-
PDF
- Split View
-
Views
-
Cite
Cite
Iain Wilson, Adam Peters, Graham Merrington, Stijn Baken, Following the evidence and using the appropriate regulatory tools: A European‐wide risk assessment of copper in freshwaters, Integrated Environmental Assessment and Management, Volume 19, Issue 6, 1 November 2023, Pages 1570–1580, https://doi.org/10.1002/ieam.4768
- Share Icon Share
Abstract
The ecological risks of copper (Cu) in freshwaters have been the focus of regulatory assessments for several decades. Recently, it has been suggested by the European Commission that Cu represents a continent‐wide risk to freshwaters. We assessed to what extent this suggestion is supported by the available evidence if Cu bioavailability is considered in the assessment of risk. We used several evidence‐driven metrics to assess the continental‐wide risks of Cu to European freshwaters. Such an approach is recommended and readily applicable where comprehensive data sets are available. We confirmed the validity of a bioavailability‐based Environmental Quality Standard of 1 µg L−1 for Cu and used this to characterize the risks of Cu in 286 185 regulatory monitoring samples from 17 307 sites across 19 European countries between 2006 and 2021. These data show that risks, based on site averages and accounting for bioavailability, were identified in only two countries (Spain and Portugal). Investigation of these risks showed them to be highly localized to a single region in Spain and not reflective of the country‐wide risks for either country. The 95th percentile of all the risk quotients for the continent‐wide data set is 0.35. The relatively low levels of risk associated with Cu are supported by long‐term trend data from sites on two large European rivers (Rhine and Meuse), where highly significant (p < 0.001) decreases in Cu concentrations over the last 40 years can be observed. We conclude that it is critical to consider metal bioavailability in both effects and exposures in assessments of potential risk to ensure ecological relevance. Integr Environ Assess Manag 2023;19:1570–1580. © 2023 WCA Environment Ltd. Integrated Environmental Assessment and Management published by Wiley Periodicals LLC on behalf of Society of Environmental Toxicology & Chemistry (SETAC).
Key Points
We confirmed the validity of a bioavailability‐based Environmental Quality Standard of 1 µg L−1 for copper (Cu).
Following the applicable European Commission guidance and by using a set of metrics that are consistent with the approach adopted by the European Commission (JRC 2016; JRC 2021), we have demonstrated that Cu is unlikely to pose a continent‐wide risk.
It is critical to consider metal bioavailability in both effects and exposures in assessments of potential risk to ensure ecological relevance.
INTRODUCTION
Copper (Cu) is an essential trace metal and, in some aquatic organisms, has a relatively narrow concentration range between deficiency and toxicity (Fort et al., 2000). Copper has been regulated in surface waters by many jurisdictions using Environmental Quality Standards (EQSs), Quality Guidelines, Criteria, and Limit Values for over four decades. During this period, as scientific understanding of the behavior, fate, and ecotoxicity of Cu has developed, there has been a regulatory shift from the numerical values derived to determine Cu risks based upon water hardness ranges toward accounting for bioavailability through the use of mechanistic tools such as Biotic Ligand Models (BLMs; Di Toro et al., 2009). Effectively, bioavailability here is representative of what the aquatic organism experiences in terms of exposure in the water column. While such approaches have been available for over 20 years (Adams et al., 2019), routine regulatory implementation of bioavailability has taken somewhat longer to achieve and remains a “work in progress.” Nevertheless, in Europe, under the Water Framework Directive (WFD), new guidance for regulatory scientists and practitioners is now available to consistently set and implement metal EQSs that are bioavailability based to deliver an ecologically relevant assessment of risk (EC, 2018, 2019). Similarly, bioavailability‐based approaches are now used in the United States (USEPA, 2007), Canada (Environment and Climate Change Canada, 2021), Australia, and New Zealand (Anzecc & Armcanz, 2000).
Environmental Quality Standards for Cu in Europe
In the European Union (EU), Cu EQSs are derived nationally under Annex VIII of the WFD, and there is currently no continent‐wide standard, as it has not been deemed a continental‐wide risk. The numerical values of Cu EQSs can vary between countries. In total, 21 countries have set EQSs for Cu in freshwaters; the numerical values of these vary between 0.5 and 120 µg Cu L−1 (European Environment Agency, 2022). These EQSs are aimed to protect the aquatic environment from risks associated with long‐term Cu exposures. Likely, a few of these EQSs were derived appropriately using the most recent European Technical Guidance for Deriving Environmental Quality Standards (EQSs TGD) (CIS Guidance Document No. 27, European Commission, 2018).
In the United Kingdom, the Technical Advisory Group on the WFD derived a freshwater EQSbioavailable for Cu of 1 µg L−1 (UKTAG, 2012). This EQS accounts for bioavailability in its derivation and its implementation. A previous assessment demonstrated that if an EU‐wide EQSbioavailable for Cu was derived using the EQS TGD to ensure that it is protective of 95% of waters in the most sensitive region, it would be 1.1 µg L−1 (Peters et al., 2019); this value is also broadly in line with the value used by the JRC of 1 µg L−1 as cited in French legislation as EQSbioavailable for Cu (Journal officiel de la République française, 2015; JRC, 2022). An EQSbioavailable is effectively operationally defined in that bioavailability needs to be accounted for if the EQS is to deliver the relevant ecological measure of potential Cu risk in freshwaters. This is no different in principle from an EQS, for example, based on a measure of “free cyanide” or one that is derived from being measured in specific biota (such as mussels) or parts of biota (such as fish fillets). To be ecologically relevant measures of potential risk, it is imperative that such EQSs are appropriately implemented (e.g., Merrington et al., 2016; Peters et al., 2019, 2022).
Copper in European freshwaters and accounting for bioavailability
Historically, measures of Cu concentrations in surface waters were often considered “total” concentrations (e.g., The European Dangerous Substances Directive, 76/464/EEC), and over time, in line with local regulatory requirements, some jurisdictions moved toward filtered or “dissolved” Cu concentrations, reflective of apparent greater environmental relevancy (Mance et al., 1984). In Europe, there are publicly available measured data for dissolved Cu in freshwaters for more than 20 countries.
The UK Environment Agency developed an approach to account for bioavailability in measured concentrations of copper, nickel, zinc, and manganese based on the existing chronic BLMs and using a tiered framework (e.g., Comber et al., 2008). They recognized that the respective BLMs for these metals, while technically appropriate, are data intensive, too complex to run on routine software and interpret the outputs from, and so not an appropriate regulatory fit for routine assessments of large numbers of sampling sites. Therefore, a simplified tool was developed based on the outputs from the BLMs but just using inputs for water pH, calcium, dissolved organic carbon (DOC), and dissolved metal concentrations (M‐BAT, UKTAG, 2014). This tool is used in a tiered, risk‐based approach (UKTAG, 2012). The tool developed by the Environment Agency has now been further developed and validated against relevant and reliable ecotoxicity data (www.bio-met.net (bio‐met, 2019); Peters et al., 2020). The bio‐met tool is specifically aimed at regulatory use, and especially compliance assessment under the WFD for copper, nickel, zinc, lead, and cobalt, and only requires the same water chemistry inputs as the Environment Agency approach.
