-
PDF
- Split View
-
Views
-
Cite
Cite
Massimo A. Padalino, Andrea Quarti, Emanuela Angeli, Anna C. Frigo, Vladimiro L. Vida, Marco Pozzi, Gaetano Gargiulo, Giovanni Stellin, Early and mid-term clinical experience with extracellular matrix scaffold for congenital cardiac and vascular reconstructive surgery: a multicentric Italian study, Interactive CardioVascular and Thoracic Surgery, Volume 21, Issue 1, July 2015, Pages 40–49, https://doi.org/10.1093/icvts/ivv076
- Share Icon Share
Abstract
The aim of this multicentric study was to outline surgical indications and evaluate mid-term outcomes of porcine extracellular matrix (ECM) in surgery for congenital heart disease (CHD).
The use of ECM was categorized into four major groups: A, valve repair; B, septal reconstruction; C, arterial plasty; D, other use. Primary endpoints of analysis were reintervention (either surgical or interventional) when related to ECM, and functional ECM failure. Secondary endpoints were evidence of calcification and of persistent inflammation at follow-up.
One hundred and three patients (M/F = 61/42, median age 19.7 months, 1 day-62 years) underwent surgical repair for CHD. Among ECM use categories, 38 patients were in Group A, 16 in Group B, 71 in Group C and 7 in Group D. There were neither complications nor deaths related to ECM. At a median follow-up of 23.3 months (0.3–55.2), 19 patients underwent reoperation (ECM-related in 6); 11 patients underwent interventional cardiology procedures (ECM-related in 8). Reinterventions were significantly more frequent on the aortic valve (surgical, P = 0.0056) and pulmonary arteries (interventional, P = 0.0159). In addition, interventional procedures on pulmonary arteries were significantly more frequent in infants <12 months (P = 0.0474). No calcifications were detected.
Surgical use of ECM in CHD repair is characterized by a suboptimal functional late performance on reconstruction of valve leaflet or pulmonary artery wall. Longer follow-up and larger clinical experience may support these preliminary results on mid-term outcomes, so as to assess the optimal indication for an ECM graft.
INTRODUCTION
Most of congenital heart diseases (CHDs) are currently amenable to surgical repair in early infancy or childhood, with good early and late outcomes. However, most CHD-related surgical procedures require the use of prosthetic material for reconstruction of intracardiac and extracardiac structures. Several different biological and prosthetic materials are commonly used in surgery for CHDs, such as autologous pericardium (with or without glutaraldehyde rinsing) [1], preserved homograft, bovine pericardium [2] and polytetrafluoroethylene [3, 4]. None of the above-mentioned represents the ideal prosthetic tissue, which should be pliable and easy to handle, resistant to tearing, calcification or shrinkage, and most importantly should have growth potential and should not induce remodelling of scar tissue.
Tissue engineering represents a new approach to tissue and organ regeneration and replacement. Specifically, cardiac and vascular tissue engineering is an emerging field that may hold a great promise for advancement in the treatment of CHDs [5]. The extracellular matrix (ECM) scaffold is a material that is derived from different tissues. These ECM materials are supposed to provide an interim bioscaffold that enables the patient's own cells to repopulate and repair tissues, and have already been shown to facilitate the constructive remodelling of many different tissues in both preclinical animal studies [6, 7] and in human clinical applications [8, 9].
Based on this knowledge, the use of a biological scaffold material derived from porcine small intestine submucosa (SIS)–ECM may reflect a new type of approach [5–7]. We describe our clinical experience with surgical application of a SIS–ECM scaffold to repair congenital cardiac and vascular anomalies.
MATERIALS AND METHODS
Study design
This is a multicentre, prospective, non-randomized clinical study, which was designed to evaluate the technical (such as handling characteristics and haemostatic status) and clinical (such as calcification potential safety, durability and late functional results) characteristics of the SIS–ECM scaffold (CorMatrix Cardiovascular, Atlanta, GA, USA), which is available for clinical use in many applications, and is marketed with FDA and CE approval for extracardiac and cardiac use in humans for many years now, as reported elsewhere [7–9]. Our study includes patients who underwent surgery for CHD, in whom the SIS–ECM was used as a scaffold to perform surgical repair. A review of medical records was approved by each hospital local ethics committee on clinical investigation. Informed consent was obtained prior to surgery from all patients or their parents or legal guardian. None of the authors has any conflict of interest to declare and the study was spontaneous.
Patients were evaluated preoperatively with routine controls, including baseline values of haematology; SIS–ECM was used in selected patients with CHDs in whom regenerative potential was considered highly desirable so as to decrease, or at least delay, the probability for reoperation. The use of the SIS–ECM scaffold was categorized into four major groups: A, valve repair, when SIS–ECM was used as a material to reconstruct valve leaflets, according to different techniques, including aortic leaflet extension [10] and leaflet augmentation (Fig. 1A), or pulmonary valve (PV) reconstruction (Fig. 1B); B, septal reconstruction, whenever SIS–ECM was used to septate atria or ventricles, in cases of partial or total anomalous pulmonary venous connection patch repair, or complex intraventricular blood flow rerouting in double outlet right ventricle (DORV) repair with uncommitted ventricular septal defects (SDs); C, arterial plasty, when SIS–ECM was used as patch augmentation for any arterial (either systemic or pulmonary) vessel (Fig. 2); D, other use, such as pericardium reconstruction, or venous vessel plasty. In addition, each major group was subdivided in subcategories according to the specific anatomical site which underwent surgery with the SIS–ECM scaffold, such as on aortic valve (AoV), PV, mitral valve (MV), tricuspid valve (TV), interatrial or intraventricular septa, aorta (either ascending aorta or arch), main pulmonary artery (MPA), branch pulmonary arteries (PAs), pericardium or other less common sites (Supplementary Material, Appendix 1).
