted across various regions of Uttarakhand. The data collection involved comprehensive field surveys in major river valleys such as Bhagirathi, Alakananda, Ramganga, Gori, Mandakini, Kosi, and Dhauliganga. These surveys were complemented by expert knowledge-based sampling, leveraging tools like Google Earth Pro to identify and validate the presence of A. nepalensis. This combination of on-ground field surveys and remote sensing ensured a robust and accurate dataset, crucial for the subsequent habitat suitability modeling and analysis. A total of 94 presence locations of A. nepalensis were collected (Figure 2, See online supplementary material Table S1). The Sentinel-2 (2021) data were used to prepare the land use and land cover (LULC) of the study area and Landsat 4-5, Sentinel-2, and Google Earth images from the years 1998, 2004, 2019, 2015, and 2020 were used to conduct the landslide slope recovery assessment.
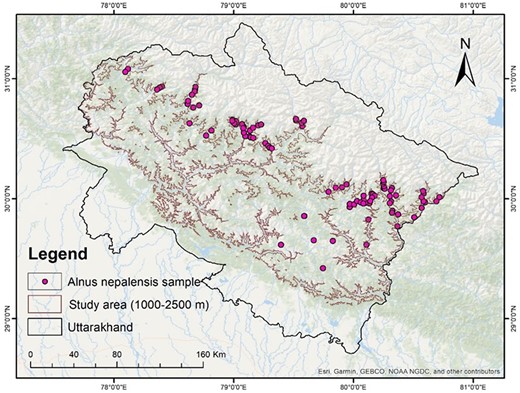
Topographic parameters were typically used to some extent when developing predictive models for mountainous terrain, with the digital elevation model serving as the primary requirement. The WorldClim Digital Elevation Model (DEM) downloaded at spatial resolution of 1 km was used to create the topographic variables slope, aspects, and terrain roughness. The 21 bioclimatic layers for the current scenario (1950–2000) were downloaded from WorldClim (WorldClim, 2024) at the spatial resolution of 1 km (30 arc∼seconds).
Ukhimath landslide recovery mapping
Landslide mapping in the field is frequently a difficult task for the following reasons: (i) the size of the landslide, which is frequently too large to be observed in full in the field; (ii) the investigator's vantage point, which is frequently insufficient to see all parts of a landslide (e.g., the scarp, lateral edges, deposit, and toe) with equal detail; and (iii) the fact that past landslides are frequently covered with vegetation, either entirely or partially, or that they have been largely destroyed by additional landslides, erosion processes, and human activity such as agricultural and forestry operations (Bardi et al., 2014). Due to the local standpoint on the landslide scale and the fact that the landslide boundary is frequently unclear, it is challenging to precisely follow a landslide boundary in the field due to the reduced visibility of the slope collapse. Therefore, viewing the landslide from a distance is preferable because it can lead to more precise and thorough mapping (Bardi et al., 2014).
In recent years, it has become apparent that geographic information systems (GIS) and remote sensing (RS) are essential components of landslide investigations (Pandey et al., 2023). Understanding the connections between the distribution of the landslides and the underlying causes requires mapping the existing landslides. Because field surveys in rough, mountainous terrain are not feasible for mapping every single landslide, a thorough mapping of landslides in GIS using RS image interpretation, assisted by field verifications at sampling places, is a realistic alternative. Landslide information extracted from satellite images with high spatial resolution successfully maps existing landslides. Satellite imagery’s ability to detect slides relies heavily on spectral properties, size, shape, contrast, and morphological expression. Landslides and the surrounding region generally have a clear spectral contrast (Sarkar & Kanungo, 2017).
The landslide and its recovery by vegetation in the Ukhimath area were mapped using Landsat 4-5 (1998 and 2010), Google Earth (2004), and Sentinel-2 (2015 and 2020) imagery by implying visual interpretation and digitization techniques in Arc GIS 10.5. Various field photographs were collected for the years 1998 (Das et al., 2023), 2012, and 2021. The observations of 60 residents (40 males, 20 females) were also recorded, followed by a detailed survey in the landslide zone in 2012, 2013, and 2021 by the authors. The present study adhered to qualitative investigation techniques as outlined in the work of (Chinnasamy & Srivastava, 2021).
Environmental and bioclimatic data
The study used 12 predictive variables: seven bioclimatic, four topographic, and LULC, to simulate the potential distribution. Given their contribution and significance in the model calibration and collinearity between variables, jackknife analysis, and biological relevance to the focal species, the study chose significant variables for modeling. One of the two strongly correlated variables (Pearson correlation coefficient, r > 0.80) was selected to be included in the model due to its biological significance to the species and simplicity of interpretation (Kumar & Stohlgren, 2009; Rana et al., 2018). Finally, a subset of the factors with the lowest correlation was chosen as predictor variables (Table 1).
