-
PDF
- Split View
-
Views
-
Cite
Cite
Adrian Ranga, Olivier Bouchot, Rosaire Mongrain, Patricia Ugolini, Raymond Cartier, Computational simulations of the aortic valve validated by imaging data: evaluation of valve-sparing techniques, Interactive CardioVascular and Thoracic Surgery, Volume 5, Issue 4, August 2006, Pages 373–378, https://doi.org/10.1510/icvts.2005.121483
- Share Icon Share
Abstract
The goal of this study has been to develop a numerical model of the aortic valve, to validate it with in-vivo data and to computationally evaluate the effect of two types of aortic valve-sparing reconstructions on valve dynamics and hemodynamics. A model of the native aortic valve and two models of the valve after surgical reconstruction (reimplantation with a straight conduit and remodeling with a shaped conduit) were created. These models were transferred to a finite element analysis software where the interaction between valve structures and blood was taken into account in a dynamic manner. Leaflet and blood dynamics, as well as tissue compliance and stresses were evaluated. Leaflet dynamics and blood velocities were also assessed by magnetic resonance imaging in 15 healthy volunteers. Computational results in the native valve model correlated closely with the in-vivo imaging data. The creation of neo-sinuses was shown to restore leaflet opening and closing dynamics. Loss of compliance at the commissures led to altered stress distribution patterns. Preservation of sinus geometry was an important factor in end systolic vortex formation. This is the first study to have incorporated the effect of blood flow in the numerical evaluation of aortic reconstructions using a computational model validated by in-vivo data. Differences in valve dynamics after surgical reconstruction reported in this computational study match trends previously reported in other in-vivo studies. Numerical models such as this one can serve as increasingly sophisticated tools in the study of aortic valve pathologies and in the optimization of new surgical reconstruction techniques.
1. Introduction
The aortic valve incorporates a number of features which allow it to fulfill its role in regulating the flow of blood over the course of the cardiac cycle. Its uniquely bulbous geometry, with three sinus spaces between each leaflet is thought to promote the creation of vortices helpful in initiating the closing phase, prevent the leaflet from hitting the root wall at systole, as well as optimize coronary flow. Its compliance allows it to expand at the commissures, thus optimizing the orifice area in systole and promoting a pull-back mechanism in diastole. When a diseased, dilated root pulls otherwise normal leaflets apart, an aortic valve-sparing procedure can be performed. A number of surgical variants of this procedure, such as the remodeling (Yacoub) and reimplantation (David) are currently practiced [1]. More recently, sinus-shaped prostheses have begun to be used. While short to mid-term clinical results have generally proven positive with most valve-sparing options [2], investigations on the effects of modified compliance and shape on leaflet dynamics, and possible implications on patient outcomes have been limited. In this study, we propose to use computational simulations to conduct such an evaluation. While recent computational studies have helped in the understanding of normal aortic valve function [3], none has studied the effects of different aortic root reconstructions on valve dynamics and associated hemodynamics with an emphasis on clinically available in-vivo validation modalities. In this work, a control and two surgically reconstructed valve models were created and analyzed. The intent was to first validate the control model by comparing computed valve characteristics with equivalent in-vivo magnetic resonance imaging (MRI) data obtained from healthy volunteers, and to then investigate the effect of two surgical reconstruction techniques on dynamic and hemodynamic functional parameters.
2. Methods
2.1. MRI
Fifteen healthy volunteers (10 men and 5 women aged 23–61 years; mean, 34 years) with no history of cardiovascular disease were evaluated by MRI velocity mapping. The study was approved by the Ethics Committee of the Montreal Heart Institute, and informed consent was obtained from all subjects. Experiments were performed on a Philips Intera CV 1.5T (Philips, the Netherlands), with measurements synchronized to the cardiac cycle using electrocardiogram gating. Three parameters were used to evaluate valve dynamics: rapid valve opening time (RVOT), rapid valve closing time (RVCT) and total ejection time (ET). RVOT was defined as the time interval between the initiation of leaflet opening and full valve opening, RVCT as the time interval between initiation of valve closure and full valve closure, and ET as the total time between initiation of valve opening and complete valve closure. Aortic blood flow was studied by MR flow velocity mapping with post-processing performed using MRI-FLOW software (Medis, Leesburg, VA, USA).