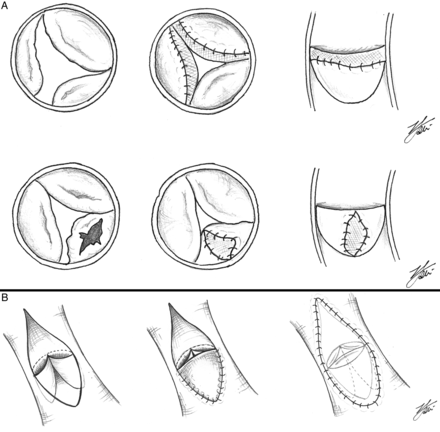
(A) Diagram showing the aortic valve repair technique: in the upper diagrams, the leaflet extension technique is described, in which usually the thickened and dysplastic leaflet edge is excised, or the leaflet is shaved, and the nodular lesions are excised. The length of the free edge of the leaflet is usually measured and the height of the leaflet extension is determined by measuring the depth of the native left coronary leaflet. Based on these two measurements, rectangular-shaped SIS–ECM extensions are sewn to the free edge of the cusps by 6.0 or 7.0 polypropylene sutures; the new commissures are then created by sewing each of the two leaflets of the aortic wall together upwards towards the new sinotubular junction. In the lower diagrams, leaflet augmentation is described, in which the leaflet surface (which is deficient congenitally or presents a perforation) is augmented by the inclusion of a SIS–ECM patch inside the native tissue, which completely surrounds the SIS–ECM patch. (B) Diagram showing pulmonary valve reconstruction in a transannular repair of tetralogy of Fallot by means of the SIS–ECM patch applied as pulmonary valve anterior leaflet; then the right ventricular outflow tract and the main pulmonary artery are reconstructed by means of another larger SIS–ECM patch. SIS: small intestine submucosa; ECM: extracellular matrix.
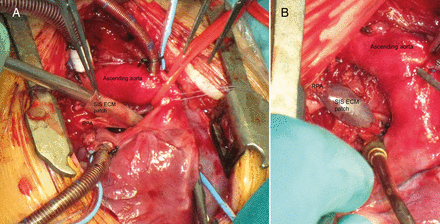
In an 8-month old patients with a single ventricle and hypoplastic right pulmonary artery (RPA), a SIS–ECM patch plasty is performed on the RPA: in (A), the RPA is incised longitudinally and the rectangular-shaped SIS–ECM patch is calibrated on a Hegar dilator whose diameter is adequate to the RPA of the patient; the SIS–ECM patch is sutured with 7.0 prolene on the RPA and the final result of SIS–ECM patch plasty is shown in (B). SIS: small intestine submucosa; ECM: extracellular matrix.
Intraoperative surgical assessment included handling characteristics, haemostasis and overall seating of the implanted patch. The surgical techniques varied according to the type of CHD and either repair or palliative procedures were included in this series. Each procedure-specific risk was evaluated with the Aristotle Basic Score, so as to adjust the specific surgical risk bias with SIS–ECM material utilization.
Postoperative and follow-up evaluation included standard clinical and imaging assessment in each centre, such as echocardiography (at about 6, 12, 24 and 36 months), cardiac catheterization (diagnostic or interventional) and magnetic resonance imaging (MRI) or CT scan, whenever indicated, so as to determine surgical outcomes and SIS–ECM-related events, calcification or anatomical distortion.
Primary endpoints of analysis were as follows: (i) reoperation, when related to SIS–ECM; (ii) interventional cardiology procedure, when related to SIS–ECM; (iii) functional ECM failure, defined specifically for all different groups, as: valve failure in Group A (in the presence of a significant stenosis or regurgitation detected on two–dimensional (2D) echocardiography; specifically, in the AoV subgroup, a peak gradient >40 mmHg defined a significant stenosis; when a regurgitant jet was 70% or more with Doppler echocardiography, it was defined as significant valve regurgitation); septal reconstruction failure in Group B (when bidimensional echocardiography was detecting a significant residual shunt, or an intracavitary SIS–ECM tissue bulging causing a haemodynamically significant inflow stenosis or distortion of surrounding structures); arterial plasty failure in Group C (if vessel stenosis or aneurysm was detected on bidimensional echocardiography and MRI); any adverse event in Group D. Association between the ECM scaffold and adverse event was discussed and evaluated together with the referring surgeon and cardiologist.
Secondary additional endpoints for each surgical application group were analysed:
evidence of calcification, detected at reoperation or at roentgenographic imaging;
evidence of persistent inflammation, evaluated as persistence of hyperpyrexia, unexplained increase of inflammatory response mediators or C-reactive protein (if available) or procalcitonin, with no evidence of infection, and no response to antibiotic therapy. In addition, further analysis of outcomes in Category A was performed to evaluate whether leaflet augmentation technique (in which the scaffold was ‘included’ in native host tissue) had a better late outcome when compared with the leaflet extension technique (when the scaffold was sutured to the edge of the leaflet; Fig. 1A).
Statistical analysis
Statistical analysis investigated the possible effects of SIS–ECM scaffold surgical application on the onset of adverse events, previously outlined as primary or secondary endpoints at follow-up. We evaluated patients' characteristics (anatomical diagnosis of single ventricle; age <1 year at ECM surgery, weight <10 kg at ECM surgery, surgical ECM category, ECM use on different anatomical sites—PV, AoV, PA main and branches and procedure-specific Aristotle Basic Score) as possible risk factors for adverse ECM-related events. Survival curves (freedom from reoperation, interventional cardiology procedure, functional failure and their combination) were estimated with the Kaplan–Meier method. Analysis was performed on ECM related events, which were associated to the prevalent ECM surgical category in each patient when more than one use was done; the prevalent surgical category was chosen by the referring surgeon according to the clinical impact of the surgical procedure on the clinical result). The association of patients’ and technique characteristics to the major endpoints (surgical reoperation, interventional cardiology procedure or functional failure) and their combination were investigated with univariate Cox regression analysis with Firth's penalized maximum likelihood estimation when at least one estimate was diverging to infinity. The variables with P < 0.2 at the univariate analysis were introduced in a multivariate Cox regression model and a backward selection method was applied for identification of the independent predictors of functional failure and of the combined endpoint at the 5% significance level. No multivariate analysis was done for the other two endpoints owing to the small number of events. The results are presented as number and percentages of patients in each category of predictor considered and as hazard ratio, 95% confidence interval and P-values. Analyses were conducted with SAS 9.2 (SAS Institute, Inc., Cary, NC, USA) for Windows.
RESULTS
Early outcomes
Between August 2009 and January 2014, 103 patients with CHD (M/F = 61/42, median age at ECM surgery: 19.7 months, range 1 day-62 years, median weight: 10 kg, range 1.8–132) were enrolled in three different centres. All patients underwent a cardiac surgical procedure in which the SIS–ECM scaffold was employed for the treatment of CHDs. Overall, there were 132 ECM surgical procedures performed on 103 patients (1.28 per patient). There were several types of complex CHDs, including single-ventricle physiology CHD; the median Aristotle score was 8 (Table 1). In 43 patients, the SIS–ECM scaffold was employed in a reoperation. All demographic data are summarized in detail in Table 1. According to surgical classification of SIS–ECM use, 38 patients (28.8%) underwent valve repair (Category A), 16 (12.1%) underwent surgical septation (Category B), 71 (53.8%) had arterial wall plasty (Category C) and 7 (5.3%) had some other different use of SIS–ECM (Category D).
