-
PDF
- Split View
-
Views
-
Cite
Cite
Baolei Guo, Riaz Akhtar, Haofei Liu, Cheng Yan, Daqiao Guo, Benjamin Patterson, Weiguo Fu, Staged surgically created type B aortic dissection model with endovascular reintervention for different morphological features, Interdisciplinary CardioVascular and Thoracic Surgery, Volume 37, Issue 5, November 2023, ivad133, https://doi.org/10.1093/icvts/ivad133
- Share Icon Share
Abstract
Understanding morphology and how this relates to treatment strategy is critical for achieving remodelling in aortic dissection. A controllable and reproducible large animal model is required for investigating new therapeutic devices and interventions.
Our experimental protocol involved the development of surgically created type B aortic dissection (TBAD) and endovascular reintervention-induced TBAD porcine models. The sample was randomly divided into 2 groups: 1 underwent a secondary tear creation (STC) procedure and the other underwent a false lumen extension (FLE) procedure. Anatomical features were observed at 1 and 3 months, and 2 animals in each group were euthanized at 3 months after the procedures. The aorta and main branches were harvested en bloc, cross-sectioned and prepared for histological examination.
All surgically created TBAD models were successfully generated, and no unintended complications occurred. The endovascular reintervention-induced TBAD model was successfully created in 11 of 12 animals, with 6 in the STC group and 5 in the FLE group. In the STC group, the intraoperative mean diameter of the new secondary tear was 7.23 mm, and a slight increase was observed at first 30 days (P = 0.0026). In the FLE group, the intraoperative new propagation length was (235.80 ± 84.94) mm. The FL propagation length at the 1-month follow-up was significantly longer than that measured intraoperatively (P = 0.0362). Histological evaluation demonstrated that the elastic fibres in the media layer of the aortic wall were disrupted and appeared to be significantly stretched on the adventitial side of the false lumen.
Our endovascular reintervention is a reliable, minimally invasive approach for producing specific TBAD models with different morphologies.
INTRODUCTION
Endovascular thoracic aortic repair (TEVAR) has become the first-line treatment for patients with complicated, and to an increasing extent, uncomplicated type B aortic dissection (TBAD) [1, 2]. Some of these patients require additional treatment after the index TEVAR due to an endoleak, endograft infection, distal aortic degeneration or endograft material deterioration [3]. The morphology of each dissection is heterogeneous, exhibiting variation in propagation length, number of secondary tears and true lumen (TL) and false lumen (FL) volume. Addressing these morphological features is crucial for achieving optimal remodelling and therefore informs treatment strategy. Therefore, the success of TEVAR alone in sealing the primary entry tear (PET) is dependent on the underlying haemodynamic mechanisms, morphology and how these can be altered by intervention [4, 5].
A validated and reproducible large animal aortic dissection model with different anatomical morphologies for investigating new interventions could improve the current knowledge in this realm. The Food and Drug Administration has made recommendations that new models are needed not only to replicate biological conditions encountered over time (in the acute and subacute phases of the disease) but also to assess the effectiveness of new devices [6]. Although several animal models have previously been proposed [7–10], the majority did not attempt to study the morphological characteristics of TBAD in vivo. Focused has mainly been on establishing an animal model of the biological mechanism underlying TBAD. Furthermore, previous open surgical techniques for creating a porcine model involved aortic cross-clamping and pentobarbital which led to a low animal survival rate [9].
In our previous study, we reported the successful creation of an open surgical porcine TBAD model and found that the depth of the intimal tear had a significant direct influence on the extent of propagation of the dissection plane [10]. Based on previous knowledge of porcine TBAD and anaesthesia, we aimed to describe a novel animal model of TBAD with morphology analogous to human disease. This could subsequently be used in the development and evaluation of new imaging, pharmacological and interventional strategies.
MATERIALS AND METHODS
Ethics statement
The study was approved by the Institutional Animal Care and Use Committee of Zhongshan Hospital Fudan University, China (approval reference number Y2021-072). Experiments were performed at a medical innovation centre in strict accordance with the principles of laboratory animal care advocated by the animal experiment committee. The animals were kept in an animal care facility with ad libitum feeding for an acclimatization period of 14 days. All in vivo experiments reported in this study followed the NC3Rs guidelines [11].