Category . | Variables name . | Resolution . | Source . |
---|---|---|---|
Thematic | Land use land cover, 2021 | 10 m resampled to 30 arcs ∼ sec | Sentinel 2A |
Topographic | Slope | 30 arc ∼ sec | Derived from WorldClim DEM |
Aspect (northness) | 30 arc ∼ sec | Derived from WorldClim DEM | |
Aspect (eastness) | 30 arc ∼ sec | Derived from WorldClim DEM | |
Terrain roughness | 30 arc ∼ sec | WorldClim data | |
Climatic | Isothermality (bio3) | 30 arc ∼ sec | WorldClim data |
Temperature seasonality (bio4) | 30 arc ∼ sec | WorldClim data | |
Min temperature of the coldest month (bio6) | 30 arc ∼ sec | WorldClim data | |
The mean temperature of the driest quarter (bio9) | 30 arc ∼ sec | WorldClim data | |
Precipitation seasonality (bio15) | 30 arc ∼ sec | WorldClim data | |
Precipitation of driest quarter (bio17) | 30 arc ∼ sec | WorldClim data | |
Precipitation of warmest quarter (bio18) | 30 arc ∼ sec | WorldClim data |
Category . | Variables name . | Resolution . | Source . |
---|---|---|---|
Thematic | Land use land cover, 2021 | 10 m resampled to 30 arcs ∼ sec | Sentinel 2A |
Topographic | Slope | 30 arc ∼ sec | Derived from WorldClim DEM |
Aspect (northness) | 30 arc ∼ sec | Derived from WorldClim DEM | |
Aspect (eastness) | 30 arc ∼ sec | Derived from WorldClim DEM | |
Terrain roughness | 30 arc ∼ sec | WorldClim data | |
Climatic | Isothermality (bio3) | 30 arc ∼ sec | WorldClim data |
Temperature seasonality (bio4) | 30 arc ∼ sec | WorldClim data | |
Min temperature of the coldest month (bio6) | 30 arc ∼ sec | WorldClim data | |
The mean temperature of the driest quarter (bio9) | 30 arc ∼ sec | WorldClim data | |
Precipitation seasonality (bio15) | 30 arc ∼ sec | WorldClim data | |
Precipitation of driest quarter (bio17) | 30 arc ∼ sec | WorldClim data | |
Precipitation of warmest quarter (bio18) | 30 arc ∼ sec | WorldClim data |
Abbreviation: DEM = Digital Elevation Model
Category . | Variables name . | Resolution . | Source . |
---|---|---|---|
Thematic | Land use land cover, 2021 | 10 m resampled to 30 arcs ∼ sec | Sentinel 2A |
Topographic | Slope | 30 arc ∼ sec | Derived from WorldClim DEM |
Aspect (northness) | 30 arc ∼ sec | Derived from WorldClim DEM | |
Aspect (eastness) | 30 arc ∼ sec | Derived from WorldClim DEM | |
Terrain roughness | 30 arc ∼ sec | WorldClim data | |
Climatic | Isothermality (bio3) | 30 arc ∼ sec | WorldClim data |
Temperature seasonality (bio4) | 30 arc ∼ sec | WorldClim data | |
Min temperature of the coldest month (bio6) | 30 arc ∼ sec | WorldClim data | |
The mean temperature of the driest quarter (bio9) | 30 arc ∼ sec | WorldClim data | |
Precipitation seasonality (bio15) | 30 arc ∼ sec | WorldClim data | |
Precipitation of driest quarter (bio17) | 30 arc ∼ sec | WorldClim data | |
Precipitation of warmest quarter (bio18) | 30 arc ∼ sec | WorldClim data |
Category . | Variables name . | Resolution . | Source . |
---|---|---|---|
Thematic | Land use land cover, 2021 | 10 m resampled to 30 arcs ∼ sec | Sentinel 2A |
Topographic | Slope | 30 arc ∼ sec | Derived from WorldClim DEM |
Aspect (northness) | 30 arc ∼ sec | Derived from WorldClim DEM | |
Aspect (eastness) | 30 arc ∼ sec | Derived from WorldClim DEM | |
Terrain roughness | 30 arc ∼ sec | WorldClim data | |
Climatic | Isothermality (bio3) | 30 arc ∼ sec | WorldClim data |
Temperature seasonality (bio4) | 30 arc ∼ sec | WorldClim data | |
Min temperature of the coldest month (bio6) | 30 arc ∼ sec | WorldClim data | |
The mean temperature of the driest quarter (bio9) | 30 arc ∼ sec | WorldClim data | |
Precipitation seasonality (bio15) | 30 arc ∼ sec | WorldClim data | |
Precipitation of driest quarter (bio17) | 30 arc ∼ sec | WorldClim data | |
Precipitation of warmest quarter (bio18) | 30 arc ∼ sec | WorldClim data |
Abbreviation: DEM = Digital Elevation Model
Modeling approach
The model was built using the maximum entropy modeling (MaxEnt) machine learning technique. The jackknife test was used to determine the relative importance of each predictor variable (Phillips et al., 2006). The predictive model also enabled the development of duplicated runs for cross-ratification, bootstrapping, and repeated subsampling. The regularization factor was chosen at 0.1 to avoid overprediction and lessen model overfitting (Phillips et al., 2006). Therefore, the present data were randomly split into training and test sets in the proportion of 75:25 while keeping the other values as defaults (Bell & Fielding, 1997). According to Pearson et al., (2007), a jackknife test entails predicting a single location not included in the “training” dataset. By using receiver operating characteristic (ROC) analysis, accurate skill statistics, Kappa statistics, sensitivity (actual positive rate), and false alarm rate (1-specificity), the model’s predictive ability was assessed. The area under the curve (AUC) number was another way to judge how reliable the model was (Rane et al., 2023). The AUC displays a graph of varying index values, typically between a maximum value of 1 or 100% and a minimum value of 0.5 or 50% (Rane et al., 2023). The AUC value of 0.9 shows especially good model performance, 0.8 to 0.9 as good model performance, 0.7 to 0.8 as reasonable model classification, and 0.6 as poor model performance; thus, 0.6 is the recommended minimum limit. A higher AUC value indicates greater accuracy and influence of the factors (Silalahi et al., 2019; Dwivedi et al., 2022).