The European Commission has recently suggested that Cu represents an EU‐wide concern and that risks are present in freshwaters (JRC, 2022). The suggestion of EU‐wide risks due to Cu means that an EU‐wide mandatory EQS is to be derived and implemented. The “suggestion” is based on a face‐value comparison of the EQSbioavailable for Cu of 1 µg L−1 against a 95th percentile Cu concentration estimated from less than 5000 European sites, without accounting for bioavailability. This approach takes the operationally defined EQSbioavailable for Cu but compares it with dissolved Cu concentrations that are not corrected for bioavailability. This scientifically compromised and ecologically irrelevant approach to assessing potential Cu risks is not in line with the Technical Guidance for implementing EQSs for metals (EC, 2019). This guidance states that waterbodies exceeding the EQSbioavailable at tier 1 should progress to tier 2, where the use of simplified tools is recommended, and if required, tier 3, where local refinement can be implemented (EC, 2019).
Here, we assess Cu risks in freshwaters using the European Commission's own recently published guidance documents on setting and implementing bioavailability‐based EQSs for metals (EC, 2018; 2019) and publicly available regulatory monitoring data from across Europe. This extensive continental‐scale assessment will determine the scale and magnitude of the potential risks from Cu in freshwaters using an appropriate ecologically relevant metric. We will provide context to this assessment by examination of long‐term time trends of Cu concentrations in European freshwaters.
MATERIALS AND METHODS
Copper concentrations in European freshwaters
Measured Cu concentrations used in this assessment were obtained from European Member State (MS) environment agencies, through either direct contact or from online dissemination portals, and from International River commissions, following the process performed in Peters et al. (2022) (references for all data sets are included in the Supporting Information). Dissolved Cu data were collected for the years 2006–2021, and data were obtained from 19 EU MS plus Switzerland; the data set contained 286 185 samples from 17 307 sites (Table 1). All data were generated by, or on behalf of, European competent authorities and are used for national and international monitoring requirements and are therefore deemed suitable for use for the purpose of this assessment.
Country | 2006 onward | 2013 onward | 2018–2020 | ||||||
No. of sites | No. of samples | Samples with pH, DOC, and Ca | No. of sites | No. of samples | Samples with pH, DOC, and Ca | No. of sites | No. of samples | Samples with pH, DOC, and Ca | |
Austria | 94 | 1897 | 1806 | 94 | 1668 | 1578 | 13 | 98 | 57 |
Bulgaria | 12 | 828 | 0 | 12 | 828 | 0 | 12 | 160 | 0 |
Croatia | 12 | 1602 | 668 | 11 | 1097 | 666 | 8 | 21 | 0 |
Czech Republic | 2 | 286 | 132 | 2 | 132 | 84 | 2 | 24 | 0 |
Estonia | 236 | 2240 | 264 | 213 | 1974 | 264 | 119 | 730 | 104 |
Finland | 171 | 2284 | 233 | 126 | 1965 | 106 | 53 | 293 | 28 |
France | 6390 | 151 199 | 50 267 | 6082 | 117 198 | 44 240 | 4164 | 39 730 | 20 460 |
Germany | 8 | 617 | 500 | 7 | 249 | 161 | 3 | 52 | 14 |
Hungary | 15 | 2501 | 125 | 15 | 1367 | 0 | 15 | 255 | 0 |
Ireland | 277 | 5463 | 1639 | 277 | 5463 | 1639 | 176 | 1413 | 570 |
Italy | 138 | 2402 | 1938 | 138 | 2402 | 1938 | 112 | 1588 | 1124 |
Latvia | 403 | 3197 | 3258 | 403 | 3197 | 3137 | 312 | 2429 | 2422 |
Netherlands | 4815 | 47 954 | 26 130 | 4460 | 44 301 | 22 609 | 3242 | 30 382 | 8692 |
Portugal | 1137 | 6290 | 44 | 1137 | 6258 | 44 | 1001 | 3761 | 34 |
Romania | 21 | 2910 | 1172 | 21 | 1963 | 1149 | 21 | 453 | 439 |
Slovak Republic | 6 | 1051 | 0 | 6 | 604 | 0 | 6 | 133 | 0 |
Slovenia | 2 | 280 | 48 | 2 | 144 | 48 | 2 | 24 | 0 |
Spain | 2853 | 44 190 | 241 | 2853 | 44 190 | 241 | 2832 | 38 052 | 237 |
Sweden | 646 | 8737 | 885 | 622 | 7906 | 786 | 313 | 3738 | 575 |
Switzerlanda | 1 | 125 | 125 | 1 | 6 | 6 | 0 | 0 | 0 |
Total | 17 239 | 286 053 | 89 475 | 16 482 | 242 912 | 78 696 | 12 406 | 123 336 | 34 756 |
Country | 2006 onward | 2013 onward | 2018–2020 | ||||||
No. of sites | No. of samples | Samples with pH, DOC, and Ca | No. of sites | No. of samples | Samples with pH, DOC, and Ca | No. of sites | No. of samples | Samples with pH, DOC, and Ca | |
Austria | 94 | 1897 | 1806 | 94 | 1668 | 1578 | 13 | 98 | 57 |
Bulgaria | 12 | 828 | 0 | 12 | 828 | 0 | 12 | 160 | 0 |
Croatia | 12 | 1602 | 668 | 11 | 1097 | 666 | 8 | 21 | 0 |
Czech Republic | 2 | 286 | 132 | 2 | 132 | 84 | 2 | 24 | 0 |
Estonia | 236 | 2240 | 264 | 213 | 1974 | 264 | 119 | 730 | 104 |
Finland | 171 | 2284 | 233 | 126 | 1965 | 106 | 53 | 293 | 28 |
France | 6390 | 151 199 | 50 267 | 6082 | 117 198 | 44 240 | 4164 | 39 730 | 20 460 |
Germany | 8 | 617 | 500 | 7 | 249 | 161 | 3 | 52 | 14 |
Hungary | 15 | 2501 | 125 | 15 | 1367 | 0 | 15 | 255 | 0 |
Ireland | 277 | 5463 | 1639 | 277 | 5463 | 1639 | 176 | 1413 | 570 |
Italy | 138 | 2402 | 1938 | 138 | 2402 | 1938 | 112 | 1588 | 1124 |
Latvia | 403 | 3197 | 3258 | 403 | 3197 | 3137 | 312 | 2429 | 2422 |
Netherlands | 4815 | 47 954 | 26 130 | 4460 | 44 301 | 22 609 | 3242 | 30 382 | 8692 |
Portugal | 1137 | 6290 | 44 | 1137 | 6258 | 44 | 1001 | 3761 | 34 |
Romania | 21 | 2910 | 1172 | 21 | 1963 | 1149 | 21 | 453 | 439 |
Slovak Republic | 6 | 1051 | 0 | 6 | 604 | 0 | 6 | 133 | 0 |
Slovenia | 2 | 280 | 48 | 2 | 144 | 48 | 2 | 24 | 0 |
Spain | 2853 | 44 190 | 241 | 2853 | 44 190 | 241 | 2832 | 38 052 | 237 |
Sweden | 646 | 8737 | 885 | 622 | 7906 | 786 | 313 | 3738 | 575 |
Switzerlanda | 1 | 125 | 125 | 1 | 6 | 6 | 0 | 0 | 0 |
Total | 17 239 | 286 053 | 89 475 | 16 482 | 242 912 | 78 696 | 12 406 | 123 336 | 34 756 |
Abbreviations: DOC, dissolved organic carbon; EU, European Union.