Animal care
A total of 12 Shanghai Landrace pigs with an average weight of 67.58 ± 7.43 kg and a mean age of 4.4 ± 0.6 months were used in the study. An intramuscular injection of ketamine (15 mg/kg) and atropine sulphate (0.04 mg/kg) was used to sedate each animal. General anaesthesia was induced with isoflurane (5%) administered via a face mask and a target-controlled infusion of remifentanil at 4 ng/ml. Rocuronium was given at 0.5 mg/kg to facilitate endotracheal intubation. Anesthesia was then maintained with isoflurane (1.5–3%), oxygen (0.8–1.5 l/min) and mechanical ventilation.
Experimental protocol
Surgically created type B aortic dissection model
The surgically created TBAD (SC-TBAD) porcine model was performed surgically via a thoracotomy first. According to previously published methods for establishing SC-TBAD porcine models [10], 12 models were successfully created with the primary dissection level located in the middle of the media. Follow-up observations were performed by computed tomography angiography (CTA) within 2–4 weeks after the procedures. Anatomical features of the aortic dissection were measured by CTA imaging. Then, the animal models were randomly divided into 2 groups in a 1:1 ratio; 1 group (n = 6) underwent the secondary tear creation (STC) procedure, and the other group (n = 6) underwent the false lumen extension (FLE) procedure.
Endovascular reintervention-induced type B aortic dissection model
Prophylactic amoxicillin/clavulanate (antibiotic) was administered half an hour before the invasive procedures. The experiment took place in a research hybrid operating room equipped with an international radiology system (Optima IGS 330, GE Healthcare). Invasive blood pressure monitoring was obtained using left femoral artery access. A stab incision was made in the right groyne, and an introducer sheath (7 Fr) was inserted via the femoral artery. The right common carotid artery (CCA) was exposed through a median neck incision under sterile conditions and cannulated with an 8-Fr Fustar Steerable sheath (Lifetech Scientific Inc., Shenzhen, China) (Fig. 1A). Heparin (50 IU/kg) was administered intravenously and further doses were given to achieve an activated clotting time of >300 s.
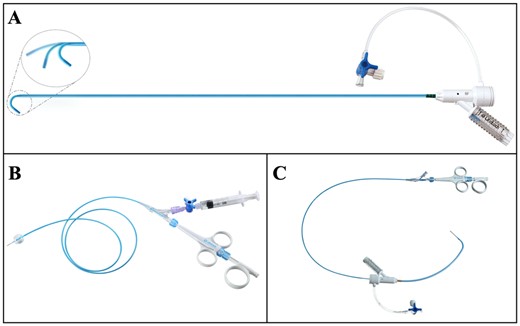
(A) Futar Steerable sheath (Lifetech Scientific Inc., Shenzhen, China) with an adjustable tip. (B) FuThrough needle puncture system (Lifetech Scientific Inc., Shenzhen, China). (C) Illustration of the combination of the 2 devices.
Secondary tear creation model procedure
A 0.035′ inch guidewire (Terumo, Tokyo, Japan) and a 5-Fr pigtail catheter were advanced along the TL into the aortic arch to allow angiography of the aorta and its branches. Subsequently, the Fustar sheath was deployed through the PET to the middle of the FL via the right CCA (Fig. 2A and B). According to the design of the experiment, the Fustar sheath was adjusted to be as perpendicular as possible to the flap at the location of the STC. An adjustable puncture needle system (FuThrough, Lifetech, Shenzhen, China) (Fig. 1B and C) was deployed to the tip of the Fustar sheath. The penetration depth was preselected on the device. A secondary tear was created after pushing the trigger of the puncture needle. Following this, a V-18 wire (Boston Scientific, Marlborough, MA, USA) was advanced into the TL through the central lumen of the puncture needle. Then, the fenestration was sequentially dilated using balloon catheter of 7-mm diameter (Fig. 2C and D). Finally, angiography was performed with the pigtail catheter placed in the TL just above the newly created tear (Supplementary Material, Fig. S1).
