-
PDF
- Split View
-
Views
-
Cite
Cite
Dong Woog Yoon, Tae Ho Kim, Min Jae Cha, Gun-Hee Kim, ByoungSoo Lee, Hyung Giun Kim, Minhyeok Kwon, Donghoon Jang, Byungjoon Park, Three-dimensional printed pure-titanium implantation for chest wall reconstruction involving the sternum and ribs: a novel approach, Interdisciplinary CardioVascular and Thoracic Surgery, Volume 38, Issue 4, April 2024, ivae037, https://doi.org/10.1093/icvts/ivae037
- Share Icon Share
Abstract
Chest wall reconstruction is challenging due to the complex shape and large defect size. The three-dimensional printing technology enables the fabrication of customized implants, and 3D-printed pure-titanium could provide superior mechanical properties to conventional materials. The aim of this study was to evaluate long-term outcomes of patients undergoing chest wall reconstruction with a 3D-printed pure-titanium implant. Between August 2018 and May 2021, 5 patients underwent surgery due to sternal metastasis (n = 3), postoperative sternal wound infection (n = 1) and deformity (n = 1). The customized implant was designed and constructed based on the size and shape of the chest wall defect measured on computed tomography. All patients demonstrated uneventful recovery without complications during the hospital course. During the median follow-up of 20 months, 1 patient underwent revision surgery due to implant breakage, and 1 removed the implant due to trauma-related chest wall infection. One patient died from cancer progression, while 3 patients are alive without any implant-related complications. Chest wall reconstruction using a 3D-printed pure-titanium implant could be a novel alternative for patients with various conditions affecting the sternum and ribs.
INTRODUCTION
Chest wall reconstruction (CWR) surgery is a necessary procedure for addressing a variety of conditions affecting the sternum, parasternal cartilage and ribs [1]. Traditionally, rigid or non-rigid reconstruction using titanium alloys, bone cement or polypropylene with mesh has been commonly used for CWR. However, the chest wall (sternum and ribs) presents a wide range of reconstruction areas and complex shapes, making effective reconstruction challenging [2]. Moreover, the use of titanium alloy implants for CWR can result in various complications, such as breakage and infection [3]. Additionally, corrosion or wear of titanium alloys can result in the release of vanadium and aluminium particles that may be harmful to the body [4].
In recent years, the emergence of three-dimensional printing (3DP) technology has brought significant advancements to the field of CWR, allowing for the development of customized implants tailored to individual patients. Various kinds of advanced chest wall implants have been reported in the literature [5–7]. These personalized implants, which were previously unavailable in standard ready-made commercial options, have shown promising results: reduced surgical time, rapid adaptation to postoperative movements, and potentially fewer complications, leading to improved quality of life [6, 7]. However, there is a lack of reported long-term outcomes for patients undergoing CWR using 3DP implants, and there has been limited research on the optimal choice of materials and 3DP techniques to achieve the best results for patients requiring CWR.
In this pilot study, we aimed to elucidate the long-term outcomes of patients who underwent CWR with a 3DP pure-titanium (3DP-pTi) implant. Additionally, this article discusses the iterative process involved in the development of the 3DP-pTi implant. To the best of our knowledge, this article represents the first report on the clinical outcomes of patients who underwent CWR with a 3DP-pTi implant.
MATERIALS AND METHODS
Study population
From August 2018 to May 2021, patients undergoing CWR with 3DP-pTi at Chung-Ang University Hospital in Seoul, Korea, were enrolled. The inclusion criteria involved patients needing extensive CWR due to malignancy involving the sternum, sternal defects or distortions resulting from post-sternotomy sternal infections, or complicated congenital chest wall disorders. Follow-up data were obtained from the patient's most recent records, and the modified medical research council (mMRC) dyspnoea scale and pulmonary function were evaluated, including forced vital capacity (FVC) and forced expiratory volume in 1 s (FEV1) at 6 months postoperatively. The institutional review board of Chung-Ang University Hospital approved this study (IRB number 2301-017-539), and the study was underway after obtaining informed consent from each patient.
Manufacturing the patient-specific 3DP-pTi implant
The 3DP-pTi implant, made of commercially pure Ti (pTi), was manufactured by Cubelabs, Inc., Seoul, Korea. To achieve optimal implant design in all cases, we measured the extent of resection and reconstruction precisely to guide the production of the implants and conducted 1:1 simulation for each case (Fig. 1 and Supplementary Material, Fig. S1). Measurement was based on the extraction of 2D images from computed tomography scans with a thickness of 1 mm, followed by bone segmentation and 3D image reconstruction. To achieve symmetry, we determined the size of the implant by referencing the opposite side. Alternatively, when the opposite side was affected, the implant sizing was guided by visually imaging a normal bone shape. The design process, lasting 2 to 3 days, is followed by 3D printing, heat treatment, sanding, post-processing, washing, drying, and packaging, which collectively take an additional 10–14 days after design confirmation.