Variable . | Patients . | % . |
---|---|---|
Total patients | 103 | 100 |
Age at ECM surgery (months; median, range) | 19.7 (0.03–746.03) | |
Weight at ECM surgery (kg; median, range) | 10 (1.8–132) | |
Redo surgery | 44/103 | 42.7 |
Male | 61/103 | 59.2 |
Total ECM surgical procedures | 132 | 100 |
ECM surgical category A | 38 | 28.8 |
ECM surgical category B | 16 | 12.1 |
ECM surgical category C | 71 | 53.8 |
ECM surgical category D | 7 | 5.3 |
Aristototles’ score (median, range) | 8 (1–15) | |
CHD diagnosis | ||
Tetralogy of Fallot | 22 | 21.35 |
Single ventricle | 20 (HLHS in 3) | 19.4 |
Aortic valve regurgitation/stenosis | 14 (2 in HLHC) | 13.6 |
Supravalvar aortic stenosis | 9 | 8.7 |
Transposition of the great arteries | 8 | 7.7 |
Double-outlet right ventricle | 5 (2 in Taussig-Bing) | 4.8 |
Partial anomalous pulmonary venous connection | 5 | 4.8 |
Truncus arteriosus | 4 | 3.8 |
Ebstein anomaly | 3 | 2.9 |
Tetralogy of Fallot, with pulmonary atresia and MAPCAs | 3 | 2.9 |
Mitral regurgitation | 2 | 2.9 |
Atrioventricular septal defect | 3 | 1.9 |
Pulmonary stenosis | 1 | 0.9 |
Total anomalous pulmonary venous connection | 1 | 0.9 |
Ventricular septal defect | 1 | 0.9 |
Vascular ring | 1 | 0.9 |
Aorto-pulmonary window | 1 | 0.9 |
Variable . | Patients . | % . |
---|---|---|
Total patients | 103 | 100 |
Age at ECM surgery (months; median, range) | 19.7 (0.03–746.03) | |
Weight at ECM surgery (kg; median, range) | 10 (1.8–132) | |
Redo surgery | 44/103 | 42.7 |
Male | 61/103 | 59.2 |
Total ECM surgical procedures | 132 | 100 |
ECM surgical category A | 38 | 28.8 |
ECM surgical category B | 16 | 12.1 |
ECM surgical category C | 71 | 53.8 |
ECM surgical category D | 7 | 5.3 |
Aristototles’ score (median, range) | 8 (1–15) | |
CHD diagnosis | ||
Tetralogy of Fallot | 22 | 21.35 |
Single ventricle | 20 (HLHS in 3) | 19.4 |
Aortic valve regurgitation/stenosis | 14 (2 in HLHC) | 13.6 |
Supravalvar aortic stenosis | 9 | 8.7 |
Transposition of the great arteries | 8 | 7.7 |
Double-outlet right ventricle | 5 (2 in Taussig-Bing) | 4.8 |
Partial anomalous pulmonary venous connection | 5 | 4.8 |
Truncus arteriosus | 4 | 3.8 |
Ebstein anomaly | 3 | 2.9 |
Tetralogy of Fallot, with pulmonary atresia and MAPCAs | 3 | 2.9 |
Mitral regurgitation | 2 | 2.9 |
Atrioventricular septal defect | 3 | 1.9 |
Pulmonary stenosis | 1 | 0.9 |
Total anomalous pulmonary venous connection | 1 | 0.9 |
Ventricular septal defect | 1 | 0.9 |
Vascular ring | 1 | 0.9 |
Aorto-pulmonary window | 1 | 0.9 |
CHD: congenital heart disease; ECM: extracellular matrix; HLHC: hypoplastic left heart complex; HLHS: hypoplastic left heart syndrome; MAPCAs: major aorto-pulmonary collateral arteries.
Variable . | Patients . | % . |
---|---|---|
Total patients | 103 | 100 |
Age at ECM surgery (months; median, range) | 19.7 (0.03–746.03) | |
Weight at ECM surgery (kg; median, range) | 10 (1.8–132) | |
Redo surgery | 44/103 | 42.7 |
Male | 61/103 | 59.2 |
Total ECM surgical procedures | 132 | 100 |
ECM surgical category A | 38 | 28.8 |
ECM surgical category B | 16 | 12.1 |
ECM surgical category C | 71 | 53.8 |
ECM surgical category D | 7 | 5.3 |
Aristototles’ score (median, range) | 8 (1–15) | |
CHD diagnosis | ||
Tetralogy of Fallot | 22 | 21.35 |
Single ventricle | 20 (HLHS in 3) | 19.4 |
Aortic valve regurgitation/stenosis | 14 (2 in HLHC) | 13.6 |
Supravalvar aortic stenosis | 9 | 8.7 |
Transposition of the great arteries | 8 | 7.7 |
Double-outlet right ventricle | 5 (2 in Taussig-Bing) | 4.8 |
Partial anomalous pulmonary venous connection | 5 | 4.8 |
Truncus arteriosus | 4 | 3.8 |
Ebstein anomaly | 3 | 2.9 |
Tetralogy of Fallot, with pulmonary atresia and MAPCAs | 3 | 2.9 |
Mitral regurgitation | 2 | 2.9 |
Atrioventricular septal defect | 3 | 1.9 |
Pulmonary stenosis | 1 | 0.9 |
Total anomalous pulmonary venous connection | 1 | 0.9 |
Ventricular septal defect | 1 | 0.9 |
Vascular ring | 1 | 0.9 |
Aorto-pulmonary window | 1 | 0.9 |
Variable . | Patients . | % . |
---|---|---|
Total patients | 103 | 100 |
Age at ECM surgery (months; median, range) | 19.7 (0.03–746.03) | |
Weight at ECM surgery (kg; median, range) | 10 (1.8–132) | |
Redo surgery | 44/103 | 42.7 |
Male | 61/103 | 59.2 |
Total ECM surgical procedures | 132 | 100 |
ECM surgical category A | 38 | 28.8 |
ECM surgical category B | 16 | 12.1 |
ECM surgical category C | 71 | 53.8 |
ECM surgical category D | 7 | 5.3 |
Aristototles’ score (median, range) | 8 (1–15) | |
CHD diagnosis | ||
Tetralogy of Fallot | 22 | 21.35 |
Single ventricle | 20 (HLHS in 3) | 19.4 |
Aortic valve regurgitation/stenosis | 14 (2 in HLHC) | 13.6 |
Supravalvar aortic stenosis | 9 | 8.7 |
Transposition of the great arteries | 8 | 7.7 |
Double-outlet right ventricle | 5 (2 in Taussig-Bing) | 4.8 |
Partial anomalous pulmonary venous connection | 5 | 4.8 |
Truncus arteriosus | 4 | 3.8 |
Ebstein anomaly | 3 | 2.9 |
Tetralogy of Fallot, with pulmonary atresia and MAPCAs | 3 | 2.9 |
Mitral regurgitation | 2 | 2.9 |
Atrioventricular septal defect | 3 | 1.9 |
Pulmonary stenosis | 1 | 0.9 |
Total anomalous pulmonary venous connection | 1 | 0.9 |
Ventricular septal defect | 1 | 0.9 |
Vascular ring | 1 | 0.9 |
Aorto-pulmonary window | 1 | 0.9 |
CHD: congenital heart disease; ECM: extracellular matrix; HLHC: hypoplastic left heart complex; HLHS: hypoplastic left heart syndrome; MAPCAs: major aorto-pulmonary collateral arteries.