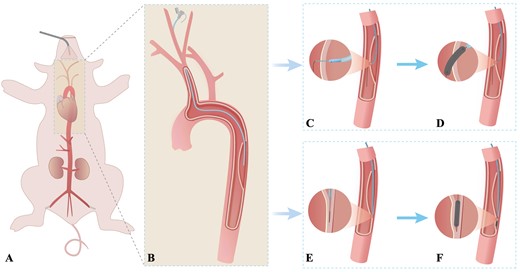
Schematic diagram of the endovascular reintervention-induced porcine type B aortic dissection model. (A and B) The right common carotid artery is exposed through a median neck incision under sterile conditions and cannulated with an 8-Fr Fustar Steerable sheath (Lifetech Scientific Inc., Shenzhen, China), which is deployed through the primary entry tear to the middle of the false lumen. (C and D) Procedure conducted in the secondary tear creation model. The fenestration in the flap is created by combining the adjustable puncture needle system and the Fustar Steerable sheath. Then, the secondary tear is dilated using different balloon diameters as needed. (E and F) Procedure conducted in the false lumen extension model. The tip of the support catheter and guide wire are carefully drilled into the subintima of the aortic wall using the guidewire in the loop formation. The space under the intima was sequentially enlarged using different balloons as needed.
Technical success was defined as the completion of piercing of the dissection flap, guidewire passage, balloon dilation of fenestration, blood flow communication between the TL and FL around the created tear on angiography and 24-h survival of the animal.
False lumen extension model procedure
The Fustar sheath was deployed through the PET to the end of the FL via the right CCA. A V-18 wire and support catheter were advanced to the end of the dissecting flap via the Fustar sheath. Then, the Fustar sheath was adjusted towards the middle layer of the aortic wall. The support catheter and guide wire were carefully drilled into the subintima and the dissection tear level. Angiography was quickly performed through the support catheter to determine if it was located in the dissecting layer and not the intraluminal or extraluminal formation. Next, the V-18 wire was advanced with the support catheter into the created dissected space to the desired location using the guidewire in the loop formation. The area under the intima was sequentially enlarged using balloons of increasing diameters (3, 5 and 7 mm) step by step (Fig. 2E and F). Following balloon dilatation, angiography was performed to determine the acute dissection propagation and FL extension (Supplementary Material, Fig. S2).
Technical success was defined when angiography confirmed that the acute expanded FL reached or exceeded the experimentally expected position, the maximum diameter of the created FL > 10.0 mm and 24-h survival of the animal.
All dissection creation manoeuvres were performed via the femoral arteries and CCA throughout the procedure. The carotid puncture was sutured with 6–0 prolene suture thread. Manual pressure was maintained on the femoral access site for 30 min after sheath retrieval, and puncture sites were closed with medical glue. After a period of recovery, the animals were returned to their cages under veterinary care with continuous access to food and water. All animals received acetylsalicylic acid at a dosage of 100 mg daily post-procedures.
Imaging modalities and tissue postprocessing
CTA and/or intravascular ultrasound imaging follow-up was performed at 30 and 90 days. The animals were maintained on a regular diet until follow-up evaluation, and 100 mg acetylsalicylic acid was administered daily. Two animals in each group were randomly euthanized at 3 months after the procedure, and each animal’s aorta and main branches were carefully removed with their adipose tissue and fixed in 10% phosphate-buffered formaldehyde. For histological evaluation, cross-sectional slices along the specimen were obtained and embedded in paraffin and stained with haematoxylin and eosin and Masson and Elastica van Gieson stain. After preparation, these sections were examined microscopically by an experienced pathologist. Finally, the results of the histological examination were compared with those of the healthy thoracic aortas of the animal models.
Statistics
Animal features and peri-operative progress was reported for analysis. Data were assessed for normality and expressed as n (%) for categorical variables and means ± standard deviations for continuous variables. Continuous variables were compared using Student's t-test or Mann–Whitney U test, and categorical variables were compared using the Fisher’s exact test. Procedural details were compared between the 2 models using the Wilcoxon signed-rank test (nonparametric test for paired data). The study data were analysed using SPSS version 20.0 (IBM, Armonk, NY, USA). A P-value of <0.05 was considered statistically significant.