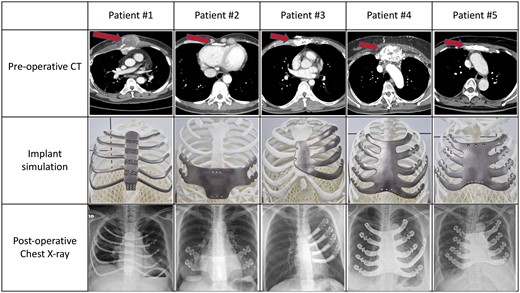
Preoperative findings, implant simulation, and postoperative chest X-ray of study participants.
The 3D printing technique initially employed electron beam melting (EBM) for the first patient and then transitioned to selective laser melting (SLM) for subsequent patients. For the first patient, a personalized artificial rib cage was designed using stacked 2D computed tomography images taken from the patient. In particular, the sternum was designed with a lattice structure to lower the elastic modulus, and an elliptical design for the ribs was applied to increase the mechanical strength. An EBM was employed using an Arcam A2X (Sweden) for metal 3D printing of the designed artificial rib cage. Commercially pTi grade 2 (ASTM F67) powder from Arcam was used as the raw material, which had a perfectly spherical shape with an average size of 73 μm. For other patients, SLM was employed using an SLM280 (Germany) with commercially pTi grade 2 (ASTM F67) powder from SLM Solutions, which had a perfectly spherical shape with an average size of 41 μm. Afterward, a heat treatment was conducted at 590°C for 2 h in a 10–5 torr vacuum furnace to relieve thermal residual stress.
Mechanical properties of the 3DP-pTi implant
The 3DP-pTi exhibited superior strength and elongation rate compared with standard pTi grade 2 (Supplementary Material, Table S1). In order to ascertain the structural integrity of an implant in a cardiopulmonary resuscitation scenario, where the average contact area is 16 cm2 with a force of 556.22 N, measurements were conducted to determine both the maximum stress and stiffness during PCR scenario (Supplementary Material, Table S2).
Surgical technique
The surgical approach involved either a sternotomy or bilateral anterior thoracotomy (clamshell incision). Meticulous dissection was performed along the sternum, ribs and muscles. Any affected bones or prostheses occupying the reconstruction area were resected, and the bone margins were carefully trimmed. Following thorough irrigation of the reconstruction site, a 3DP-pTi implant was inserted. To ensure stability, the Sternal ZipFix (Synthes GmbH, Oberdorf, Switzerland) system, which uses the cable-tie principle and is composed of PEEK (polyetheretherketone), was used for fixation.
RESULTS
Five patients (2 men and 3 women) with a median age of 55 years (interquartile range, 37, 59.5) underwent CWR using 3DP-pTi implants. The median operation time was 320 min (interquartile range, 292.5, 687.5). Patients’ characteristics and the perioperative data are summarized in Table 1. The perioperative recovery was uneventful.