According to the anatomical site of repair, the most frequent surgical procedures were MPA and PA arterial wall patch plasty (29 and 21, respectively, accounting for ∼35% of all procedures), followed by aortic wall patch plasty (21 cases) and PV plasty or reconstruction (18 cases). In particular, PV reconstruction was performed by means of some type of leaflet extension techniques, or as PV monocusp reconstruction (Fig. 1B), as described elsewhere [11]. In addition, in Category A (valve repair), most patients with AoV disease (11) underwent valve repair by means of leaflet extension technique [10]. In Category C, several patients had PA reconstruction (mostly main PA), whereas the ascending aorta was reconstructed with SIS–ECM in 13 patients (including also a Damus–Kaye–Stensel procedure in 3) and aortic arch patch reconstruction was performed in 8 neonates and infants.
All patients underwent cardiac surgical procedures requiring cardiopulmonary bypass. The general impression among various surgeons in the three different centres was favourable: handling characteristic and overall seating of the implanted patch was good, and haemostasis was excellent. However, it was indicated by some surgeons that the four-layer patch could occasionally defoliate when rinsed for longer than 5 min. There were no cases of graft rupture or dehiscence. Major postoperative complications occurred in 26 patients (25.2%), but none of them could be directly related to SIS–ECM scaffold application. There were 4 early deaths (3.8%). One neonate affected by scimitar syndrome, who had undergone intra-atrial baffling of right upper pulmonary veins with a pericardial patch, and plasty of the lower right pulmonary vein with the SIS–ECM scaffold, could not be weaned off cardiopulmonary bypass because of severe hypoxia and required extracorporeal membrane oxygenation (ECMO) support; even though serial echocardiographic and catheterization assessments did not reveal any significant residual lesions, the patient died after 10 days. Another patient (18 months of age) with tetralogy of Fallot, pulmonary atresia and major aorto-pulmonary collateral arteries underwent PA bifurcation enlargement patch plasty with SIS–ECM, in addition to a right modified Blalock-Taussig shunt (MBTS); the patient developed MBTS thrombosis which required two reoperations and ECMO support; despite the absence of significant residual lesions on echocardiographic evaluation, the patient died 2 days after ECMO. Another 17-month old child with Ebstein anomaly underwent a TV plasty with SIS–ECM (leaflet extension) and a cavopulmonary anastomosis; because of persistent hypoxia, 5 days later he required a take-down of the cavopulmonary anastomosis and a systemic-to-pulmonary shunt, with ECMO support, followed by salvage biventricular assist device implant; however, he died a few days later from multiorgan failure. One last neonate (20 days of life) with hypoplastic left heart syndrome (HLHS) underwent a Norwood procedure with reconstruction of the aortic arch with SIS–ECM, but died for low cardiac output syndrome 15 days later. None of these hospital deaths could be directly related to the ECM scaffold or its early failure when autopsy was performed.
Late outcomes
At a median follow-up of 23.33 months (range 0.3–55.23, follow-up completeness 100%), there were 4 late deaths (3.9%), but none of them could be related to the SIS–ECM scaffold or its late failure. Two patients died because of chronic congestive heart failure. A 39-year old woman, with unbalanced atrioventricular (AV) SD, pulmonary stenosis and severe regurgitant AV valve, in situs inversus, previously treated with multiple palliations, who underwent AV valve replacement and SIS–ECM pericardial reconstruction, died 1 year later after refusing orthotopic heart transplant. A second patient, a 3-year old male with intermediate atrioventricular septal defect (AVSD), common atrium and anomalous connection of the right pulmonary veins in the right side of the posterior wall of the common atrium, close to the superior vena cava, in heterotaxy syndrome, underwent complete biventricular repair with the SIS–ECM scaffold for interatrial septation; he developed chronic congestive heart failure (ECM not related) due to residual left AV regurgitation and left ventricle–right atrium shunt, together with a spontaneous AV block requiring a pacemaker; he died 2 years after repair. A third patient, 6 months old, with situs inversus, double-outlet right ventricle and Taussig-Bing type, who had undergone at birth aortic arch reconstruction with SIS–ECM, died 15 days after Damus Kaye Stensel palliation because of untreatable low cardiac output syndrome. At post-mortem examination, the aortic arch was unobstructed. One last patient with HLHS, who underwent a successful Norwood procedure at birth with aortic arch reconstruction with SIS–ECM, died 16 months later in another hospital of vasculitis. The patient was admitted with persistent and recurrent fever; a CT scan preformed at admission revealed a normal morphology of the SIS–ECM scaffold previously implanted.
A reoperation was necessary in 19 patients (18.4%): in 13 of them, it was part of the surgical plan for completing repair or palliation of complex CHD, whereas in 6 it was related to ECM scaffold failure, and it occurred after a median time of 25.2 months (range 2.5–34.1 months) from ECM surgery. Redo surgery consisted in valve replacement for failing AoV plasty in 5 out of 10 patients who had undergone leaflet extension repair with SIS–ECM (mechanical AoV prosthesis in 3 and Ross procedure in 2), and for failing MV plasty in 1 who had undergone an anterior mitral leaflet extension (mechanical prosthesis). At the current follow-up, patients who underwent AoV leaflet repair with leaflet augmentation present with good functional outcome on echocardiographic assessment.