RESULTS
Surgically created type B aortic dissection model
The SC-TBAD model was successfully induced and confirmed by angiography in all 12 experimental pigs. No complications occurred during the SC-TBAD procedure in any animal. All pigs underwent a CTA scan within 30 days after the procedures. During follow-up, the mean dissected length was 136.3 ± 76.9 mm, while the mean maximum diameter of the created dissection was 21.23 ± 5.02 mm. The mean interval time between the SC-TBAD and endovascular reintervention-induced TBAD (ERI-TBAD) procedures was 23.7 ± 5.8 days. Re-entry tears at the distal of developed dissection were found in all SC-TBAD models at the 30 days’ follow-up, while no other significant re-entry tears were observed in the middle of the dissecting flaps.
Endovascular reintervention-induced type B aortic dissection model
The ERI-TBAD model was successfully induced and confirmed by angiography in 11 animals, with a technical success rate of the model of 91.7% (11/12). One model in the FLE model group failed because the guidewire did not successfully drill into the subintima despite several attempts with different wires and catheters. The mean time for establishing the ERI-TBAD models was 34.2 ± 10.41 min, with 30.32 ± 8.72 min in the STC group and 38.08 ± 11.23 min in the FLE group; the difference was not significant. In the STC group, the intraoperative mean diameter of the endovascular created tear was 7.23 ± 1.02 mm, and a slight increase was observed at the 1-month follow-up (7.65 ± 0.99 mm, P = 0.0026). However, the new secondary tear was stable during the follow-up at 3 months (Fig. 3A). In the FLE group, the intraoperative new propagation length was 235.80 ± 84.94 mm. Notably, the mean length of the FL at the 1-month follow-up 272.50 ± 90.39 mm was significantly greater than that measured intraoperatively based on digital subtraction angiography images (P = 0.0362). The new FLs did not have significant propagations at 3 months’ follow-up (Fig. 3B). Detailed results between the 2 groups are shown in Table 1 and Supplementary Material, Table.
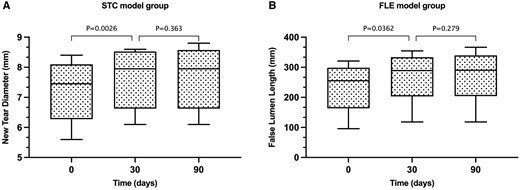
(A) In the secondary tear creation model group, the diameter of new created tear had a slight increase at 30 days after the procedure (P = 0.0026). However, the new tear was stable without significant change at 3 months’ follow-up. (B) In the false lumen extension model group, the average propagation length of the induced new false lumen was greater at the 30 days’ follow-up than that measured intraoperatively. During follow-up, the average propagation length did not change significantly through time.
Characteristics of the 12 animals in the secondary tear creation model and false lumen extension model groups
. | STC model (n = 6) . | FLE model (n = 6) . | P-Value . |
---|---|---|---|
Weight (kg), mean ± SD | 68.37 ± 4.09 | 66.78 ± 10.16 | 0.73 |
Primary SC-TBAD, mean ± SD | |||
Aortic dissection length (mm) | 156.20 ± 71.21 | 116.40 ± 83.64 | 0.39 |
Interval time (days) | 21.67 ± 7.17 | 25.83 ± 3.43 | 0.23 |
Maximum diameter of dissection (mm) | 22.12 ± 6.37 | 20.33 ± 3.60 | 0.56 |
Technical success rate, % (n) | 100% (6/6) | 83.33% (5/6) | 0.99 |
Procedural time (min), mean ± SD | 30.32 ± 8.72 | 38.08 ± 11.23 | 0.21 |
Contrast dosage (ml), mean ± SD | 76.33 ± 22.90 | 60.83 ± 17.86 | 0.59 |
. | STC model (n = 6) . | FLE model (n = 6) . | P-Value . |
---|---|---|---|
Weight (kg), mean ± SD | 68.37 ± 4.09 | 66.78 ± 10.16 | 0.73 |
Primary SC-TBAD, mean ± SD | |||
Aortic dissection length (mm) | 156.20 ± 71.21 | 116.40 ± 83.64 | 0.39 |
Interval time (days) | 21.67 ± 7.17 | 25.83 ± 3.43 | 0.23 |
Maximum diameter of dissection (mm) | 22.12 ± 6.37 | 20.33 ± 3.60 | 0.56 |
Technical success rate, % (n) | 100% (6/6) | 83.33% (5/6) | 0.99 |
Procedural time (min), mean ± SD | 30.32 ± 8.72 | 38.08 ± 11.23 | 0.21 |
Contrast dosage (ml), mean ± SD | 76.33 ± 22.90 | 60.83 ± 17.86 | 0.59 |
FLE: false lumen extension; SC: surgically created; SD: standard deviation; STC: secondary tear creation; TBAD: type B aortic dissection.