Switzerland is a non‐EU country; however, data were available for this country in the International Commission for the Protection of the Rhine data set, and therefore, were included in this assessment.
Country | 2006 onward | 2013 onward | 2018–2020 | ||||||
No. of sites | No. of samples | Samples with pH, DOC, and Ca | No. of sites | No. of samples | Samples with pH, DOC, and Ca | No. of sites | No. of samples | Samples with pH, DOC, and Ca | |
Austria | 94 | 1897 | 1806 | 94 | 1668 | 1578 | 13 | 98 | 57 |
Bulgaria | 12 | 828 | 0 | 12 | 828 | 0 | 12 | 160 | 0 |
Croatia | 12 | 1602 | 668 | 11 | 1097 | 666 | 8 | 21 | 0 |
Czech Republic | 2 | 286 | 132 | 2 | 132 | 84 | 2 | 24 | 0 |
Estonia | 236 | 2240 | 264 | 213 | 1974 | 264 | 119 | 730 | 104 |
Finland | 171 | 2284 | 233 | 126 | 1965 | 106 | 53 | 293 | 28 |
France | 6390 | 151 199 | 50 267 | 6082 | 117 198 | 44 240 | 4164 | 39 730 | 20 460 |
Germany | 8 | 617 | 500 | 7 | 249 | 161 | 3 | 52 | 14 |
Hungary | 15 | 2501 | 125 | 15 | 1367 | 0 | 15 | 255 | 0 |
Ireland | 277 | 5463 | 1639 | 277 | 5463 | 1639 | 176 | 1413 | 570 |
Italy | 138 | 2402 | 1938 | 138 | 2402 | 1938 | 112 | 1588 | 1124 |
Latvia | 403 | 3197 | 3258 | 403 | 3197 | 3137 | 312 | 2429 | 2422 |
Netherlands | 4815 | 47 954 | 26 130 | 4460 | 44 301 | 22 609 | 3242 | 30 382 | 8692 |
Portugal | 1137 | 6290 | 44 | 1137 | 6258 | 44 | 1001 | 3761 | 34 |
Romania | 21 | 2910 | 1172 | 21 | 1963 | 1149 | 21 | 453 | 439 |
Slovak Republic | 6 | 1051 | 0 | 6 | 604 | 0 | 6 | 133 | 0 |
Slovenia | 2 | 280 | 48 | 2 | 144 | 48 | 2 | 24 | 0 |
Spain | 2853 | 44 190 | 241 | 2853 | 44 190 | 241 | 2832 | 38 052 | 237 |
Sweden | 646 | 8737 | 885 | 622 | 7906 | 786 | 313 | 3738 | 575 |
Switzerlanda | 1 | 125 | 125 | 1 | 6 | 6 | 0 | 0 | 0 |
Total | 17 239 | 286 053 | 89 475 | 16 482 | 242 912 | 78 696 | 12 406 | 123 336 | 34 756 |
Country | 2006 onward | 2013 onward | 2018–2020 | ||||||
No. of sites | No. of samples | Samples with pH, DOC, and Ca | No. of sites | No. of samples | Samples with pH, DOC, and Ca | No. of sites | No. of samples | Samples with pH, DOC, and Ca | |
Austria | 94 | 1897 | 1806 | 94 | 1668 | 1578 | 13 | 98 | 57 |
Bulgaria | 12 | 828 | 0 | 12 | 828 | 0 | 12 | 160 | 0 |
Croatia | 12 | 1602 | 668 | 11 | 1097 | 666 | 8 | 21 | 0 |
Czech Republic | 2 | 286 | 132 | 2 | 132 | 84 | 2 | 24 | 0 |
Estonia | 236 | 2240 | 264 | 213 | 1974 | 264 | 119 | 730 | 104 |
Finland | 171 | 2284 | 233 | 126 | 1965 | 106 | 53 | 293 | 28 |
France | 6390 | 151 199 | 50 267 | 6082 | 117 198 | 44 240 | 4164 | 39 730 | 20 460 |
Germany | 8 | 617 | 500 | 7 | 249 | 161 | 3 | 52 | 14 |
Hungary | 15 | 2501 | 125 | 15 | 1367 | 0 | 15 | 255 | 0 |
Ireland | 277 | 5463 | 1639 | 277 | 5463 | 1639 | 176 | 1413 | 570 |
Italy | 138 | 2402 | 1938 | 138 | 2402 | 1938 | 112 | 1588 | 1124 |
Latvia | 403 | 3197 | 3258 | 403 | 3197 | 3137 | 312 | 2429 | 2422 |
Netherlands | 4815 | 47 954 | 26 130 | 4460 | 44 301 | 22 609 | 3242 | 30 382 | 8692 |
Portugal | 1137 | 6290 | 44 | 1137 | 6258 | 44 | 1001 | 3761 | 34 |
Romania | 21 | 2910 | 1172 | 21 | 1963 | 1149 | 21 | 453 | 439 |
Slovak Republic | 6 | 1051 | 0 | 6 | 604 | 0 | 6 | 133 | 0 |
Slovenia | 2 | 280 | 48 | 2 | 144 | 48 | 2 | 24 | 0 |
Spain | 2853 | 44 190 | 241 | 2853 | 44 190 | 241 | 2832 | 38 052 | 237 |
Sweden | 646 | 8737 | 885 | 622 | 7906 | 786 | 313 | 3738 | 575 |
Switzerlanda | 1 | 125 | 125 | 1 | 6 | 6 | 0 | 0 | 0 |
Total | 17 239 | 286 053 | 89 475 | 16 482 | 242 912 | 78 696 | 12 406 | 123 336 | 34 756 |
Abbreviations: DOC, dissolved organic carbon; EU, European Union.
Switzerland is a non‐EU country; however, data were available for this country in the International Commission for the Protection of the Rhine data set, and therefore, were included in this assessment.