At reoperation, usually there was no finding of calcification, and the patch was visible and looked intact, with fibrotic remodelling, and mild stiffness. There were some inflammatory cells, lymphocytes and some giant cells, but no signs of regeneration were found on the aortic site, as outlined by histological analysis (Fig. 3).
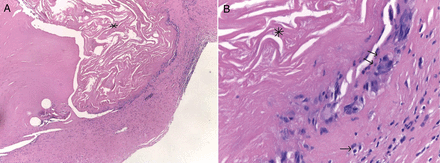
Dystrophic aortic valve leaflet reconstructed with SIS–ECM (Cormatrix) in a 16-year old female, with a diagnosis of congenital aortic stenosis (bicuspid), who had undergone aortic valve balloon dilatation at 5 days of life. This is a histological finding at reoperation (Ross operation) which occurred 24.4 months after aortic valve repair (leaflet extension), at 14 years of age. (A) Haematoxylin and eosin staining shows an intact SIS–ECM scaffold material inside the native leaflet valve tissue (asterisk), with no cell repopulation and no calcifications (×20 of magnification). (B) At greater magnification (×40 of magnification), in the same specimen, with intact SIS–ECM scaffold material inside the native leaflet valve tissue (asterisk), it is possible to visualize a weak inflammatory infiltrate in the valve leaflet–SIS–ECM scaffold transition area, characterized by lymphocytes (double arrows) and giant cells (single arrow). SIS: small intestine submucosa; ECM: extracellular matrix.
An interventional cardiology procedure was done in 11 patients (10.7%), and they were all performed on the PAs (either MPA or PAs). In 8 patients (72.7%), the procedure was required for stenosis occurring at the site of the SIS–ECM scaffold after a median time of 12.6 months (range 2.7–23.0 months) from ECM surgery. Interventional procedures consisted in balloon dilatation in 4 (MPA in 3 and PA in 1) and stenting in 4 (PAs in 3, right ventricular outflow tract and the MPA in 1), with no complications. At the current follow-up time, functional failure (described as above) occurred in 28 patients (27.2%, Table 2), mostly in Categories A and C. In particular, AoV and PV (AoV in 7, 5 of whom were replaced; PV in 8) and PAs (5 in the MPA and 5 in PAs) were mostly affected by failures, which occurred as residual progressive regurgitation and stenosis, respectively.
Variable . | Patients, n (%) . |
---|---|
Total patients | 103 (100) |
Early outcome | |
Major complication during hospitalization (patients) | 26 (25.2) |
Major complication during hospitalization related to Cormatrix (patients) | 0 (0) |
Median ICU stay (days) | 3 (1–90) |
Median IPPV duration (h) | 20 (1–2160) |
Median hospital stay (days) | 15 (6–115) |
Hospital death (patients) | 4 (3.8) |
Hospital death, related to Cormatrix (patients) | 0 (0) |
Late outcome | |
Follow-up length (months; median, range) | 23.33 (0.3–55.23) |
Late death (patients) | 4 (3.8) |
Late death, related to ECM (patients) | 0 (0) |
Reoperations (patients) | 19 (18.4) |
Reoperations, related to ECM (patients) | 6 (5.8) |
Interval between ECM surgery and reoperation (months; median, range) | 25.2 (2.5–34.1) |
(Group A) Valve repair | 6 |
(Group B) Septal reconstruction | 0 |
(Group C) Arterial plasty | 0 |
(Group D) Other | 0 |
Interventional cardiology procedures (patients) | 11 (10.7) |
Interventional cardiology procedures, related to ECM (patients) | 8 (7.7) |
Interval between ECM surgery and interventional cardiology procedure (months; median, range) | 12.6 (2.7–23.0) |
(Group A) Valve repair | 0 |
(Group B) Septal reconstruction | 0 |
(Group C) Arterial plasty | 8 |
(Group D) Other | 0 |
ECM functional failure at follow-up (patients) | 28 (27.2) |
(Group A) Valve repair | 21 |
(Group B) Septal reconstruction | 0 |
(Group C) Arterial plasty | 6 |
(Group D) Other | 1 |
Evidence of calcification (patients) | 1 (0.9) |
Clinical evidence of persistent systemic inflammation (patients) | 2 (1.9) |
Variable . | Patients, n (%) . |
---|---|
Total patients | 103 (100) |
Early outcome | |
Major complication during hospitalization (patients) | 26 (25.2) |
Major complication during hospitalization related to Cormatrix (patients) | 0 (0) |
Median ICU stay (days) | 3 (1–90) |
Median IPPV duration (h) | 20 (1–2160) |
Median hospital stay (days) | 15 (6–115) |
Hospital death (patients) | 4 (3.8) |
Hospital death, related to Cormatrix (patients) | 0 (0) |
Late outcome | |
Follow-up length (months; median, range) | 23.33 (0.3–55.23) |
Late death (patients) | 4 (3.8) |
Late death, related to ECM (patients) | 0 (0) |
Reoperations (patients) | 19 (18.4) |
Reoperations, related to ECM (patients) | 6 (5.8) |
Interval between ECM surgery and reoperation (months; median, range) | 25.2 (2.5–34.1) |
(Group A) Valve repair | 6 |
(Group B) Septal reconstruction | 0 |
(Group C) Arterial plasty | 0 |
(Group D) Other | 0 |
Interventional cardiology procedures (patients) | 11 (10.7) |
Interventional cardiology procedures, related to ECM (patients) | 8 (7.7) |
Interval between ECM surgery and interventional cardiology procedure (months; median, range) | 12.6 (2.7–23.0) |
(Group A) Valve repair | 0 |
(Group B) Septal reconstruction | 0 |
(Group C) Arterial plasty | 8 |
(Group D) Other | 0 |
ECM functional failure at follow-up (patients) | 28 (27.2) |
(Group A) Valve repair | 21 |
(Group B) Septal reconstruction | 0 |
(Group C) Arterial plasty | 6 |
(Group D) Other | 1 |
Evidence of calcification (patients) | 1 (0.9) |
Clinical evidence of persistent systemic inflammation (patients) | 2 (1.9) |
ECM: extracellular matrix; ICU: intensive care unit; IPPV: intermittent positive airway ventilation.