2.2. Computational
Three configurations were created and evaluated: a normal valve, a valve having undergone the remodeling procedure, and one having undergone a reimplantation procedure with a straight cylindrical graft.
The computer models developed here were based on parameters chosen from a survey of previously published data on the dimensions of the unpressurized aortic root [4–6] and on typical graft sizing during valve-sparing procedures, with dark regions in Fig. 1 representing tissue material and light regions representing graft material.
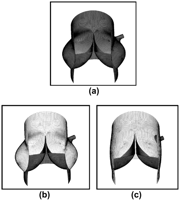
Meshes of the three models: normal (a), remodeling with shaped graft (b), reimplantation with straight conduit (c).
A novel approach was taken to impose fluid pressure using the LS-DYNA (LSTC, Livermore, CA, USA) finite element software. The fluid domain was modeled as a cylinder which fully enclosed the structural valve. At the inlet of the model, corresponding to the left ventricular outflow tract, the instantaneous pressure differential between these two regions was applied (Fig. 2 ).
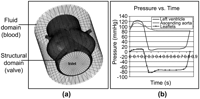
Structural valve model enclosed in the fluid mesh illustrating the pressure reservoir approach (a). Aortic, ventricular and leaflet pressure waveforms over a cardiac cycle (b).
3. Results
Three different simulations were run; commissural displacements, displacement at the tip of the leaflets, systolic stresses over the surface of the leaflets, and peak flow velocities at the center of the valve were recorded and analyzed. Validation was carried out for the valve structure based on leaflet dynamics, and on the blood flow based on flow velocities.
3.1. Morphological observations
The valve can be visualized in a sequence of ‘snapshots’ in time over the cardiac cycle (Fig. 3 ).
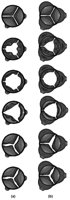
Valve behavior over the cardiac cycle in normal and straight conduit reimplantation models.
In the normal valve model the leaflets exhibited a smooth, billowing opening behavior aided by visible commissural expansion, leading to a rounded triangularized systolic shape. Conversely, the straight conduit model exhibited no visible motion at the commissures, and leaflets opening displayed an initially folded configuration, leading to a hexagonal opening shape at peak systole. These observations point to the complex computational and physiological phenomena occurring in these coupled simulations, where the close relationship of valve dynamics on hemodynamics is evidenced (Videos 1 and 2 ).
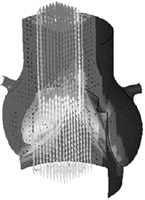
Simulations of the normal valve model over the full cardiac cycle.
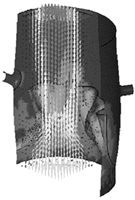
Simulation of the reimplantation model over the full cardiac cycle.
3.2. Root compliance
Root compliance (tracked at the commissures) in the normal model was close to physiologically reported values, decreased significantly (90.9%) in the remodeling case, while the reimplantation with straight conduit model exhibited nearly no compliance (Fig. 4 ).
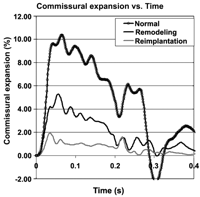
3.3. Leaflet dynamics
The same dynamic parameters evaluated in the MRI study (RVOT, RVCT and ET) were analyzed from the simulation results. Comparisons of the native valve model were made with MRI data from healthy volunteers (Figs. 5 and 6 ).
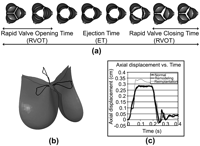
Representation of the phases of leaflet dynamics over the cardiac cycle (a), diagram of tracked directions for displacements and velocities (b), and plot of axial displacements of the leaflet tip over time (c).