Additionally, where available, pH, DOC, and dissolved Ca were also collected for each sample to allow for bioavailability to be accounted for. In total, 18 countries had data that could be bioavailability‐corrected, and the data set equated to 89 439 samples (Table 1 and Supporting Information). To perform the same calculations as those advocated by the European Commission, additional data were also collected (e.g., JRC, 2015; von der Ohe et al., 2011). For the calculation of the country‐specific 95th percentile of risk quotients (RQs), site average concentrations were utilized (see below). Assessments were performed on three versions of the data set, which each used different approaches for data selection:
A: a data set containing all the data obtained for the purpose of this assessment,
B: a data set that excludes samples below the limit of quantification (LoQ) if the LoQ is greater than two times the EQSbioavailable. This approach is consistent with the one used by the European Commission (JRC, 2022), where an EU‐wide risk due to Cu was suggested; and
C: a data set that additionally excludes samples outside the validated range of the Cu BLM (and so also Bio‐met; the validated range is pH 6–8.5 and 3.1–129 mg L−1 for dissolved calcium) to ensure that bioavailability predictions are being implemented correctly.
A high portion of samples and sites in the data set were from France (∼53% of the overall number of samples). Therefore, the assessment was performed both with and without French data to assess if the weighting of the data toward a single country influenced the overall European compliance rate. Further assessments were also performed using subsets of the original data set covering the years from 2013 to 2021 and from 2018 to 2020 (the last three complete sampling years) to assess any changes in compliance with the EQSbioavailable over time.
Confirming the value of the EQSbioavailable for copper
The previously derived EQSbioavailable value of 1.1 µg L−1 was derived from a relatively limited data set. Based on the more extensive and more recent exposure data set and following the approach outlined by the European Commission (2018), we assessed whether that value (for the purposes of this study rounded to 1 µg L−1) is still valid. We have used data from Austria for this assessment (Table 1), as it has been previously shown that Austria is the most sensitive region to Cu exposure in Europe (Peters et al., 2019). The sensitivity of the waters to Cu was calculated using CuBioM version 1.0 (UKCEH, 2022) for all samples with matched data for pH, DOC, and Ca. CuBioM implements the Cu bioavailability models from the Copper Voluntary Risk Assessment agreed upon by EUMS in 2008. It utilizes the same bioavailability models that MS use to derive the EQSbioavailable for Cu (UKCEH, 2022).
Assessing compliance against the EQSbioavailable
This assessment of compliance against the EQSbioavailable follows the Tiered Approach originally set out by the UK Environment Agency (UKTAG, 2012) and modified in the recent European Commission guidance (EC, 2019). At tier 1, a “face value comparison” is made between the dissolved Cu concentration in the sample and the EQSbioavailable. At tier 2, the bioavailable Cu concentration (calculated using the bio‐met tool) is compared to the EQSbioavailable as recommended in the European Commission guidance (EC, 2019). At both tiers, RQs are determined by dividing the exposure concentration by the EQSbioavailable.
In this assessment, several metrics have been developed previously and used for identifying continent‐wide risks of chemicals in water in the context of substance prioritization under the WFD (JRC, 2016, 2021). These metrics include the following:
The Spatial, Temporal and Extent of exceedances (STE) score; this score considers the Spatial scale, the Temporal scale, and the Extent of the Predicted No Effect Concentration exceedances and is discussed in greater detail below (JRC, 2015; von der Ohe et al., 2011).
The 95th percentile of the RQs is calculated for all samples in the data set; if this metric exceeds 1, an EU‐wide risk could be indicated (JRC, 2021).
The number of countries where more than 5% of the sites exceed the EQSs. If four or more countries have greater than 5% exceedances, this could be indicative of an EU‐wide risk (JRC, 2021).
The STE score is a metric that accounts for potential risk by summing the spatial factor of exceedances (Fspatial), the temporal extent of exceedances (Ftemporal), and the extent factor of exceedances (Fextent) to provide an overall score, with the magnitude of the overall STE score indicating the potential risk. This method has been developed by the JRC (JRC, 2015) based on the work of von der Ohe et al. (2011); it has been used for many risk assessment purposes and also utilized in other publications (e.g., Peters et al., 2022). The overall STE score is banded to indicate an EU‐wide risk, as detailed below (JRC, 2016).
<0.6 = very low risk
≥0.6 < 1.2 = low risk
≥1.2 < 1.8 = intermediate risk
≥1.8 < 2.4 = high risk
≥2.4 ≤ 3 = very high risk
This metric was calculated at tier 1 and tier 2. At tier 2, when accounting for bioavailability, samples lacking the required supporting parameters were excluded as they could not be assessed for accounting for the bioavailability and did not allow for the correct implementation of the compliance metric. To implement the EQSbioavailable correctly, bioavailability must be accounted for.
To provide context to the compliance assessment, long‐term data sets were gathered for two sites on major European rivers. Data covering the 1968–2020 time span were obtained for the Lobith monitoring site on the Rhine River in the Netherlands for both total (n = 1419 samples) and dissolved Cu (n = 1428 samples), with 724 samples from 1978 to 2020 having the parameters needed for bioavailability correction, specifically pH, DOC, and dissolved calcium (Rijkswaterstaat, 2022). Data covering the 1972–2020 time span were obtained for the Eijsden ponton monitoring site on the River Meuse for both total (n = 1914 samples) and dissolved Cu (n = 1898 samples), with 864 samples from 1982 onward having the parameters needed for bioavailability correction (Rijkswaterstaat, 2022). Plots of these data sets are included in the Supporting Information.
RISK ASSESSMENT OF COPPER AT THE CONTINENTAL SCALE
To be able to assess compliance on an EU‐wide scale, the initial step is to set an EU‐wide EQS for Cu. After the metric has been calculated, tier 1 and tier 2 assessments can then be performed.
Setting an EU‐wide EQS for copper
The data set for the EU region that is most sensitive to Cu (Austria) includes 1806 samples from 94 sites with matched pH, DOC, Ca, and dissolved Cu available. Bioavailability calculations for these samples resulted in a set of HC5 values with a 5th percentile of 1.1 µg L−1 and a median of 3.9 µg L−1. The 5th percentile for Austrian surface waters is consistent with that calculated by Peters et al. (2019) and that used for the UK EQS (UKTAG, 2012). According to EC (2018), the EQSbioavailable is therefore set on the basis of this value, for the purpose of simplicity, rounded to 1 µg L−1 Cu. This is slightly conservative relative to the 5th percentile HC5 value of the Austrian data set. The surface waters in Austria are typified by a relatively high pH (median = 8.14), low DOC (median = 2.0 mg L−1), and moderate Ca concentrations (median = 51.5 mg L−1). Copper exposures are generally relatively low (median = 1.16 µg L−1), and RQ values in these samples are low, with a median value of 0.27 and a 95th percentile value of 1.09. Typical water chemistry conditions resulting in an HC5 value equal to the EQSbioavailable of 1.0 µg L−1 are pH 8.4, 0.7 mg L−1 DOC, and 60 mg L−1 Ca.