Variable . | Patients, n (%) . |
---|---|
Total patients | 103 (100) |
Early outcome | |
Major complication during hospitalization (patients) | 26 (25.2) |
Major complication during hospitalization related to Cormatrix (patients) | 0 (0) |
Median ICU stay (days) | 3 (1–90) |
Median IPPV duration (h) | 20 (1–2160) |
Median hospital stay (days) | 15 (6–115) |
Hospital death (patients) | 4 (3.8) |
Hospital death, related to Cormatrix (patients) | 0 (0) |
Late outcome | |
Follow-up length (months; median, range) | 23.33 (0.3–55.23) |
Late death (patients) | 4 (3.8) |
Late death, related to ECM (patients) | 0 (0) |
Reoperations (patients) | 19 (18.4) |
Reoperations, related to ECM (patients) | 6 (5.8) |
Interval between ECM surgery and reoperation (months; median, range) | 25.2 (2.5–34.1) |
(Group A) Valve repair | 6 |
(Group B) Septal reconstruction | 0 |
(Group C) Arterial plasty | 0 |
(Group D) Other | 0 |
Interventional cardiology procedures (patients) | 11 (10.7) |
Interventional cardiology procedures, related to ECM (patients) | 8 (7.7) |
Interval between ECM surgery and interventional cardiology procedure (months; median, range) | 12.6 (2.7–23.0) |
(Group A) Valve repair | 0 |
(Group B) Septal reconstruction | 0 |
(Group C) Arterial plasty | 8 |
(Group D) Other | 0 |
ECM functional failure at follow-up (patients) | 28 (27.2) |
(Group A) Valve repair | 21 |
(Group B) Septal reconstruction | 0 |
(Group C) Arterial plasty | 6 |
(Group D) Other | 1 |
Evidence of calcification (patients) | 1 (0.9) |
Clinical evidence of persistent systemic inflammation (patients) | 2 (1.9) |
Variable . | Patients, n (%) . |
---|---|
Total patients | 103 (100) |
Early outcome | |
Major complication during hospitalization (patients) | 26 (25.2) |
Major complication during hospitalization related to Cormatrix (patients) | 0 (0) |
Median ICU stay (days) | 3 (1–90) |
Median IPPV duration (h) | 20 (1–2160) |
Median hospital stay (days) | 15 (6–115) |
Hospital death (patients) | 4 (3.8) |
Hospital death, related to Cormatrix (patients) | 0 (0) |
Late outcome | |
Follow-up length (months; median, range) | 23.33 (0.3–55.23) |
Late death (patients) | 4 (3.8) |
Late death, related to ECM (patients) | 0 (0) |
Reoperations (patients) | 19 (18.4) |
Reoperations, related to ECM (patients) | 6 (5.8) |
Interval between ECM surgery and reoperation (months; median, range) | 25.2 (2.5–34.1) |
(Group A) Valve repair | 6 |
(Group B) Septal reconstruction | 0 |
(Group C) Arterial plasty | 0 |
(Group D) Other | 0 |
Interventional cardiology procedures (patients) | 11 (10.7) |
Interventional cardiology procedures, related to ECM (patients) | 8 (7.7) |
Interval between ECM surgery and interventional cardiology procedure (months; median, range) | 12.6 (2.7–23.0) |
(Group A) Valve repair | 0 |
(Group B) Septal reconstruction | 0 |
(Group C) Arterial plasty | 8 |
(Group D) Other | 0 |
ECM functional failure at follow-up (patients) | 28 (27.2) |
(Group A) Valve repair | 21 |
(Group B) Septal reconstruction | 0 |
(Group C) Arterial plasty | 6 |
(Group D) Other | 1 |
Evidence of calcification (patients) | 1 (0.9) |
Clinical evidence of persistent systemic inflammation (patients) | 2 (1.9) |
ECM: extracellular matrix; ICU: intensive care unit; IPPV: intermittent positive airway ventilation.
There was no evidence of ECM calcification at follow-up in all patients, either on reoperation and postoperative imaging, or on post-surgery histological analysis (Fig. 3)
Finally, in 2 patients from two different centres we reported the persistence of clinical signs of inflammation (persistent fever and increase of PCR). A 5-month old patient after tetralogy of Fallot repair developed increased PCR and fever not responsive to antibiotic therapy; 2 months later, he presented with pulmonary haemorrhage due to rupture of a right lower lobe PA branch of possible infective aetiology and required urgent right lobectomy. Another patient with DiGeorge syndrome and AoV regurgitation, who previously underwent AoV repair by means of leaflet extension, was readmitted 29 months later with diagnosis of acute endocarditis. Despite negative blood cultures and large-spectrum antibiotic therapy, the clinical situation did not improve. Thus, he underwent AoV replacement, with complete removal of the reconstructed leaflets. Microbiological evaluation did not demonstrate bacterial growth. However, after AoV replacement, the patient recovered, with discontinuation of persistent fever and normalization of PCR.
Kaplan–Meier analysis (Fig. 4) on late outcomes of 103 patients undergoing surgery with the SIS–ECM scaffold has shown that:
freedom from ECM-related surgical reoperation (Fig. 4A) was significantly lower in Category A (P = 0.04202: C versus B, HR 0.077, CI 0.001–8.952; D versus B, HR 0.851, CI 0.007–99.202; A versus B, HR 1.318, CI 0.035–49.543);
freedom from ECM-related interventional cardiology procedures (Fig. 4B) was significantly lower in Category C (P = 0.4524: C versus B, HR 2.619, CI 0.108–63.590; D versus B, HR 1.528, CI 0.019–123.197; A versus B, HR 0.217, CI 0.003–17.541);
freedom from functional failure and any adverse events (Fig. 4C and D) were greater in Categories B, C and D when compared with Category A, despite being non-significant (P = 0.1770).

Kaplan–Meier (KM) analysis on late outcomes of 103 patients undergoing surgery with the SIS–ECM scaffold. (A) KM curve shows significantly lower freedom from surgical reoperations in Category A (P = 0.04202: C versus B, HR 0.077, CI 0.001–8.952; D versus B, HR 0.851, CI 0.007–99.202; A versus B, HR 1.318, CI 0.035–49.543). (B) KM curve shows significantly lower freedom from ECM-related interventional cardiology procedures in Category C (P = 0.4524: C versus B, HR 2.619, CI 0.108–63.590; D versus B, HR 1.528, CI 0.019–123.197; A versus B, HR 0.217, CI 0.003–17.541). (C) KM curve shows greater (not significant, P = 0.1770) freedom from functional failure in Categories B, C and D when compared with Category A. (D) KM curve shows greater (not significant, P = 0.1770) freedom from any adverse events in Categories B, C and D when compared with Category A. SIS: small intestine submucosa; ECM: extracellular matrix.