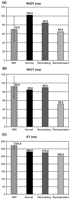
Comparison of leaflet dynamics parameters Rapid Valve Opening Time (RVOT) (a), Rapid Valve Closing Time (RVCT) (b) and ejection time (ET) (c) for in-vivo data set, native valve, remodeling and reimplantation models.
Simulation results indicated an overestimation of valve opening time as compared to the in-vivo results, with a value of 102.5 ms (compared to 70±27 ms in-vivo). Closing time was very well matched in the simulations (85 ms compared to 92±23 ms), and ejection time was underestimated (280 ms compared to 321±23 ms). The control case geometry provided a benchmark upon which to compare the remodeling and reimplantation cases. The general trend indicated that both types of surgical reconstruction decreased opening times and decreased closing time, which translated overall, into a shorter ejection time as compared to the normal valve. The remodeling procedure, which preserves a more physiological root geometry and compliance, evidenced dynamics which more closely matched those of the control model, while the reimplantation approach evidenced the most change. Comparing the two procedures, decreases of 30.8%, 41.7% and 8.2% in RVOT, RVCT and ET, respectively, are evidenced when going from a remodeling to a reimplantation procedure.
3.4. Leaflet stresses in systole
The state of stress in the tissue is an evaluative parameter which is not readily measured in-vivo. High mechanical stresses have been implicated as a potential mechanism for leaflet damage or failure, particularly when associated with notions of mechanical fatigue.
Stress distribution was significantly different between the normal and straight conduit models, with a well-localized region of high stresses at the attachment line in the former and a pattern oriented along the folds of the leaflet in the latter. Stress distribution patterns in the remodeling case were similar to those in the control case. While the peak overall stress (Von Mises) magnitudes were decreased in the surgically reconstructed cases (5.37×105 dynes/cm2 for remodeling, 5.67×105 dynes/cm2 for the reimplantation compared to 6.55×105 dynes/cm2 for the control), in the reimplantation case these stresses are transferred to a region of the leaflet which is perhaps more vulnerable to their effects. The compressive stress component in the reimplantation case was significantly increased with respect to the normal (1.37×105 dynes/cm2 vs. 1.01×105 dynes/cm2).
3.5. Blood velocities
In order to compare flow characteristics from the simulations to the in-vivo MR data, peak flow velocities at the level of leaflet coaptation were mapped over the cardiac cycle. Fig. 7(a) illustrates the close correspondence in evolution over time between in-vivo and computational results.
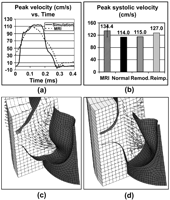
Plot of peak velocity over the cardiac cycle for a sample MRI-derived data set and normal valve model (a), comparison of maximal systolic velocities for MRI data set and computational models (b) and vortex and blunt velocity profile in remodeling (c) and reimplantation (d) cases, respectively.
Using again the normal model as a benchmark for comparing surgical reconstructions alternatives, the reimplantation modification induced an 11.4% increase in peak velocities at the valve (127.0 cm/s vs. 114.0 cm/s), while the remodeling procedure restored normal peak velocities (115 cm/s vs. 114.0 cm/s). It has also been shown that late systolic vortex regions in the sinuses may aid in closure of the normal valve and that there is an absence of such phenomena in the reimplantation model (Fig. 7c,d).
4. Discussion
In this study a close association was made between computed valve dynamics' parameters and those recorded in-vivo by MR imaging techniques. By validating the dynamic response of the control model with these clinical data, it was possible to ensure that subsequent computational models could then serve as a ‘virtual laboratory’ where the effect of changes in specific parameters such as aortic root materials and geometry could be isolated for investigation.
Aortic root compliance has long been known to affect valve function. Thubrikar and Robiscek investigated the effect of root stiffening in a left heart simulator [7]. They concluded that the compliance of the root serves to generate a ‘pull-and-release’ commissural function and contributes to a smooth, gradual and symmetric opening of the leaflets during systole and synchronous coordinated leaflet closure during diastole which was lacking in the stiffened roots. These experimental characterizations are in agreement with those of the current computational models.