Tier 1 assessment
The importance of accounting for bioavailability during metal risk assessments has been well documented (e.g., Mebane et al., 2020; Venelinov & Tsakovski, 2022). However, to provide context, a tier 1 assessment of all data has been performed (Table 2). At tier 1, without accounting for bioavailability, the STE score indicates an intermediate risk in all data sets. The spatial scale of exceedance (Fspatial component) is the largest contribution to the score (Table 2). However, it is important to note that the score decreases as older data are removed from the assessment. Almost all countries have exceedances in more than 5% of the samples, and the 95th percentile of RQs in the data set is far above 1. Taken together, these metrics do not indicate the existence of a severe EU‐wide risk, as could be assumed at first glance. Rather, they emphasize the need to proceed to tier 2, as described in the EC (2018) guidance.
Risk assessment of dissolved Cu in EU freshwater using various metrics without accounting for bioavailability (tier 1)
Time span of the data set | From 2006 onward | From 2013 onward | 2018–2020 |
Data selection approach | A (all data) | A (all data) | A (all data) |
No. of samples | 286 185 | 200 756 | 103 164 |
No. of sites | 17 307 | 14 899 | 11 800 |
No. of countries | 20 | 20 | 19 |
Fspatial | 0.84 | 0.81 | 0.77 |
Ftemporal | 0.65 | 0.64 | 0.67 |
Fextent | 0.28 | 0.18 | 0.18 |
STE score | 1.76 | 1.63 | 1.62 |
95th percentile of risk quotients | 5.47 | 5.00 | 5.84 |
Number of countries with more than 5% exceedances | 20 | 20 | 18 |
Time span of the data set | From 2006 onward | From 2013 onward | 2018–2020 |
Data selection approach | A (all data) | A (all data) | A (all data) |
No. of samples | 286 185 | 200 756 | 103 164 |
No. of sites | 17 307 | 14 899 | 11 800 |
No. of countries | 20 | 20 | 19 |
Fspatial | 0.84 | 0.81 | 0.77 |
Ftemporal | 0.65 | 0.64 | 0.67 |
Fextent | 0.28 | 0.18 | 0.18 |
STE score | 1.76 | 1.63 | 1.62 |
95th percentile of risk quotients | 5.47 | 5.00 | 5.84 |
Number of countries with more than 5% exceedances | 20 | 20 | 18 |
Note: Italic values are key metrics.
Abbreviations: Cu, copper; EU, European Union; STE, Spatial, Temporal and Extent of exceedances.
Risk assessment of dissolved Cu in EU freshwater using various metrics without accounting for bioavailability (tier 1)
Time span of the data set | From 2006 onward | From 2013 onward | 2018–2020 |
Data selection approach | A (all data) | A (all data) | A (all data) |
No. of samples | 286 185 | 200 756 | 103 164 |
No. of sites | 17 307 | 14 899 | 11 800 |
No. of countries | 20 | 20 | 19 |
Fspatial | 0.84 | 0.81 | 0.77 |
Ftemporal | 0.65 | 0.64 | 0.67 |
Fextent | 0.28 | 0.18 | 0.18 |
STE score | 1.76 | 1.63 | 1.62 |
95th percentile of risk quotients | 5.47 | 5.00 | 5.84 |
Number of countries with more than 5% exceedances | 20 | 20 | 18 |
Time span of the data set | From 2006 onward | From 2013 onward | 2018–2020 |
Data selection approach | A (all data) | A (all data) | A (all data) |
No. of samples | 286 185 | 200 756 | 103 164 |
No. of sites | 17 307 | 14 899 | 11 800 |
No. of countries | 20 | 20 | 19 |
Fspatial | 0.84 | 0.81 | 0.77 |
Ftemporal | 0.65 | 0.64 | 0.67 |
Fextent | 0.28 | 0.18 | 0.18 |
STE score | 1.76 | 1.63 | 1.62 |
95th percentile of risk quotients | 5.47 | 5.00 | 5.84 |
Number of countries with more than 5% exceedances | 20 | 20 | 18 |
Note: Italic values are key metrics.
Abbreviations: Cu, copper; EU, European Union; STE, Spatial, Temporal and Extent of exceedances.
Tier 2 assessment: Accounting for bioavailability
When the bioavailability of Cu is taken into account, the STE scores for all data sets are less than 0.6, which indicates very low risk, with the maximum score being 0.43 (Table 3). When comparing the results to the tier 1 assessment, this shows that accounting for bioavailability has a dramatic effect on the calculated risk from dissolved Cu in European waters. The low scores for Fspatial and Fextent demonstrate that the spatial scale of Cu exceedances is very minimal and that the exceedances are only slight.
Risk assessment of dissolved Cu in EU freshwater using various metrics at tier 2 (with bioavailability accounted for)
Time span of the data set | From 2006 onward | From 2013 onward | 2018–2020 | ||||||
Data selection approacha | A | B | C | A | B | C | A | B | C |
No. of samplesb | 89 439 | 88 709 | 77 617 | 78 781 | 78 325 | 68 456 | 34 650 | 34 410 | 30 828 |
No. of sitesb | 8714 | 8667 | 8102 | 8122 | 8096 | 7566 | 5482 | 5456 | 5076 |
No. of countriesb | 18 | 18 | 18 | 17 | 17 | 17 | 14 | 14 | 14 |
Fspatial | 0.05 | 0.05 | 0.04 | 0.04 | 0.04 | 0.03 | 0.04 | 0.04 | 0.03 |
Ftemporal | 0.20 | 0.20 | 0.18 | 0.22 | 0.21 | 0.19 | 0.35 | 0.33 | 0.32 |
Fextent | 0.04 | 0.00 | 0.00 | 0.04 | 0.04 | 0.00 | 0.04 | 0.04 | 0.00 |
STE score | 0.29 | 0.24 | 0.22 | 0.30 | 0.29 | 0.22 | 0.43 | 0.41 | 0.35 |
95th percentile of risk quotients | 0.38 | 0.35 | 0.35 | 0.37 | 0.35 | 0.35 | 0.45 | 0.41 | 0.40 |
Number of countries with more than 5% exceedances | 3 | 3 | 2 | 4 | 3 | 1 | 3 | 1 | 1 |
Time span of the data set | From 2006 onward | From 2013 onward | 2018–2020 | ||||||
Data selection approacha | A | B | C | A | B | C | A | B | C |
No. of samplesb | 89 439 | 88 709 | 77 617 | 78 781 | 78 325 | 68 456 | 34 650 | 34 410 | 30 828 |
No. of sitesb | 8714 | 8667 | 8102 | 8122 | 8096 | 7566 | 5482 | 5456 | 5076 |
No. of countriesb | 18 | 18 | 18 | 17 | 17 | 17 | 14 | 14 | 14 |
Fspatial | 0.05 | 0.05 | 0.04 | 0.04 | 0.04 | 0.03 | 0.04 | 0.04 | 0.03 |
Ftemporal | 0.20 | 0.20 | 0.18 | 0.22 | 0.21 | 0.19 | 0.35 | 0.33 | 0.32 |
Fextent | 0.04 | 0.00 | 0.00 | 0.04 | 0.04 | 0.00 | 0.04 | 0.04 | 0.00 |
STE score | 0.29 | 0.24 | 0.22 | 0.30 | 0.29 | 0.22 | 0.43 | 0.41 | 0.35 |
95th percentile of risk quotients | 0.38 | 0.35 | 0.35 | 0.37 | 0.35 | 0.35 | 0.45 | 0.41 | 0.40 |
Number of countries with more than 5% exceedances | 3 | 3 | 2 | 4 | 3 | 1 | 3 | 1 | 1 |
Note: Italic values are key metrics.