Results and discussion
In Uttarakhand, western Himalaya, high rainfall in combination with fragile geology creates high risk of landslides, especially during monsoon seasons, which further exacerbates the problem by accelerating soil erosion and runoff. Mitigating slope failures is crucial to prevent loss of life and economic losses. This study investigated the effectiveness of A. nepalensis for bioengineering applications in slope stabilization efforts in the temperate region by analyzing the Ukhimath landslide.
Ukhimath landslide (1998) and its natural recovery during 1998–2020
In August 1998, a landslide of about 54.61 ha occurred about 10 km from Ukhimath town (30°34'1.72'' N, 79°8'19.21'' E) in the Rudraprayag district of Uttarakhand, western Himalaya (Bist & Sah, 1999). The Kothi mountain slope failed, taking with it Kothi and Bhenti villages and depositing the debris on the other side of the Amrit Ganga River (originating from Madhmaheshwar), damaging the entire Poundar village. The landslide occurred in the main central thrust zone, characterized by continued minor and periodic major seismic events, highly crushed and pulverized rock fans, and cones of loose debris on steep slope seepage. The landslide was triggered by continuous excessive heavy rainfall in the region.
The observed elevation range of slide terrain was 1,300 to 2,000 m and had varying slope angles. The findings revealed a substantial area of approximately 28.35 ha, constituting 51.91% of the total, exhibiting significant revegetation, predominantly attributed by A. nepalensis. Moreover, the recuperated vegetation area in 2010 amounted to 36.93 ha (67.62%), and by 2015, it had expanded to 47.51 ha (86.99%). The unstable slope significantly recovered, reaching 98.20% (53.63 ha) by 2020. A. nepalensis showed an extraordinary capability to settle unstable slopes (see Figures 3, 4, and Table 2), as the gentler slopes were revegetated within 5–6 years.
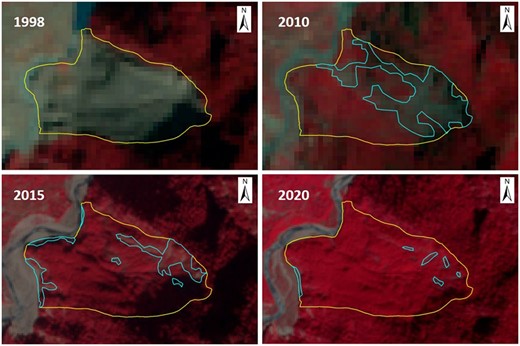
Vegetation recovered in the Ukhimath (Kothi-Bhenti) landslide (1998–2020).

Field photographs of the Ukhimath landslide depicting complete slope stabilization (revegetated) by Alnus nepalensis within 23 years.
Years . | Landslide area (with no vegetation cover) (haa) . | Recovered area (vegetation) (ha) . | Recovered (%) . | Data used . |
---|---|---|---|---|
1998 | 54.61 | 0 | 0 | Landsat 4-5 |
2004 | 26.26 | 28.35 | 51.91 | Google Earth |
2010 | 17.68 | 36.93 | 67.62 | Landsat 4-5 |
2015 | 7.10 | 47.51 | 86.99 | Sentinel-2 |
2020 | 0.98 | 53.63 | 98.20 | Sentinel-2 |
Years . | Landslide area (with no vegetation cover) (haa) . | Recovered area (vegetation) (ha) . | Recovered (%) . | Data used . |
---|---|---|---|---|
1998 | 54.61 | 0 | 0 | Landsat 4-5 |
2004 | 26.26 | 28.35 | 51.91 | Google Earth |
2010 | 17.68 | 36.93 | 67.62 | Landsat 4-5 |
2015 | 7.10 | 47.51 | 86.99 | Sentinel-2 |
2020 | 0.98 | 53.63 | 98.20 | Sentinel-2 |
Note. ha = hectare.