Characteristics of the 12 animals in the secondary tear creation model and false lumen extension model groups
. | STC model (n = 6) . | FLE model (n = 6) . | P-Value . |
---|---|---|---|
Weight (kg), mean ± SD | 68.37 ± 4.09 | 66.78 ± 10.16 | 0.73 |
Primary SC-TBAD, mean ± SD | |||
Aortic dissection length (mm) | 156.20 ± 71.21 | 116.40 ± 83.64 | 0.39 |
Interval time (days) | 21.67 ± 7.17 | 25.83 ± 3.43 | 0.23 |
Maximum diameter of dissection (mm) | 22.12 ± 6.37 | 20.33 ± 3.60 | 0.56 |
Technical success rate, % (n) | 100% (6/6) | 83.33% (5/6) | 0.99 |
Procedural time (min), mean ± SD | 30.32 ± 8.72 | 38.08 ± 11.23 | 0.21 |
Contrast dosage (ml), mean ± SD | 76.33 ± 22.90 | 60.83 ± 17.86 | 0.59 |
. | STC model (n = 6) . | FLE model (n = 6) . | P-Value . |
---|---|---|---|
Weight (kg), mean ± SD | 68.37 ± 4.09 | 66.78 ± 10.16 | 0.73 |
Primary SC-TBAD, mean ± SD | |||
Aortic dissection length (mm) | 156.20 ± 71.21 | 116.40 ± 83.64 | 0.39 |
Interval time (days) | 21.67 ± 7.17 | 25.83 ± 3.43 | 0.23 |
Maximum diameter of dissection (mm) | 22.12 ± 6.37 | 20.33 ± 3.60 | 0.56 |
Technical success rate, % (n) | 100% (6/6) | 83.33% (5/6) | 0.99 |
Procedural time (min), mean ± SD | 30.32 ± 8.72 | 38.08 ± 11.23 | 0.21 |
Contrast dosage (ml), mean ± SD | 76.33 ± 22.90 | 60.83 ± 17.86 | 0.59 |
FLE: false lumen extension; SC: surgically created; SD: standard deviation; STC: secondary tear creation; TBAD: type B aortic dissection.
Histological findings
According to our macroscopic examination, the dissection plane was located in the media layer of the aorta. Microscopic examination of the normal thoracic aorta demonstrated that the vasa vasorum was mainly distributed in the adventitia and the outer third of the media (Fig. 4). Histological findings generally aligned with the macroscopic examination (Fig. 5A). Furthermore, microscopic examination revealed that the elastic fibres in the media layer of the aortic wall were disrupted. The elastic fibres were visibly more stretched on the adventitial side of the FL than on the intimal side (Fig. 5B and C). Histological analysis was also performed on the aortic dissection at the branch of an intercostal artery (Fig. 5D and E). Elastica van Gieson staining demonstrated that the elastic fibres on the intimal side gradually gathered and terminated at the ostium of the branch. In contrast, the elastic fibres on the adventitial side extended into the aortic branch.
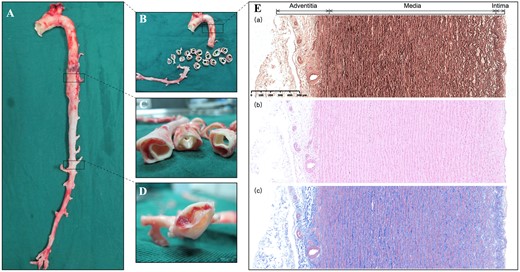
(A and B) On macroscopic examination, the false lumen is extended into the orifice of the superior mesenteric artery. (C) The created dissection is located in the media layer of the aorta. (D) A re-entry tear found distal to the false lumen. (E) Microscopic examination of the normal thoracic aorta, including Elastica van Gieson (a), haematoxylin and eosin (b) and Masson staining (c), demonstrating that the aortic wall comprises 3 layers: the intima, the media and the adventitia. The vasa vasorum in the aortic wall is distributed in the adventitia and the outer third of the media.