A small number of studies using transesophageal echocardiography measurements of valve dynamics have focused on differences between valve-sparing approaches [8–10]. What has emerged from the current study, in combination with a critical and selective interpretation of the echocardiography data, is a clearer picture of normal leaflet dynamics and the influence of surgical reconstruction techniques in altering these. Root reconstructions which do not preserve root compliance and sinus geometry lead to an opening and closure which is more rapid both in time and in velocity. These altered dynamics may lead to clinical manifestations in the long-term, and may serve as one starting point in understanding the underlying causes of valve damage initiation from a mechanical point of view.
Indeed, it was shown in this study that the lack of sinuses induced complex bending in the belly of the leaflets leading to an altered stress distribution pattern, with peak stresses in the reimplantation model no longer located at the attachment line but within the belly, a region of the leaflet perhaps less able to bear load. Compressive stresses were also higher in the reimplantation model, which, combined with the shift in stress location, may have an implication in long-term fatigue deterioration of the leaflet.
Only two studies have focused on the evaluation of aortic valve-sparing techniques using MRI. In one study [11], late systolic vortex formation was reported in the normal cases, but was undetectable in the reconstructed valve patients, while in another [12], vorticity was observed in all reconstructions, whether with a shaped or straight graft. Our numerical results indicate that there is little full vortex formation in the straight conduit reimplantation at peak systole, where there is limited space for these to develop, and that closure is initiated by a flow pattern exhibiting a blunter profile than in the remodeling and control models. This characteristic of late systolic flow may serve as an explanation for the more rapid valve closure seen in the reimplantation model.
A number of limitations in the current work are inherent in attempting to model complex biological systems. Indeed, the constructed geometries are based on approximated average values of key dimensions, and do not take into account natural variability. Using MRI data from healthy patients to validate the model also highlights the importance of matching input conditions between the simulations and in-vivo data in order to obtain close correlations. Finally, validation was only carried out for the control model with data from healthy volunteers. While further validation with a similar data set from a surgically reconstructed case would be helpful in assessing the validity of our findings, our results matched the trends reported in other in-vivo studies.
5. Conclusion
This is the first study to have incorporated blood hemodynamics in the numerical evaluation of aortic reconstructions. A number of parameters which have helped quantify differences between different valve-sparing alternatives were analyzed Results indicated that from a biomechanical and hemodynamic point of view the remodeling aortic root reconstruction procedure is closer to the natural aortic valve than the reimplantation approach, proving better at restoring valve dynamics and flow patterns to their normal state. Numerical models such as this one can serve as increasingly sophisticated tools in the study of aortic valve pathologies, in the optimization of new surgical reconstruction techniques and in prosthetic device design. The current goal for these types of simulations will be to use them for evaluation and surgical guidance on a patient-by-patient basis.
Presented at the joint 19th Annual Meeting of the European Association for Cardio-thoracic Surgery and the 13th Annual Meeting of the European Society of Thoracic Surgeons, Barcelona, Spain, September 25–28, 2005.
This work was funded by the Natural Sciences and Engineering Research Council of Canada (NSERC) and by CoroNeo Inc, Montreal, Canada. A. Ranga is currently employed at CoroNeo Inc. The other authors have no financial relationships with CoroNeo Inc.
References
Appendix. Conference discussion
Dr T. David (Toronto, Canada): That is a very elegant computer modeling of the aortic root. Unfortunately your conclusions bear no relationship with clinical outcomes. We now have 18 years' experience with aortic valve sparing operation. The only difference in clinical outcomes between the remodeling procedure and reimplantation of the aortic valve is a higher incidence of moderate aortic insufficiency in patients who had the remodeling procedure. We learned some 5 or 6 years and have since abandoned the remodeling procedure altogether.
Aortic root aneurysm is often a genetic disorder and the expression of the genes that cause dilatation is temporal. The operation is entirely incidental. It depends largely on the size of the sinuses. The bigger the sinuses the more likely we are to intervene with or without aortic insufficiency. We learned that a more perfect geometric operation is not necessarily a more durable operation.