Years . | Landslide area (with no vegetation cover) (haa) . | Recovered area (vegetation) (ha) . | Recovered (%) . | Data used . |
---|---|---|---|---|
1998 | 54.61 | 0 | 0 | Landsat 4-5 |
2004 | 26.26 | 28.35 | 51.91 | Google Earth |
2010 | 17.68 | 36.93 | 67.62 | Landsat 4-5 |
2015 | 7.10 | 47.51 | 86.99 | Sentinel-2 |
2020 | 0.98 | 53.63 | 98.20 | Sentinel-2 |
Years . | Landslide area (with no vegetation cover) (haa) . | Recovered area (vegetation) (ha) . | Recovered (%) . | Data used . |
---|---|---|---|---|
1998 | 54.61 | 0 | 0 | Landsat 4-5 |
2004 | 26.26 | 28.35 | 51.91 | Google Earth |
2010 | 17.68 | 36.93 | 67.62 | Landsat 4-5 |
2015 | 7.10 | 47.51 | 86.99 | Sentinel-2 |
2020 | 0.98 | 53.63 | 98.20 | Sentinel-2 |
Note. ha = hectare.
In addition to stabilizing hill slopes, the role of alder as a pioneer species extends beyond its individual growth and survival. Leveraging its adaptation capabilities, rapid growth, nitrogen-fixing ability, and soil improvement effects, it plays a pivotal role in the broader restoration and development of ecosystems by creating conditions conducive to the growth of diverse plant species. This process is essential to ecological succession, where pioneer species pave the way for more complex and diverse communities to thrive.
Globally, Alnus nepalensis is recognized for its ability to flourish on precarious slopes in a variety of mountainous locales, such as Tibet, Thailand, Vietnam, India, China, Nepal, and Bhutan (Shrestha et al., 1998; Zhengyi et al., 1999). Hence, the application of indicator species to identify and delineate the hill slope instability is discussed and proposed as a feasible ecological technique at local as well as global scales. Investigations in Uttarakhand, Himalaya, show that instability caused by road cutting and water erosion in 2000 (Barsu, Rudraprayag district) led to the colonization of the unstable slope by alder species in 2001. This taxon, growing at 145 cm/year, marked the unstable area by 2005. At the Bhanti site, multiple generations of indicator plants (aged 45, 20, and 1–3 years) correspond with landslide records, indicating phases of slope instability.
In Manipal, the presence of precursor taxa along seasonal water channels signals slope instability due to gully erosion during rains (Negi, 2013). The invasion of precursor species into potential hill slope movement areas is influenced by interdisciplinary factors and microclimatic conditions. The varying radiative energy balance on different slope aspects creates local air flows (Negi, 2002), aiding the dissemination of A. nepalensis seeds. These winged seeds are dispersed by air currents, including those from strong local and regional winds. In suitable habitats, the seeds germinate and grow, indicating slope instability. The species’ fast growth, nitrogen fixation, and ability to thrive in infertile, rocky soils contribute to its successful establishment on unstable slopes, making it relevant to the current study on slope movement dynamics. Soil bioengineering, leveraging the power of plant root systems, offers a sustainable and cost-effective solution for mitigating environmental hazards (landslides, floods) and promoting landscape restoration, especially in fragile ecosystems (Bahri, 2024). Planting grasses and shrubs on denuded slopes with exposed loose soil and waterlogged areas can help arrest surface erosion and enrich the soil with organic matter (Patial et al., 2022).
Grasses offer short-term surface erosion protection due to their fast growth and dense shallow roots provides resistance against surface erosion that is short lived, and grasses will struggle in dry seasons (Gray & Sotir, 1996; Dorairaj & Osman, 2021). However, woody vegetation offers a range of benefits for mass stability, including mechanically reinforcing and restraining the soil with its roots and stems while also modifying slope hydrology by drawing moisture from the ground through evapotranspiration (Ray & Choudhary, 2014). Alnus nepalensis, a pioneer woody species that thrives in landslide areas, demonstrates exceptional potential for use in bioengineering interventions to mitigate slope failures promoting a sustainable ecological solution.