Calcification was absent in all but one patient. Inflammation occurred only in 2 cases in Category A and did not reach statistical significance (P = 0.2857).
At univariate Cox regression analysis, we found that:
ECM surgical procedures on AoV and semilunar valves were significantly related to reoperation at follow-up (P = 0.0056, HR 16.010, CI 2.254–113.698, and P = 0.0468, HR 7.277, CI 1.029–51.472, respectively);
ECM surgery in infants aged <12 months and procedures on branch PAs and PAs (including the MPA) were significantly related to interventional cardiology procedures at follow-up (P = 0.0474, HR 5.048, CI 1.019–25.020; P = 0.0159, HR 5.827, CI 1.392–24.402 and P = 0.0735, HR 15.858, CI 0.769–327.023, respectively);
ECM surgery on valves (surgical Category A), including AoV, PV and both semilunar valves, was significantly related to functional failure at follow-up [P = 0.0664, HR 2.193 (0.948–5.072); P = 0.0003, HR 4.162 (1.915–9.047); P < 0.0001, HR 5.280 (2.373–11.745), respectively];
similarly, ECM surgery on valves (surgical Category A), including AoV, PV and both semilunar valves, was significantly related to greater incidence of any combination of adverse events at follow-up (P = 0.0632, HR 2.198, CI 0.958–5.045; P = 0.0014, HR 3.521, CI 1.624–7.631; P < 0.0001, HR 4.935, CI 2.217–10.985, respectively).
Multivariate Cox regression analysis showed that only surgery on semilunar valves was a statistically significant predictor of functional failure and occurrence of adverse events at follow up (P < 0.0001).
When analysing specifically the outcome of two different techniques in the use of the scaffold in valve repair (Fig. 1A), we found that freedom from surgical reoperation, functional failure and any adverse events (related to SIS–ECM) were greater in the leaflet augmentation technique, when compared with the leaflet extension technique, albeit not statistically significant (P = 0.4048, 0.1262 and 0.1255, respectively). Finally, the Aristotle Basic Score of surgical procedure did not influence significantly the early and late outcomes of the SIS–ECM scaffold.
DISCUSSION
Currently, surgical repair of most CHDs is successfully performed at an early age in order to reduce the negative drawbacks of palliation and unphysiological circulation. The use of prosthetic material in surgical repair of complex CHD is often mandatory for reconstruction of missing cardiac structures or dysplastic valves, or enlargement of hypoplastic arteries. The need of regenerative biological tissues for vascular and cardiac structures is compelling in paediatric patients with CHD, who often need reoperation because of mismatch between prosthetic materials and the child's growth. Tissue engineering and regenerative medicine is a multidisciplinary effort to reconstruct functional tissues, with cell- or bioscaffold-based approaches [5, 6]. Major concerns are related to possible patch dehiscence, calcification, degeneration, thickening and retraction. Several authors have reported on the use of several materials for cardiac reconstruction [1–4], with various short- and long-term results. The ideal patch should be a bioscaffold, which would be pliable, haemostatic and resistant to tearing; should not shrink or calcify; should not induce an inflammation cascade and should grow with the patient and promote regeneration of native tissue. Bioscaffolds can be composed of intact ECM, or individual components of ECM such as collagen, laminin or hyaluronan [5].
Collagen accounts for more than 90% of ECM, mostly type I collagen [5]. Depending on various factors including kind of manufacture, decellularization process efficiency, use of chemical cross-linking agents and the age of source tissue from which ECM is harvested, the host response to an implanted ECM scaffold may vary from unacceptable to excellent [5]. When placed in contact with healthy surrounding tissue and subjected to appropriate mechanical loads, biological ECM scaffolds are first infiltrated by neutrophil and mononucleate cells as the natural inflammatory host response. Subsequently, ECM degrades and endogeneous stem/progenitor cells are recruited to the treatment site, neomatrix deposition occurs and the remodelling process culminates with the deposition of newly formed site appropriate tissue. Biological scaffold composed of SIS–ECM have been shown to be effective in experimental [4, 7, 12, 13] and human clinical applications [8, 9]. When compared with ECM scaffolds with dense and compact ultrastructure such as dermal ECM, this material derived from a less dense tissue such as SIS is supposed to provide an interim bioscaffold that can be easily decellularized and rapidly degraded in vivo [5], enabling the patient's own cells to repopulate and repair tissues more rapidly. However, despite the initial great enthusiasm, some recent reports have re-dimensioned the general expectations [14, 15].
For this reason, we have embarked on a prospective multicentre clinical study with the aim of evaluating as objectively as possible our results with clinical application of the SIS–ECM scaffold. To the best of our knowledge, this is the largest reported clinical series of patients who have undergone surgical application of SIS–ECM for repair of CHD. Our clinical results have been evaluated according to different surgical categories in order to outline the best application for such a material. In our series, several types of CHD have been included, despite anatomical complexity or age. We included any patient in whom the potential of growth could be highly effective to reduce the incidence of reintervention.
In our experience, this material resulted in being pliable and haemostatic, and no major operative complications have been reported. It is widely known that long-term calcification is one of the major drawbacks of conventional biological materials [16]. However, in our experience, calcification has never been detected yet, either at reoperations or at follow-up imaging or at medium-term histological analysis after explantation (Fig. 3). As reported elsewhere [13, 17], our clinical series seems to confirm that at midterm follow-up, SIS–ECM may be resistant to the degenerative calcification process.
In our series, the majority of patients underwent arterial wall patch plasty, either on the aortic or pulmonary sides (surgical Category C, 55.6%), and valve reconstruction (surgical Category A, 28.2%), especially on semilunar valves. In fact, the lack of an ideal prosthetic substitute in CHD repair is still crucial, especially for reconstructing vessel and valves. In a clinical series of 472 neonates with an interrupted aortic arch, McCrindle et al. [18] demonstrated that aortic arch repair with a polytetrafluoroethylene (PTFE) interposition graft or patch augmentation was associated with a not negligible incidence of reintervention for late aortic arch obstruction. In addition, Ashcraft et al. [19] reported a 30% recoarctation after a Norwood procedure in a series of 117 patients, and the use of bovine pericardium showed the greatest risk for potential recoarctation (HR 1.81, P = 0.09). On the contrary, in our experience, among 8 cases of aortic arch repair with the SIS–ECM scaffold, no case of restenosis or aneurysm has occurred in aortic position at medium-term follow-up. Thus, SIS–ECM may be a good alternative to heterologous pericardium whenever pulmonary homograft material (which is usually utilized by most centres) is not available for aortic reconstruction in neonates. In particular, ascending aortic reconstruction in patients with supravalvar aortic stenosis has been characterized by excellent late outcomes in our series.