Abbreviations: Cu, copper; EU, European Union; STE, Spatial, Temporal and Extent of exceedances.
Data selection approach A includes all data; data selection approach B excludes some samples below the limit of quantification (LoQ), in line with JRC (2022); and data selection approach C excludes some samples below the LoQ as well as samples outside the validated range of the copper Biotic Ligand Models.
Numbers of samples, sites, and countries in this table refer to those data entries for which the necessary parameters to correct for bioavailability are available.
Risk assessment of dissolved Cu in EU freshwater using various metrics at tier 2 (with bioavailability accounted for)
Time span of the data set | From 2006 onward | From 2013 onward | 2018–2020 | ||||||
Data selection approacha | A | B | C | A | B | C | A | B | C |
No. of samplesb | 89 439 | 88 709 | 77 617 | 78 781 | 78 325 | 68 456 | 34 650 | 34 410 | 30 828 |
No. of sitesb | 8714 | 8667 | 8102 | 8122 | 8096 | 7566 | 5482 | 5456 | 5076 |
No. of countriesb | 18 | 18 | 18 | 17 | 17 | 17 | 14 | 14 | 14 |
Fspatial | 0.05 | 0.05 | 0.04 | 0.04 | 0.04 | 0.03 | 0.04 | 0.04 | 0.03 |
Ftemporal | 0.20 | 0.20 | 0.18 | 0.22 | 0.21 | 0.19 | 0.35 | 0.33 | 0.32 |
Fextent | 0.04 | 0.00 | 0.00 | 0.04 | 0.04 | 0.00 | 0.04 | 0.04 | 0.00 |
STE score | 0.29 | 0.24 | 0.22 | 0.30 | 0.29 | 0.22 | 0.43 | 0.41 | 0.35 |
95th percentile of risk quotients | 0.38 | 0.35 | 0.35 | 0.37 | 0.35 | 0.35 | 0.45 | 0.41 | 0.40 |
Number of countries with more than 5% exceedances | 3 | 3 | 2 | 4 | 3 | 1 | 3 | 1 | 1 |
Time span of the data set | From 2006 onward | From 2013 onward | 2018–2020 | ||||||
Data selection approacha | A | B | C | A | B | C | A | B | C |
No. of samplesb | 89 439 | 88 709 | 77 617 | 78 781 | 78 325 | 68 456 | 34 650 | 34 410 | 30 828 |
No. of sitesb | 8714 | 8667 | 8102 | 8122 | 8096 | 7566 | 5482 | 5456 | 5076 |
No. of countriesb | 18 | 18 | 18 | 17 | 17 | 17 | 14 | 14 | 14 |
Fspatial | 0.05 | 0.05 | 0.04 | 0.04 | 0.04 | 0.03 | 0.04 | 0.04 | 0.03 |
Ftemporal | 0.20 | 0.20 | 0.18 | 0.22 | 0.21 | 0.19 | 0.35 | 0.33 | 0.32 |
Fextent | 0.04 | 0.00 | 0.00 | 0.04 | 0.04 | 0.00 | 0.04 | 0.04 | 0.00 |
STE score | 0.29 | 0.24 | 0.22 | 0.30 | 0.29 | 0.22 | 0.43 | 0.41 | 0.35 |
95th percentile of risk quotients | 0.38 | 0.35 | 0.35 | 0.37 | 0.35 | 0.35 | 0.45 | 0.41 | 0.40 |
Number of countries with more than 5% exceedances | 3 | 3 | 2 | 4 | 3 | 1 | 3 | 1 | 1 |
Note: Italic values are key metrics.
Abbreviations: Cu, copper; EU, European Union; STE, Spatial, Temporal and Extent of exceedances.
Data selection approach A includes all data; data selection approach B excludes some samples below the limit of quantification (LoQ), in line with JRC (2022); and data selection approach C excludes some samples below the LoQ as well as samples outside the validated range of the copper Biotic Ligand Models.
Numbers of samples, sites, and countries in this table refer to those data entries for which the necessary parameters to correct for bioavailability are available.
The calculated STE scores are, overall, only marginally affected by the data set that is used, ranging from 0.22 to 0.43. Data selection approach C, which excludes some samples below the LoQ and those outside the validated range of the Cu BLM, results in the lowest STE scores on each occasion (Table 3). They only slightly depend on the data selection approach, with lower scores observed if data below the LOQ are excluded and/or if the data that are outside the validated ranges of the Cu bioavailability model are excluded. Some limited variation in scores is observed if the most represented country is excluded (France; Supporting Information: Table SA.4). However, the overall class of very low risk was concluded in all these assessments.
The 95th percentile of all RQs in the database is below 1 for all data selection approaches (Table 3 and Supporting Information). Depending on the data set, it ranges between 0.28 and 0.45, that is, more than a factor of 2 below the threshold of 1 that has been used to identify an EU‐wide risk.
At tier 2, the number of countries showing exceedances in more than 5% of the samples ranges from 1 to 4, depending on the data selection approach. The importance of considering bioavailability is again emphasized by comparing this outcome to the tier 1 assessment, where almost all countries were deemed to have more than 5% exceedances. In all assessments, more than half of the EUMS are represented. The suggested threshold for triggering an EU‐wide risk of four MS is only reached for one out of nine data sets: the one with all data from 2013 onward (Table 3 and Supporting Information). This data set includes samples where the result is below the LoQ of the analytical method and that LoQ is greater than twice the EQSbioavailable (i.e., data selection scenario A). Clearly, such data can skew the identification of potential risks. In all data sets that exclude such data, the number of exceedances is below 4.
It is important to note that the assessment for bioavailability in this study was performed using only the user‐friendly BLM tool bio‐met. According to the EC (2019), samples with exceedances at tier 2 should proceed to tier 3, where the full BLM or ambient background concentrations must be considered. Such an assessment could be conducted using CuBioM (UKCEH, 2022) and would likely further lower the various calculated metrics. However, the tier 3 assessment was beyond the scope of this study. Even without a tier 3 assessment, the available data clearly indicate that there is no continent‐wide risk.
Trends in copper exposure concentrations
The long‐term trend analysis shows a clear decrease in total (see Supporting Information) and dissolved (Figures 1 and 2) Cu concentrations over time. The data set was assessed to identify a breakpoint based on a segmented linear regression, which identified a breakpoint in the data set during January 1983, with a 95% confidence interval between February 1982 and November 1983. Analysis of the pre‐1983 data identified a highly significant (p < 0.001) reduction in dissolved Cu concentrations of −0.47 µg L−1 year−1, and analysis of the data from 1983 onward also identified a highly significant (p < 0.001) reduction in dissolved Cu concentrations of −0.047 µg L−1 year−1. This trend could be postulated to be due to an increased sensitivity in the analytical method; however, this cannot be confirmed when reviewing the raw data as the first censored sample does not occur at the Lobith site until 1995, and there are only two censored samples in the complete data set (Supporting Information: Table SA.2).