Spatial extend of A. Nepalensis in Uttarakhand
Assessing the suitability of A. nepalensis in Uttarakhand’s lower temperate region yielded valuable insights into its habitat distribution. The analysis revealed distinct suitability levels across the state, classified into three categories: high suitability, moderate suitability, and low suitability (Figure 5, Table 3). Out of the total study area of 7833 km2, an approximately 782.30 km2 area of the state was identified as a highly suitable habitat for A. nepalensis. These areas offer ideal conditions for the species’ growth, indicating its compatibility with the prevailing environmental factors. The highly suitable area of the state for A. nepalensis also shows that these areas have high moisture regimes and are also prone to landslides. The moderate suitability zone encompassed approximately 2,104.33 km2 of the state, representing regions where the species could thrive under favorable conditions. This suggests that A. nepalensis could establish itself and contribute to ecosystem stability in these areas, albeit with some limitations. Conversely, the study identified approximately 4,437.52 km2 as having low suitability for A. nepalensis. These regions may present challenges or limitations that hinder the species’ successful establishment or growth. Along the river valleys, the climatic spaces for species were addressed. According to the baseline climatic scenario, 10% of the total area of the examined region would be appropriate for A. nepalensis growth. The species’ most significant and continuous territory is found in the fringes of Askote (along the Gori and Kali river valleys), along the Bhagirathi river valley (after Uttarkashi) and Kedarnath Wildlife Sanctuary (along the Mandakini, Madhu Ganga, and Balkhila river valleys). However, its distribution in the western region is patchy. Most frequently in the west and to some extent in the Siwalik range, there are a few isolated areas of various but generally small size.
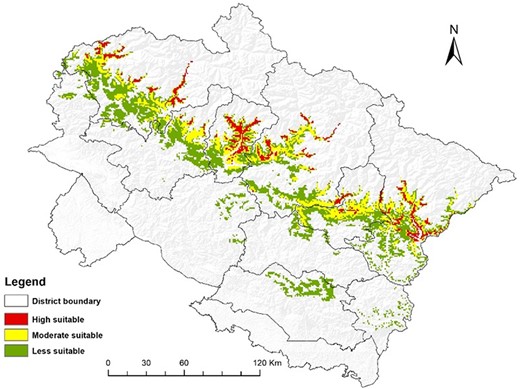
Potential distribution of Alnus nepalensis in Uttarakhand, western Himalaya.
Suitability degree . | Area (km2) . | Area (%) . |
---|---|---|
High | 782.30 | 10.68 |
Moderate | 2,104.33 | 28.73 |
Low | 4,437.52 | 60.58 |
Suitability degree . | Area (km2) . | Area (%) . |
---|---|---|
High | 782.30 | 10.68 |
Moderate | 2,104.33 | 28.73 |
Low | 4,437.52 | 60.58 |
Suitability degree . | Area (km2) . | Area (%) . |
---|---|---|
High | 782.30 | 10.68 |
Moderate | 2,104.33 | 28.73 |
Low | 4,437.52 | 60.58 |
Suitability degree . | Area (km2) . | Area (%) . |
---|---|---|
High | 782.30 | 10.68 |
Moderate | 2,104.33 | 28.73 |
Low | 4,437.52 | 60.58 |
Prioritizing highly suitable habitats (approximately 782.30 km2) is pivotal for quickly recovering landslide areas and biodiversity conservation and safeguarding both A. nepalensis and the diverse ecosystems it supports. Recognizing moderate suitability zones (roughly 2,104.33 km2) presents opportunities to promote A. nepalensis in regions where it can thrive, fostering ecosystem stability and resilience against environmental challenges. Highly suitable areas may coincide with landslide-prone regions, suggesting the strategic planting of A. nepalensis for the dual benefits of slope stabilization and enhanced habitat suitability. Identifying regions with low suitability (approximately 4,437.52 km2) informs land-use planning, minimizing conflicts between development initiatives and areas where the species may encounter growth challenges. The model’s prediction of unsuitability in the Terai and high mountains aligns with climatic scenarios, offering insights into how A. nepalensis might respond to climate change and guiding adaptive strategies. Concentrating conservation efforts in the fringes of Askote and Kedarnath Wildlife Sanctuary, with the species’ greatest territory, ensures targeted interventions in ecologically significant areas. Predictions highlighting areas where A. nepalensis is expected to thrive in the future guide conservation planning and habitat restoration initiatives, promoting sustained ecological health.
Model evaluation and variable analysis
The AUC of the ROC was used to assess the model’s performance. The sensitivity of a probabilistic occurrence forecast is plotted against the erratically projected positive proportion or commission error (1-specificity) for all feasible thresholds, yielding the ROC. In the specific case of the study’s habitat suitability map for A. nepalensis, the AUC values were 0.898 for the training data and 0.893 for the test data (Figure 6A). The elevated AUC values suggest that the habitat suitability model for A. nepalensis is reliable and accurate. Researchers can trust the model’s predictions, knowing that it performs well in distinguishing suitable and unsuitable areas for the species. Moreover, practitioners, such as conservationists and land managers, can use the habitat suitability map to identify areas likely to support A. nepalensis. This information (AUC values) is crucial for planning conservation efforts, habitat restoration projects, or targeted planting initiatives to enhance the species' presence.