I have to tell you that during the first 10 patients I used only a straight tube. Those patients have now passed the 15 years' durability; valve function hasn't changed at all in 15 years. Actually the longest one is now at 18 years and she has normal aortic valve function in a straight tube.
This doesn't mean that we should not change the operation. Over the years, like you showed, we have shown by echo that the present sinuses decrease the velocity of closure of the cusps. One would expect that a lower velocity likely correlates to lower stress. Unfortunately I cannot show that clinically yet, but it might take 40 years to show it.
Mr Ranga: That is an interesting perspective. I think perhaps one of the reasons you have shown great success is excellent technical skills. What we are demonstrating here is that perhaps when everything is taken into account in a very similar way, very controlled conditions, we do have mechanical effects that come into account. Your long-term data is very interesting, but we are still wondering perhaps even at a longer term whether some of these mechanical effects do have some kind of influence. And it is true, you have great results, but it would be interesting to see what these mechanical effects have on a longer term.
Dr David: As I mentioned, I think you are in the right direction and we need more science and but also more clinical experience. However, the fact remains that reimplantation of the aortic valve so far has given the most durable results for patients with aortic root aneurysm, which makes me wonder if the aortic cusps, since they are living structures, they are not able to adjust to the adverse environment. Just like when we do the Ross procedure, a proportion of pulmonary valves do not tolerate systemic pressure and fail; in our experience at 15 years about 10% have failed. The native aortic valve perhaps may have an intrinsic ability to adapt itself to a more stressful environment such as a rigid aortic root. It might explain why the aortic valve does not fail in hypertensive patients, for instance.
Dr M. Thubrikar (Irvine, California): About two years ago I organized a special symposium on aortic valve sparing and several surgeons were there who routinely perform the valve-sparing operation. I think, Dr Ranga, what you are doing is just excellent, and this is what the computational people and engineers do because they are not directly working with patients. There are a couple of interesting things here. The valve-sparing operation needs to have science in it. That science needs to be brought into it and it is still waiting, as Dr David pointed out.
What I found out from the symposium is that one of the key understanding necessary in the valve-sparing, is that there is a change in the leaflet length free edge. As the root dilates, the leaflets stretch out and the proportionality of the leaflet dimension, the free edge to the width ratio, changes. And during surgery, no one measures that proportionality and no one necessarily corrects for it, and they don't also know how to correct for it in a scientific mathematical way.
So what came out of that symposium was that if one could use the MRI or CT and have the actual dimensions of the root and the leaflet and then be able to use the corrections, which would include the leaflet free edge correction if necessary, that would work the best. That is the science being called for in the valve-sparing, particularly with regards to the immediate need of the patient.
Mr Ranga: That is an interesting comment. One of the things you have to understand about the current state of these numerical simulations and their value is that they are what I call all things considered equal type simulations. We are controlling everything; every aspect in this case is controlled and controllable. So the free edge length that you were discussing, for example, in these simulations is the same. That is a parameter that can be varied. All of these things can be used as future investigations.
What is demonstrated here is that when all things are considered the same, then you exhibit these types of phenomenon. So it is interesting to sort of have that feel for the motivation behind this.
Using MRI data as an input will give us a case-by-case, patient-by-patient information, which will be valuable in that respect but will then be specific. This we feel is a very general exposition of these various phenomenon.
Dr O. Yadava (New Delhi, India): I am a clinical cardiac surgeon, and this modeling looks like a very complex thing. The thing that I really want to know to bring it to clinical application, is there any validation of your numerical modeling or computer modeling or mathematical modeling, whatever you call it? Has it been validated against other modalities of investigation?
Mr Ranga: Yes, that is exactly what we showed today with the MRI data. We validated the dynamics of the leaflets and, as well, the velocity profile over the cardiac cycle, and we showed some rather good correlation despite some of the assumptions inherent in these models. Again, inputting clinical imaging data into these models we believe will bring even closer validation, but this is the first step in that process.