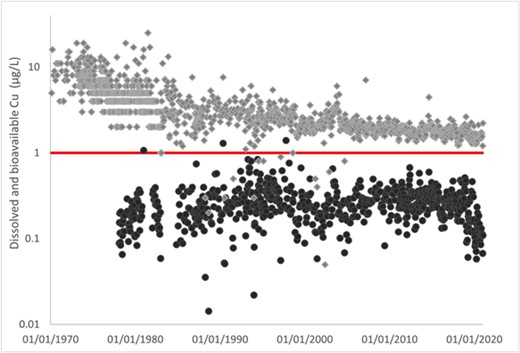
Dissolved Cu concentration (gray) and bioavailable Cu concentration (black) at Lobith monitoring station on River Rhine between 1970 and 2020 (raw data source: Rijkswaterstaat, 2022). The horizontal line indicates the EQSbioavailable for Cu of 1 µg L−1. Cu, copper; EQS, Environmental Quality Standard
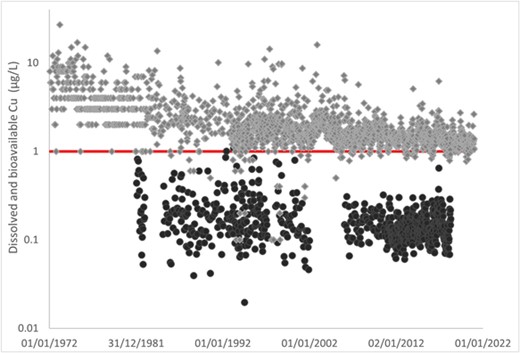
Dissolved Cu concentration (gray) and bioavailable Cu concentration (black) at Eijsden ponton monitoring station on River Meuse between 1972 and 2020 (raw data source: Rijkswaterstaat, 2022). The horizontal line indicates the EQSbioavailable for Cu of 1 µg L−1. Cu, copper; EQS, Environmental Quality Standard
The long‐term trend analysis also highlights the importance of bioavailability correction. At tier 1, more than 99% of samples at the Lobith site on the River Rhine exceed the EQSbioavailable of 1 µg L−1. However, after considering bioavailability at tier 2, only three samples out of 724 exceed the compliance metric, and these were all samples from before the year 2000 (Figure 1). Similarly, 93% of samples at the Eijsden ponton on the River Meuse exceed the EQSbioavailable at tier 1, but only four exceed the compliance metric at tier 2. The same pattern is observed when annual average data are used, with the compliance metric exceed in all years at tier 1, but at tier 2, no exceedances are observed.
DISCUSSION
This study indicates that when accounting for bioavailability, there is no EU‐wide risk of Cu in European freshwaters. This conclusion was reached using the same metrics and the same thresholds as those used by the European Commission in a recent study on substance prioritization under the WFD (JRC, 2016, 2021). There are publicly available dissolved Cu data for the great majority of EUMS from 2006 onward; however, the number of countries and sites where the bioavailability‐supporting parameters are available is not as extensive, as highlighted by only 31% of samples in the overall data set having the required supporting parameters, and two countries having no sites with the required supporting parameters (Table 1). A comparison at face value of the dissolved Cu concentration to the EQSbioavailable of 1 µg L−1 (tier 1 assessment) provides a poor estimate of risk as it does not account for bioavailability using the water chemistry conditions at the site. When risk metrics are applied at tier 1, dissolved Cu appears to present an EU‐wide risk (Table 2). This is also evident from a map with the 95th percentile of the RQs in each country (Figure 3). However, any exceedance of the EQSbioavailable in a tier 1 assessment must be followed by a more in‐depth assessment that considers bioavailability on the basis of the pH, DOC, and dissolved calcium present at a site (tier 2 assessment). In our data set, such information is available for 31% of the samples. Our work showed that, following a tier 2 assessment, dissolved Cu does not present an EU‐wide risk according to any of the metrics (Table 3 and Figure 3). This shows the importance of gathering the necessary data to allow for a bioavailability correction and, equally, to pursue the entire tiered approach before risks due to Cu are concluded.
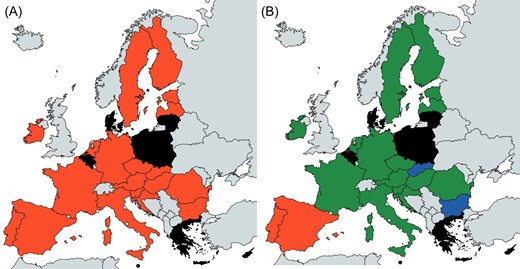
The 95th percentile of the risk quotients in each country based on a tier 1 assessment (A, left, no bioavailability considered) and a tier 2 assessment (B, right, with bioavailability considered). This assessment used data from 2006 onward and the data selection approach C. Green: 95th percentile of risk quotients below 1, orange: 95th percentile above 1, blue: no bioavailability correction parameters available, black: no data, and gray: non‐EU countries. EU, European Union
The importance of considering bioavailability, and gathering the necessary data to do so, is further illustrated through a more in‐depth investigation into the data for Spain (Figure 4) and Portugal (Figure 5). Spain has many sites, especially in the central part of the country, which cannot be assessed at tier 2 because the required parameters are lacking. These sites cannot be concluded to be at risk due to Cu exposures, as they cannot be fully assessed when implementing the appropriate procedures (EC, 2019). Furthermore, it can be observed that there are a relatively small number of sites where an exceedance of the EQSbioavailable is observed at tier 2 (31 out of 230 sites that could be assessed at tier 2), thus indicating that based on best practice, it cannot be concluded that Cu is an extensive risk in Spain. Most of these sites (28) are located in the region of Castilla y León, and the majority of sites have low DOC concentrations; this further highlights that targeted monitoring would be the most efficient use of resources rather than mandated nationwide monitoring. This also suggests that the use of the country‐specific 95th percentile concentration should not be the only metric of potential nationwide risks of a substance; for example, 24 of the 31 tier 2 exceedances are minor exceedances based on the calculated RQs (<1.5).