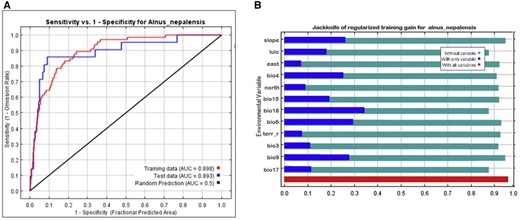
(A) Sensitivity versus 1-specificity. (B) Jackknife variable gains. AUC = area under the curve.
Sensitivity analysis
To determine which environmental factor is crucial for the probable distribution of Alnus species in Uttarakhand, the jackknife test (Figure 6B) was performed. Each modeling iteration generated 50 random distributions, facilitating the calculation of average profits with and without each variable. In the graphical representation, the red bar signifies the model’s overall performance, whereas the blue bar illustrates its performance solely based on underlying environmental variables. Conversely, the light blue bars depict the model’s performance without including relevant variables. Notably, the environmental variable bio18 emerges as a significant contributor to the model’s information content for A. nepalensis, as evidenced by its substantial gain when used independently (Figure 6B). Conversely, the variable bio17 demonstrates the highest information distinctiveness, indicating unique insights not captured by other variables, as its removal leads to the most significant reduction in gain. Additionally, bio6 emerges as the second-most crucial variable, with its absence from the complete model resulting in a noticeable decrease in average gain. Identifying crucial variables (bio18 and bio6) guides further investigations into the ecological factors influencing the habitat suitability of A. nepalensis. Practitioners involved in habitat management and restoration efforts can benefit from understanding the key environmental variables that affect the presence of A. nepalensis. Additionally, the findings contribute to evidence-based policymaking by providing a nuanced understanding of the ecological factors shaping the habitat suitability for A. nepalensis.
Percent contribution of the variables and factors influencing A. nepalensis distribution
Precipitation, temperature, and slope emerged as influential factors positively affecting the occurrence of Alnus species, with higher variable values corresponding to an increased likelihood of species presence. Among the environmental layers, slope, bio18, LULC, and bio6 played pivotal roles in delineating suitable habitats for Alnus species within the model. Conversely, other variables, including bio4, bio17, bio9, aspect, and bio15, exhibited comparatively lower gains, indicating their limited impact on predicting suitable habitat for the Alnus species (Table 4). Plant distributions may be influenced by the plant’s physiological climatic tolerance (Scotton & Andreatta, 2021). To determine the likelihood of A. nepalensis distribution and the suitability of its habitat, rainfall during the warmer and drier seasons of the year is crucial (Qazi et al., 2022). Himalayan alders exhibit survival in areas characterized by a precipitation range of 400 to 900 mm during the warmest periods of the year, as highlighted by Rana et al. (2018). This aligns with Sakalli’s (2013) findings, which specify that Alnus species are typically restricted to regions featuring an annual precipitation range of 500 to 1,100 mm and temperatures between 8°C and 15°C. Given that edaphic and biogeographic considerations that constrain them, habitat appropriateness may not always be a reliable indicator of species occurrence (Ranjitkar et al., 2014).
S.No. . | Variable . | Contribution (%) . |
---|---|---|
1 | Slope | 21.6 |
2 | Land use and land cover (LULC) | 15.4 |
3 | Temperature seasonality (bio4) | 2.2 |
4 | Aspect northness | 5.5 |
5 | Aspect eastness | 4.1 |
6 | Precipitation seasonality (bio15) | 5.2 |
7 | Precipitation of warmest quarter (bio18) | 21.8 |
8 | Minimum temperature of the coldest month (bio6) | 9.4 |
9 | Terrain roughness | 4.8 |
10 | Isothermality (bio3) | 2.5 |
11 | The mean temperature of the driest quarter (bio9) | 5.1 |
12 | Precipitation of driest quarter (bio17) | 2.4 |
S.No. . | Variable . | Contribution (%) . |
---|---|---|
1 | Slope | 21.6 |
2 | Land use and land cover (LULC) | 15.4 |
3 | Temperature seasonality (bio4) | 2.2 |
4 | Aspect northness | 5.5 |
5 | Aspect eastness | 4.1 |
6 | Precipitation seasonality (bio15) | 5.2 |
7 | Precipitation of warmest quarter (bio18) | 21.8 |
8 | Minimum temperature of the coldest month (bio6) | 9.4 |
9 | Terrain roughness | 4.8 |
10 | Isothermality (bio3) | 2.5 |
11 | The mean temperature of the driest quarter (bio9) | 5.1 |
12 | Precipitation of driest quarter (bio17) | 2.4 |
S.No. . | Variable . | Contribution (%) . |
---|---|---|
1 | Slope | 21.6 |
2 | Land use and land cover (LULC) | 15.4 |
3 | Temperature seasonality (bio4) | 2.2 |
4 | Aspect northness | 5.5 |
5 | Aspect eastness | 4.1 |
6 | Precipitation seasonality (bio15) | 5.2 |
7 | Precipitation of warmest quarter (bio18) | 21.8 |
8 | Minimum temperature of the coldest month (bio6) | 9.4 |
9 | Terrain roughness | 4.8 |
10 | Isothermality (bio3) | 2.5 |
11 | The mean temperature of the driest quarter (bio9) | 5.1 |
12 | Precipitation of driest quarter (bio17) | 2.4 |
S.No. . | Variable . | Contribution (%) . |
---|---|---|
1 | Slope | 21.6 |
2 | Land use and land cover (LULC) | 15.4 |
3 | Temperature seasonality (bio4) | 2.2 |
4 | Aspect northness | 5.5 |
5 | Aspect eastness | 4.1 |
6 | Precipitation seasonality (bio15) | 5.2 |
7 | Precipitation of warmest quarter (bio18) | 21.8 |
8 | Minimum temperature of the coldest month (bio6) | 9.4 |
9 | Terrain roughness | 4.8 |
10 | Isothermality (bio3) | 2.5 |
11 | The mean temperature of the driest quarter (bio9) | 5.1 |
12 | Precipitation of driest quarter (bio17) | 2.4 |