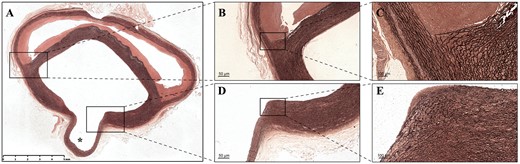
(A) Overview of the Elastica van Gieson staining of the aortic dissection at the branch of the intercostal artery (asterisk). (B) Microscopic examination of elastin staining showing the destruction of the elastic lamellar architecture in the media layer of the aortic wall. (C) Elastic fibres are much more stretched on the adventitial side of the false lumen than on the intimal side. (D and E) The elastic fibres on the intimal side gradually gather and terminate at the orifice of the aortic branch, while the elastic fibres on the adventitial side extend into the aortic branch.
DISCUSSION
Our study describes an endovascular method for creating different anatomical TBAD models based on a previous porcine model with a single PET [10]. The protocol was reproducible in 11 of 12 animals leading to the controlled creation of TBAD models with different characteristics whilst promoting survivability by limiting surgical trauma. Reproducibility is vital, but minimizing invasiveness is essential for adherence to the ARRIVE guidelines for the protection of the subjects [11]. No deaths were reported within 30 days after the primary procedures in the SC-TBAD model, and all pigs were able to undergo CTA scans and reinterventions as planned. Large pigs (average weight of 67.58 kg) were used because their vascular anatomy and vessel size is comparable to those of humans. These results indicate that pigs are candidate animals for in vivo experimental TBAD models, and appropriate for the study of novel human diagnostic and therapeutic strategies.
Previous studies have described several morphological features that are associated with late aneurysmal dilatation and mortality in subjects with TBAD [12]. Other anatomical features may also be relevant to the prognosis of TBAD but have not yet been discovered. Xu et al. [13] proposed a CT-based functional indicator to balance the position of luminal pressure differences, quantifying unique haemodynamic environments to help predict remodelling after TEVAR. However, the flow analyses in their study were based on computational fluid dynamics, and the accuracy of this methodology requires further improvement for optimized estimation of functional indicators [14]. A specific TBAD animal model would allow such study of complex haemodynamics in a real-world setting and potentially define complex risk factors such as these.
Various techniques have been used to create a proximal PET, including elastase infusion, saline infusion, the application of an endarterectomy spatula and endovascular techniques using a modified sheath [7–9, 15, 16]. Okuno et al. also reported a minimally invasive endovascular method for porcine TBAD model creation that does not require aortic cross-clamping. However, in that study, a patent, chronically dissected aortic aneurysm was successfully created in only 5 out of 14 cases. A further drawback was that the dissection propagated unpredictably once the PET was created and a steep learning curve was observed when attempting to master the endovascular technique that using a modified 10F outer catheter with a 14G stiffening metallic cannula inside to create an initial dissection [16]. We developed a new and effective technique with endovascular procedures based on previous SC-TBAD models. Devices such as the adjustable sheath (Fustar sheath) and the adjustable puncture needle system (FuThrough catheter), can assist in the creation of different morphologies, such as a new dissection space or secondary tear. These specific devices are used routinely for in situ fenestration to preserve aortic arch branches during TEVAR [17, 18]. And now they have been demonstrated to be safe and effective in assisting in the creation of endovascular TBAD models. Meanwhile, the size of balloon catheter used to create new tears or new FL were depending on the aim of the study. In our study, the newly created tear and newly induced FL only had slight growth at 30 days after the endovascular procedures. Notably, the created specific features of the TBAD models were kept stable during the following 2 months, indicating the endovascular-induced subacute or chronic TBAD models were partly controllable and reproducible.