. | Preoperative . | Perioperative . | 6-month follow-up . | Outcome . | ||||||||||||
---|---|---|---|---|---|---|---|---|---|---|---|---|---|---|---|---|
. | Age/Sex . | BMI . | Etiology . | mMRC . | FVC (l) . | FEV1 (l) . | Concomitant surgery . | Op. time (min) . | Perioperative event . | Discharge . | mMRC . | FVC (l) . | FEV1 (l) . | F/U period (months) . | Implant outcome . | Clinical outcome . |
Patient #1 | M/55 | 29.1 | Sternal invasion of undifferentiated pleomorphic sarcoma arising from right pectoralis muscle | 1 | 3.63 | 2.94 | Lung resection, latissimus dorsi muscle flap | 830 | None | POD 21 | 1 | 3.06 | 2.43 | 20 | Uneventful | Death due to cancer progression |
Patient #2 | F/42 | 18.1 | Sternal defect (lower ⅓ of sternum) due to post-sternotomy wound infection | 0 | 3.02 | 2.41 | Scar revision (department of plastic surgery) | 320 | None | POD 9 | 0 | 2.87 | 2.27 | 46.7 | Uneventful | Well-doing |
Patient #3 | M/32 | 19.1 | Sternal distortion after recurrent surgery for pectus carinatum | 0 | 4.25 | 3.54 | None | 315 | None | POD 11 | 0 | 4.15 | 3.40 | 15.2/22a | Implant revision due to implant breakage | Well-doing |
Patient #4 | F/58 | 23.3 | Sternal metastasis from renal cell carcinoma | 1 | 2.5 | 1.91 | None | 545 | None | POD 35 | N/A | N/A | N/A | 3.1 | Implant removal due to trauma-related infection | N/A |
Patient #5 | F/61 | 22.9 | Sternal metastasis from breast cancer | 1 | 3.36 | 2.66 | None | 270 | None | POD 9 | 2 | 1.42 | 1.24 | 28.7 | Uneventful | Cancer progression with lung involvement |
. | Preoperative . | Perioperative . | 6-month follow-up . | Outcome . | ||||||||||||
---|---|---|---|---|---|---|---|---|---|---|---|---|---|---|---|---|
. | Age/Sex . | BMI . | Etiology . | mMRC . | FVC (l) . | FEV1 (l) . | Concomitant surgery . | Op. time (min) . | Perioperative event . | Discharge . | mMRC . | FVC (l) . | FEV1 (l) . | F/U period (months) . | Implant outcome . | Clinical outcome . |
Patient #1 | M/55 | 29.1 | Sternal invasion of undifferentiated pleomorphic sarcoma arising from right pectoralis muscle | 1 | 3.63 | 2.94 | Lung resection, latissimus dorsi muscle flap | 830 | None | POD 21 | 1 | 3.06 | 2.43 | 20 | Uneventful | Death due to cancer progression |
Patient #2 | F/42 | 18.1 | Sternal defect (lower ⅓ of sternum) due to post-sternotomy wound infection | 0 | 3.02 | 2.41 | Scar revision (department of plastic surgery) | 320 | None | POD 9 | 0 | 2.87 | 2.27 | 46.7 | Uneventful | Well-doing |
Patient #3 | M/32 | 19.1 | Sternal distortion after recurrent surgery for pectus carinatum | 0 | 4.25 | 3.54 | None | 315 | None | POD 11 | 0 | 4.15 | 3.40 | 15.2/22a | Implant revision due to implant breakage | Well-doing |
Patient #4 | F/58 | 23.3 | Sternal metastasis from renal cell carcinoma | 1 | 2.5 | 1.91 | None | 545 | None | POD 35 | N/A | N/A | N/A | 3.1 | Implant removal due to trauma-related infection | N/A |
Patient #5 | F/61 | 22.9 | Sternal metastasis from breast cancer | 1 | 3.36 | 2.66 | None | 270 | None | POD 9 | 2 | 1.42 | 1.24 | 28.7 | Uneventful | Cancer progression with lung involvement |
BMI: body mass index; F: female; FEV1: forced expiratory volume in 1 s; FVC: forced vital capacity; M: male; mMRC: modified Medical Research Council dyspnoea scale; N/A: not available; POD: post-operative day.
Follow-up period after revision surgery.
. | Preoperative . | Perioperative . | 6-month follow-up . | Outcome . | ||||||||||||
---|---|---|---|---|---|---|---|---|---|---|---|---|---|---|---|---|
. | Age/Sex . | BMI . | Etiology . | mMRC . | FVC (l) . | FEV1 (l) . | Concomitant surgery . | Op. time (min) . | Perioperative event . | Discharge . | mMRC . | FVC (l) . | FEV1 (l) . | F/U period (months) . | Implant outcome . | Clinical outcome . |
Patient #1 | M/55 | 29.1 | Sternal invasion of undifferentiated pleomorphic sarcoma arising from right pectoralis muscle | 1 | 3.63 | 2.94 | Lung resection, latissimus dorsi muscle flap | 830 | None | POD 21 | 1 | 3.06 | 2.43 | 20 | Uneventful | Death due to cancer progression |