The A. nepalensis real range is susceptible to restriction due to factors such as seed dispersal mechanisms, colonization ability, and LULC changes; the majority of flooded and undisturbed areas consist of this range (Dwivedi et al., 2022). The important delimiting factor for its niche is climatic variables. The greatest determining factors encountered are slope, bio-18, and LULC. Besides these variables, Alnus trees are responsive to precipitation and temperature. When modeling large geographical areas, these climatic factors are essential for species occurrence (Pearson & Dawson, 2003; Ranjitkar et al., 2014). The predicted suitability areas were primarily found near riverine areas. In addition to climate, A. nepalensis seed distribution and establishment are impeded by high mountains (> 3,000 m amsl) in the northern aspect and increasing elevation (Rana et al., 2018).
Harnessing A. nepalensis for sustainable mountain ecosystems
In Uttarakhand, 10 out of 13 district headquarters, with approximately 40 municipal townships and hundreds of villages, where a significant hill population resides, fall within the study zone (1,000–2,500 m asl). With increasing climate variability and intensifying weather patterns, the state has become highly vulnerable to nature-induced calamities. Additionally, anthropogenic development activities continuously expand into areas prone to these calamities. Nature-based solutions, complemented by modern environmental engineering techniques, such as the plantation of Alnus on soil-eroded slopes and the use of soil-resistant nets, similar to the Dayara bugyal restoration model (Kuniyal et al., 2021), offer promising prospects for ecofriendly sustainability of mountain resources and ecosystems.
Apart from mechanical landslide management, A. nepalensis can be pivotal in the quick recovery of fresh and old landslide areas, especially within 1,000–2,500 m above sea level. Land managers and policymakers in Uttarakhand can utilize this species strategically for stabilizing road cuttings, using it as a road head plantation. The high and moderate suitability areas identified in this study present targeted restoration and habitat enhancement opportunities, particularly in regions prone to landslides or ecological degradation. By strategically introducing and nurturing A. nepalensis, these areas could benefit from the species’ natural stabilizing properties and its potential to facilitate ecosystem recovery. Moreover, the study’s distribution insights serve as the foundation for informed decision-making and proactive planning to mitigate the impact of landslides and promote ecological equilibrium. The suitability assessment underscores the value of A. nepalensis as a natural asset for slope stabilization and ecological restoration, highlighting its adaptability and potential contributions to enhancing soil quality and fostering plant growth in challenging environments.
Future considerations and management implications
Although this study provides valuable insights into the current suitable habitat for Alnus nepalensis, future research should incorporate climate change and land-use change scenarios to enhance its applicability for long-term bioengineering-based landslide management and policy-making. Climate change projections indicate potential alterations in temperature and precipitation patterns, which could significantly affect the distribution of suitable habitats for A. nepalensis. These changes may shift landslide risk zones, necessitating adjustments in mitigation strategies. Similarly, anticipated land-use changes, such as deforestation or infrastructure development, could fragment existing habitats or alter slope stability, underscoring the need for proactive land-use planning to minimize landslide risks. Integrating future scenarios into habitat suitability models would provide critical information for long-term planning and targeted interventions to safeguard vulnerable areas. In addition to climate and land-use change scenarios, future research could explore temporal dynamics, genetic diversity, and adaptation of A. nepalensis. Investigating the temporal dynamics of A. nepalensis growth and its impact on slope stabilization over different climatic periods could provide insights into the resilience and adaptability of this species under varying environmental conditions. Studying the genetic diversity of A. nepalensis populations across different regions could reveal adaptive traits that enhance its survival and effectiveness in slope stabilization, informing selective breeding programs for restoration projects. Examining the synergistic effects of A. nepalensis with other native species in mixed plantations could enhance ecosystem resilience and biodiversity, offering a more holistic approach to ecological restoration. Assessing the socioeconomic benefits of using A. nepalensis for slope stabilization, such as reduced infrastructure damage and improved livelihoods for local communities, could strengthen the case for its widespread adoption. Leveraging RS, GIS, and machine learning advancements to develop more accurate and dynamic habitat suitability models would improve predictive capabilities and inform real-time decision-making. Our findings provide a “need of the time” baseline for immediate management strategies and policy decisions regarding regional landslide mitigation. These initial insights can guide urgent interventions while laying the groundwork for more comprehensive future studies that incorporate evolving environmental and socio-economic factors.