Razavi et al. [15] reported a porcine model created successfully by a percutaneous procedure using a needle, but this was limited by the difficulty in reaching a predefined position and preventing the needle tip from inadvertently penetrating the outer aortic wall. In contrast, in our model allowed the controlled creation of a secondary tear or new dissection plane aided by the aforementioned adjustable devices.
Post-TEVAR sequelae requiring reintervention pose an important challenge, and many new devices and treatment strategies are being developed that need to be understood and applied to clinical practice in short order [19–22]. The models in our study will allow the design and testing of novel endovascular devices, such as plugging devices for tears, embolized materials for the FL, angioplasty balloons and stents or stent grafts. As vessel diameters in these model animals are similar to those in humans, these models can assist in the assessment of the effectiveness of new treatment devices in vivo using disease model animals instead of an in vitro simulator [23].
In our study, histological observation of the healthy thoracic aorta showed that the vasa vasorum was mainly distributed in the adventitia and the outer third of the media. According to the arrangement and distribution of elastic fibres at the aortic branch, a deeper initial dissecting flap may contribute to acute dissection propagation [10], branch ischaemia or adverse events [24]. Recently, Bondesson et al. [25] noted that helical TL morphology influenced the prognosis of TBAD after TEVAR. Therefore, anatomical morphology, representing structural features of the vessels and indicating the severity of disease, is worthy of further attention and research in the future.
Limitations
First, our conclusions are based on a limited sample size, so validation studies involving large animals in other labs are required to demonstrate reproducibility. Second, the FL propagated acute after endovascular intervention in the FLE model, leading to different anatomical features during follow-up. While our model can contribute to the development of new therapies, it cannot examine the aetiology of aortic dissection because it the iatrogenic nature of the initial intimal injury. Nevertheless, these porcine models are suitable for future research studying the haemodynamics of acute or chronic TBAD and therefore its role in the pathogenesis of aortic dissection and subsequent degeneration or remodelling. These studies will be facilitated by in vivo-specific TBAD models produced by our methods.
CONCLUSION
In this study, we proposed a minimally invasive endovascular model of TBAD with specific anatomical features that is effective in pigs, safe and reproducible. This in vivo animal model is promising and should contribute to the development of advanced therapies for aortic dissection.
SUPPLEMENTARY MATERIAL
Supplementary material is available at ICVTS online.
ACKNOWLEDGEMENTS
We would like to thank Drs Fu Meng and Guanghui Zhu in the contribution to the anaesthesia management of animals.
Funding
This work was supported by the National Natural Science Foundation of China (82000436), the Science and Technology Commission of Shanghai Municipality (21410710500, 201409004800), the Project of Clinical Research in Health Industry of Shanghai Municipal Health Commission (20214Y0274) and the Clinical Medicine Innovation Fund of Fudan Zhangjiang Institute (KP7202116).
Conflict of interest: none declared.
DATA AVAILABILITY
Databases and code will be made available from the corresponding author upon reasonable request.
Author contributions
Baolei Guo: Conceptualization; Data curation; Formal analysis; Funding acquisition; Investigation; Methodology; Supervision; Writing—original draft; Writing—review & editing. Riaz Akhtar: Conceptualization; Methodology; Writing—review & editing. Haofei Liu: Data curation; Investigation; Writing—review & editing. Cheng Yan: Data curation; Formal analysis; Investigation. Daqiao Guo: Conceptualization; Data curation; Supervision. Benjamin Patterson: Conceptualization; Methodology; Supervision; Writing—review & editing. Weiguo Fu: Conceptualization; Investigation; Supervision; Validation.
Reviewer information
Interdisciplinary CardioVascular and Thoracic Surgery thanks Roman Gottardi and the other anonymous reviewer(s) for their contribution to the peer review process of this article.
REFERENCES
ABBREVIATIONS
- CCA
Common carotid artery
- CTA
Computed tomography angiography
- ERI-TBAD
Endovascular reintervention-induced type B aortic dissection
- FL
False lumen
- FLE
False lumen extension
- PET
Primary entry tear
- SC-TBAD
Surgically created type B aortic dissection
- STC
Secondary tear creation
- TBAD
Type B aortic dissection
- TEVAR
Endovascular thoracic aortic repair
- TL
True lumen