Patient #2 | F/42 | 18.1 | Sternal defect (lower ⅓ of sternum) due to post-sternotomy wound infection | 0 | 3.02 | 2.41 | Scar revision (department of plastic surgery) | 320 | None | POD 9 | 0 | 2.87 | 2.27 | 46.7 | Uneventful | Well-doing |
Patient #3 | M/32 | 19.1 | Sternal distortion after recurrent surgery for pectus carinatum | 0 | 4.25 | 3.54 | None | 315 | None | POD 11 | 0 | 4.15 | 3.40 | 15.2/22a | Implant revision due to implant breakage | Well-doing |
Patient #4 | F/58 | 23.3 | Sternal metastasis from renal cell carcinoma | 1 | 2.5 | 1.91 | None | 545 | None | POD 35 | N/A | N/A | N/A | 3.1 | Implant removal due to trauma-related infection | N/A |
Patient #5 | F/61 | 22.9 | Sternal metastasis from breast cancer | 1 | 3.36 | 2.66 | None | 270 | None | POD 9 | 2 | 1.42 | 1.24 | 28.7 | Uneventful | Cancer progression with lung involvement |
. | Preoperative . | Perioperative . | 6-month follow-up . | Outcome . | ||||||||||||
---|---|---|---|---|---|---|---|---|---|---|---|---|---|---|---|---|
. | Age/Sex . | BMI . | Etiology . | mMRC . | FVC (l) . | FEV1 (l) . | Concomitant surgery . | Op. time (min) . | Perioperative event . | Discharge . | mMRC . | FVC (l) . | FEV1 (l) . | F/U period (months) . | Implant outcome . | Clinical outcome . |
Patient #1 | M/55 | 29.1 | Sternal invasion of undifferentiated pleomorphic sarcoma arising from right pectoralis muscle | 1 | 3.63 | 2.94 | Lung resection, latissimus dorsi muscle flap | 830 | None | POD 21 | 1 | 3.06 | 2.43 | 20 | Uneventful | Death due to cancer progression |
Patient #2 | F/42 | 18.1 | Sternal defect (lower ⅓ of sternum) due to post-sternotomy wound infection | 0 | 3.02 | 2.41 | Scar revision (department of plastic surgery) | 320 | None | POD 9 | 0 | 2.87 | 2.27 | 46.7 | Uneventful | Well-doing |
Patient #3 | M/32 | 19.1 | Sternal distortion after recurrent surgery for pectus carinatum | 0 | 4.25 | 3.54 | None | 315 | None | POD 11 | 0 | 4.15 | 3.40 | 15.2/22a | Implant revision due to implant breakage | Well-doing |
Patient #4 | F/58 | 23.3 | Sternal metastasis from renal cell carcinoma | 1 | 2.5 | 1.91 | None | 545 | None | POD 35 | N/A | N/A | N/A | 3.1 | Implant removal due to trauma-related infection | N/A |
Patient #5 | F/61 | 22.9 | Sternal metastasis from breast cancer | 1 | 3.36 | 2.66 | None | 270 | None | POD 9 | 2 | 1.42 | 1.24 | 28.7 | Uneventful | Cancer progression with lung involvement |
BMI: body mass index; F: female; FEV1: forced expiratory volume in 1 s; FVC: forced vital capacity; M: male; mMRC: modified Medical Research Council dyspnoea scale; N/A: not available; POD: post-operative day.
Follow-up period after revision surgery.
The postoperative courses are summarized in Table 1. The median follow-up period was 20 months (interquartile range, 5.15, 28.7). The pulmonary function test conducted 6 months after surgery showed normal to mildly decreased FVC and FEV1, and the subjective dyspnoea scale based on mMRC showed no change except for 1 patient with cancer progression involving the lung. During the postoperative follow-up, 2 cases of implant problems occurred. Implant breakage was observed in a young male patient (patient #3) who had an otherwise uneventful recovery. The breakage occurred 10 months postoperatively, coinciding with a period during which the patient engaged in weight training activities involving excessive load (Supplementary Material, Fig. S2A). Upon the diagnosis of the implant breakage, the decision was made to change the implant thickness in the rib area from 1 to 2 mm. Revision surgery was performed to replace the damaged implant, and during the subsequent 20-month follow-up period, the patient exhibited no symptoms, abnormalities, or complications. Patient #4, who had a favourable postoperative recovery, developed an implant infection following an anterior chest wall injury sustained in a traffic accident 3 months postoperatively. (Supplementary Material, Fig. S2B) Despite repeated irrigation procedures, the infection could not be controlled, necessitating the removal of the implant. By the time of the most recent follow-up, 1 patient (patient #1) had died from cancer progression and 3 patients (patients #2, #3 and #5) were alive without any implant-related complications.