Conclusion
The comprehensive investigation presented in this study addresses the critical issue of landslides in the fragile Himalayan region, explicitly focusing on utilizing A. nepalensis for slope stabilization and ecological restoration. The findings from the multifaceted exploration provide valuable insights and avenues for sustainable mountain ecosystem management. The following conclusions are drawn. First, the findings from the Ukhimath landslide in 1998 showcased the extraordinary capability of A. nepalensis to stabilize slipping and eroding slopes. Over the years, the species demonstrated rapid revegetation, reaching an imposing 98.20% coverage by 2020. This successful intervention highlights the species’ effectiveness in slope stabilization and emphasizes its role as a pioneer species fostering ecological succession. Second, the assessment of A. nepalensis habitat distribution in Uttarakhand’s lower temperate region revealed distinct suitability levels across the study area. The model predicted highly suitable areas, presenting opportunities for targeted conservation efforts, including slope stabilization in landslide-prone regions. Moderate suitability zones offer chances for promoting A. nepalensis in areas conducive to its growth, contributing to ecosystem stability. Third, the model’s robustness was validated through AUC analysis, signifying its reliability in distinguishing suitable and unsuitable areas for A. nepalensis. Furthermore, the sensitivity analysis identified vital environmental variables, guiding future investigations and providing practitioners with essential information for habitat management and restoration. Finally, the study identified precipitation, temperature, and slope as influential factors positively affecting the occurrence of Alnus species. Understanding the climatic tolerance and ecological factors shaping A. nepalensis habitat suitability is crucial for effective conservation planning and habitat restoration initiatives.
The study concludes by proposing practical applications of A. nepalensis for sustainable mountain ecosystems in Uttarakhand. Nature-based solutions, including strategic plantation on soil-eroded slopes, can complement traditional landslide management techniques. The identified high and moderate suitability areas offer targeted opportunities for restoration and habitat enhancement, supporting the natural stabilizing properties of A. nepalensis and promoting ecological equilibrium. This research provides a holistic understanding of the potential of A. nepalensis to mitigate landslide risks, enhance ecosystem resilience, and foster sustainable mountain ecosystems. The insights generated contribute to evidence-based decision-making for conservation, habitat restoration, and proactive planning in landslide-prone regions, promoting long-term ecological health and community well-being.
Supplementary material
Supplementary material is available online at Integrated Environmental Assessment and Management.
Data availability
Data, associated metadata, and calculation tools are available from the corresponding author Raj Singh ([email protected]).
Author contributions
Sanjay Dwivedi (Conceptualization, Data curation, Formal analysis, Investigation, Validation, Writing—original draft), Naveen Chandra (Conceptualization, Data curation, Formal analysis, Investigation, Writing—original draft), Arun Mishra (Data curation, Formal analysis, Investigation, Software, Writing—original draft, Writing—review & editing) Sonam Bahuguna (Writing—original draft, Writing—review & editing), Aman Srivastava (Data curation, Formal analysis, Investigation, Writing—original draft, Writing—review & editing), Dhani Arya (Writing—original draft, Writing—review & editing), Gajendra Singh (Formal analysis, Investigation, Writing—original draft, Writing—review & editing), Bojan Đurin (Validation, Writing—review & editing), Nikola Kranjčić (Validation, Writing—original draft, Writing—review & editing), Raj Singh (Writing—review & editing)
Funding
Dr Gajendra Singh thanks the Govind Ballabh Pant National Institute of Himalayan Environment (GBPNIHE), Ministry of Environment, Forest and Climate Change (MoEF&CC), Government of India for financial assistance under Himalayan Knowledge Network (HKN) (Uttarakhand Chapter) program.
Conflicts of interest
The authors declare no competing interests.
Acknowledgments
The authors thank the director of the Uttarakhand Space Application Centre (USAC), Dehradun, India, for providing the required facilities, support, and motivation. We duly acknowledge GB Pant National Institute of Himalayan Environment (GBPNIHE), the Ministry of Environment, Forest and Climate Change (MoEF&CC), and the Government of India for financial assistance under the Himalayan Knowledge Network (Uttarakhand Chapter) program.