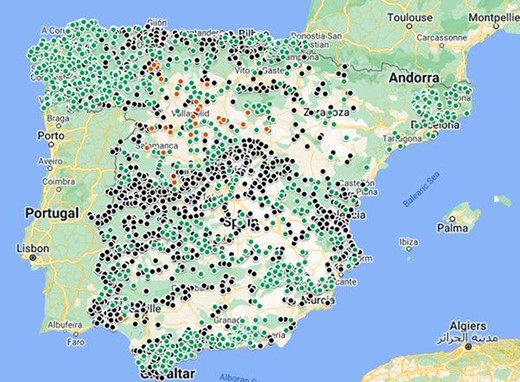
Risk assessment of copper in Spanish surface waters using site‐averaged data. Green: no risk identified at tier 1 or tier 2; black: potential risk identified at tier 1 but cannot be assessed at tier 2 due to missing data; and orange: potential risk identified at tier 2
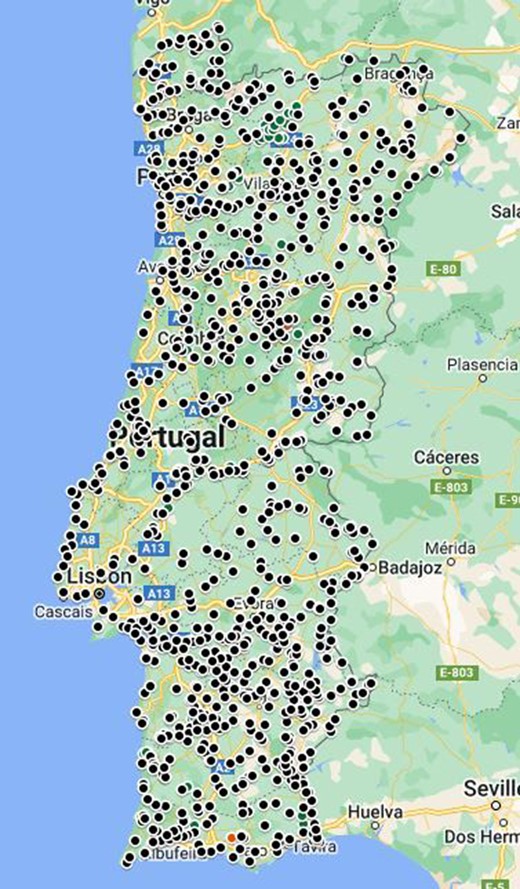
Risk assessment of copper in Portuguese surface waters using site‐averaged data. Green: no risk identified at tier 1 or tier 2; black: potential risk identified at tier 1 but cannot be assessed at tier 2 due to missing data; and orange: potential risk identified at tier 2
For Portugal, the majority of sites showing exceedances at tier 1 could not be assessed at tier 2 (1066 sites out of 1108). These sites could not be assessed due to either missing DOC data (2 sites), missing calcium data (104 sites), missing both DOC and calcium (912 sites), or missing pH data at 48 sites. Therefore, for Portugal, missing DOC and calcium data limit the application of bioavailability. Of the four sites that did show exceedances, there is no clear pattern of the exceedances (two in the District of Guarda, one in the District of Leiria, and one in the District of Faro; Figure 5), and the maximum exceedance has an RQ < 2. Therefore, based on the current best practice, Cu should not be classed as a country‐wide risk in Portugal, and additional expenditure should be made in collecting DOC and dissolved calcium data for sites that have tier 1 exceedances.
It is also important to note that not all European MS could be assessed as part of this assessment, in particular, with regard to accounting for bioavailability, as highlighted in Figure 3 and Table 1. Some countries could not be assessed due to a lack of available competent authority monitoring data, for example, Greece, or a lack of bioavailability‐supporting parameters, for example, Slovakia. With greater awareness of the latest EU guidance (EC, 2019), the lacking bioavailability parameters may be monitored more widely to be able to perform these assessments. It is to be noted that nickel already has an EU‐wide EQSbioavailable (European Parliament Council of the European Union, 2013), and therefore these data should already be collected to appropriately implement that EQS.
The analysis of time trends at two sites on two major European rivers (Rhine and Meuse) reveals that total and dissolved Cu concentrations have been declining over the past 50 years, although the decline has been most pronounced in the first two decades of monitoring. This may indicate that, following many years of emission–reduction measures, the concentrations in the environment are now tending toward ambient background concentrations and that further significant reduction in exposure levels may be difficult to achieve in major river systems. As part of the Forum of European Geological Surveys that was the European contribution to the UGS/IAGC Global Geochemical Baselines program, nine small drainage basins were sampled in 2000, and the geometric mean concentration dissolved Cu concentration was determined to be 2.03 µg L−1 (Salminen et al., 2005); the geometric mean concentrations at the Lobith and Eijsden sites were 2.09 and 1.82 µg L−1, respectively.
CONCLUSION
We have shown the importance of considering bioavailability when assessing the risks of Cu in the aquatic environment, as well as the importance of gathering the supporting data required to do so, if an assessment is to be ecologically relevant. If the scientific evidence is not followed and bioavailability is not accounted for, the assessment suggests widespread risks. Yet, by following the applicable European Commission guidance and by using a set of metrics that are consistent with the approach adopted by the European Commission (JRC, 2016, 2021), we have demonstrated that Cu is unlikely to pose a continent‐wide risk. In some countries, no conclusion could be drawn due to missing information. The evidence indicates that in some places, Cu may pose a local or regional risk (for example, Castilla y León in Spain).
Our data set consists of around 286 000 regulatory monitoring samples, of which around 90 000 had the supporting information to account for bioavailability. To allow for a more comprehensive bioavailability‐based risk assessment of metals in the aquatic environment, we encourage authorities to further increase measurements of DOC, which is the parameter that is most frequently lacking. Finally, a long‐term trend analysis in major European rivers, going back as far as 1970, shows a significant decrease in Cu concentrations that has been achieved over five decades due to reduced emissions to freshwaters.
AUTHOR CONTRIBUTION
Iain Wilson: Conceptualization; data curation; formal analysis; investigation; writing—original draft; writing—review and editing. Adam Peters: Conceptualization; formal analysis; investigation; writing—original draft; writing—review and editing. Graham Merrington: Formal analysis; investigation; writing—original draft; writing—review and editing. Stijn Baken: Conceptualization; data curation; writing—original draft; writing—review and editing.
ACKNOWLEDGMENT
The authors would like to thank the International Copper Association for funding this project. Additionally, we acknowledge the support of the Metals Environmental Research Association in assisting with the identification of some of the monitoring data used in the present study and to all the MS that provided data directly to the authors for inclusion in the database for this project. Iain Wilson, Adam Peters, and Graham Merrington are employees of a consultancy (WCA Environment Ltd.) that works for companies and regulatory and governmental organizations on projects focusing on the environmental risk assessment of chemicals. Stijn Baken works for an industry association (International Copper Association) representing companies that mine, smelt, and refine copper. The funding for this article was provided by the International Copper Association. Each author made substantial contributions to the conception, analysis, and interpretation of data and assisted in drafting the work. Each author approved the submitted version (and any substantially modified version that involves the author's contribution to the study) and agrees both to be personally accountable for the author's own contributions and to ensure that questions related to the accuracy or integrity of any part of the work, even ones in which the author was not personally involved, are appropriately investigated, resolved, and the resolution documented in the literature.
DATA AVAILABILITY STATEMENT
The raw data used for this assessment are provided as an additional file in the Supporting Information. bio‐met, the bioavailability correction tool utilized in this assessment, is publicly available and can be downloaded here: https://bio-met.net.