DISCUSSION
In this study, we used 3DP technology using pTi. In the mid-1950s, pTi initially gained recognition as an ideal material for CWR due to its optimal strength, corrosion resistance, and biocompatibility. With pTi graded into 4 levels, higher grades offer increased strength but reduced brittleness, making grade 2 pTi the preferred choice for CWR. However, its lower strength led to reported issues of early prosthesis fractures. In this study, using 3DP technology, we improved the mechanical properties of grade 2 pTi to facilitate its use as an implant. Our 3DP-pTi demonstrated higher yield strength, tensile strength, and elongation compared to standard grade 2 pTi. The utilization of pTi alleviated concerns regarding the potential release of harmful particles, a concern often associated with titanium alloys. Furthermore, although metal 3DP is typically influenced by operator dependency, our study successfully addressed these challenges through our technological expertise, producing high-quality, large pTi implants.
During the study period, there was a transition in the 3DP methodology employed. When manufacturing implants for CWR, it is crucial to address the specific requirements of small-scale, intricate features like screw holes, suture holes and sections where cable ties are affixed. Additionally, given the proximity of vital organs and the imperative of preserving surrounding tissues, achieving optimal surface roughness becomes pivotal. Consequently, we evaluated 2 distinct 3DP techniques: EBM and SLM. These methods differ in terms of energy output and titanium powder particle size, with SLM-based systems showcasing advantages in terms of compactness, precision and superior surface roughness [8]. As a result, beginning with patient #2, we adopted SLM for the fabrication process.
The method of implant fixation also underwent modification during the study period. Initially, screw fixation was employed in patient #1, but postoperative follow-up of serial chest X-ray observations revealed instances of screw loosening. The problem of late screw loosening after implant fixation has been a recurring problem reported by other centres [9]. In response, starting from patient #2, we adopted the Sternal ZipFix system, which uses the cable-tie principle and is composed of PEEK, for implant fixation [10]. Subsequently, during a follow-up period of >2 years for the study participants, we observed stable and secure implant fixation.
During the postoperative recovery period after CWR surgery, it is crucial to maintain chest wall stability while preserving breathing mechanics to restore respiratory function effectively. A previous study investigating 3DP implants demonstrated a decrease in FVC postoperatively, but no significant differences were observed in the FEV1/FVC ratio [6]. In our study, decreased lung function in 2 patients (patients #1 and #5) were observed. Patient #1 had undergone a concomitant lung resection procedure, and both patients were diagnosed with stage IV cancer, suggesting that the decline in lung function was attributed to cancer progression. On the other hand, among the remaining 2 patients (excluding patient #4, who underwent implant removal), no significant changes in pulmonary function were observed.
Implant breakage and implant infection are the 2 major complications associated with implant-based CWR [3]. In this study, 1 case of breakage and 1 case of infection were observed. As described in the ‘Materials and Methods’ section, despite designing the implants to withstand the load imposed during chest compressions in cardiopulmonary resuscitation, breakage occurred at the site near where the implant was connected to the ribs. Although excessive weight training was identified as a potential cause, we increased the thickness of the rib area of the implants to 2 mm after this complication to mitigate similar occurrences. Subsequently, no further breakage events have been reported. The case involving implant removal due to infection was an event resulting from a car accident, which occurred in an immunocompromised patient undergoing chemotherapy for renal cell carcinoma. While the incidence of complications in 2 out of the 5 patients appears relatively high, it is regarded as part of the trial-and-error process inherent in the development of new implants. A future confirmatory study involving a larger number of patients is warranted.
Limitations
This study has several limitations. First, the study had a limited sample size of 5 patients and did not include a comparison with existing methods for CWR. Second, efforts should be made to reduce the preparation time, particularly for highly aggressive tumours, as the current process of manufacturing the prosthesis takes several weeks. Third, the potential impact of metal artefacts from the 3DP-pTi implant should be taken into consideration, as they may pose difficulties in the accurate evaluation of local tumour recurrence.
CONCLUSION
In conclusion, CWR using a 3DP-pTi implant could be a novel alternative for patients with various conditions affecting the sternum and ribs. This preliminary study provided valuable information, but further confirmatory studies are required to validate these findings.
SUPPLEMENTARY MATERIAL
Supplementary material is available at ICVTS online.
FUNDING
This research was supported by the Chung-Ang University Research Grants in 2023.
Conflict of interest: none declared.
DATA AVAILABILITY
The data underlying this article will be shared on reasonable request to the corresponding author.
Reviewer information
Interdisciplinary CardioVascular and Thoracic Surgery thanks Yuji Shiraishi, Mohamed Rahouma, Angelo Carretta, Amr A Arafat and the other anonymous reviewer for their contribution to the peer review process of this article.