It is widely known that native and acquired types of PA branch stenosis can often occur after complex CHD repair or palliation, and represent a life-threatening condition often necessitating further surgical or interventional treatment [20]. There are no significant differences in late outcomes of PA branch plasty regardless of the kind of material that can be used [20]. Nevertheless, experienced centres report satisfactory early and late outcomes with reconstruction of peripheral PAs in complex CHDs such as tetralogy of Fallot (TOF) with pulmonary atresia, and malformative syndromes, using PA homograft [21], whose characteristics and pliability allow PA reconstruction also in neonates and infants. Again, as mentioned above, SIS–ECM may constitute a possible alternative to conventional patches. However, in our experience, late results of PA plasty, especially in infants, have been unsatisfactory, since most patients required balloon catheter dilatation for restenosis, after a median time of 12.6 months following a SIS–ECM surgical implant (Table 2). As described elsewhere [22], we speculate that, especially in neonates and infants, in whom PAs can be very small, and in patients with single-ventricle physiology, in which the pulmonary arterial pressure is not pulsatile, the typical early inflammatory phase of the SIS–ECM scaffold and the consequent increased thickness of the SIS–ECM reconstructed PA wall may contribute to decrease in the internal diameter of the PA. In such a situation, the pulmonary blood flow in the reconstructed vessels can be reduced and this may contribute to cause late obstruction. Thus, we currently avoid using the SIS–ECM scaffold for reconstruction of small PAs in infants.
Different techniques of semilunar valve repair have been proposed in the current literature so as to preserve the native valve, such as the leaflet extension technique with pericardium [1, 10], which has provided generally good early results in the acquired and congenitally affected AoV. It is well known that the limiting factor of the long-term function of extended aortic leaflets is the durability of the material used for extensions. Various biological materials have been employed for AoV repair [1, 2, 10], all showing that in the long term there is a structural valve degeneration caused by leaflet fibrosis, thickening and calcification. Findings on explanted PTFE valve substitutes have shown preserved pliability of leaflets and neointimal and endothelium formation on histological examination [3, 4]. However, the long-term function of PTFE as a valve substitute is still unclear.
In our experience, valve repair was accomplished with an ECM scaffold in 38 cases, mostly on semilunar valves. However, 5 of 13 patients (38.4%) who have undergone AoV repair required reoperation for AoV replacement after a median interval of 25.2 months (Table 2). When compared with Zaidi et al. [15], in which the median length of time in situ was about 2 months, in our series the scaffold did perform better for longer time. However, its durability seems lower than autologous pericardium [1, 15]. To date, we have not experienced any surgical reintervention on the PV, and only a minority required catheter reintervention. Nevertheless, in Category A (valve repair), we have found a high rate of functional failure, with a progression to regurgitation or stenosis detected at 2D echocardiographic follow-up in 21 out of 38 patients (55.2%), and for this reason, we have discontinued SIS–ECM scaffold application for semilunar valve repair. It is widely known that semilunar valve leaflet extension is a widely used technique, but with unsatisfactory long-term results. Thus, condemning the material for a bad technique would be making a dangerous shortcut. However, in our experience, the SIS–ECM scaffold does not appear to correct the drawbacks of this valve repair technique.
As far as results of use of the ECM scaffold in atrioventricular valve reconstruction, we report a better late outcome in a small series of 7 patients, since only 1 of these patients required valve replacement. Thus, as mentioned above, whenever autologous pericardium or other conventional patches are not available anymore, a SIS–ECM patch may be a valid alternative in atrioventricular valve (AVV) malformations in neonates and infants. In fact, in these low-weight patients, patch augmentation may be difficult since even autologous pericardium may be too thick and bulky in these ‘mini’-anatomies. Thus, the flexibility and thinness of the SIS–ECM scaffold may be advantageous, and the possibility of being recellularized by autologous cells may be promising. In addition, as suggested elsewhere [15], we speculate that surgical inclusion of the SIS–ECM scaffold inside viable native valve tissue (patch augmentation, Fig. 1A) may facilitate tissue regeneration and remodelling. On the contrary, leaflet extension with ECM on dysplastic tissue valve (as often occurs on the semilunar valve position) may result only in inflammatory cell infiltration and neointimal layer thickening with no cell remodelling at all.
We are reporting excellent outcomes for septation of atrial or ventricular chambers, with no dehiscence or bulging of the patch into chambers or any other complications related to the SIS–ECM scaffold. Even though these encouraging outcomes may be related to surgical technique rather than to the scaffold, we believe that the proven regenerative potential [6] may be useful particularly when dealing with complex repair, such as anomalous pulmonary venous return, or complex transposition of the great arteries or a double-outlet right ventricle with uncommitted VSD, in which intracardiac patch rerouting may expose the patient to the risk of progressive obstruction and subsequent reoperation, as reported in the literature [23].
Finally, in our series, SIS–ECM has been employed only a few times for pericardial reconstruction. Despite successful reports on pericardial regeneration of SIS–ECM [24], currently we are not considering SIS–ECM as a pericardial substitute since other materials such as anti-adhesion glues are used with excellent results [25].
In conclusion, surgical indications for SIS–ECM need to be better defined, and still more issues need to be explored and understood. ECM scaffolds consist of the structural and functional molecules secreted by the resident cells of each tissue and organ from which they are prepared. When implanted in the host tissue, proteolytic degradation of the ECM scaffold by immunomodulatory pro-remodelling macrophages produces biochemical triggers that attract progenitor cells and, together with the surrounding biomechanical and fluid dynamic environment, induce progenitor cell proliferation and phenotypic maturation. Thus, tissue regeneration may not occur as expected on dysplastic fibrotic tissues or on valves after percutaneous balloon dilatation, since these environments are not physiologically or anatomically ‘normal’. This issue may be critical especially in CHD to explain outcomes, success and failures. In this series, surgical use of SIS–ECM in CHD repair has not fulfilled the great preoperative expectations. It is characterized by good late outcomes on septal and aortic wall reconstruction, but suboptimal functional late performance on reconstruction of semilunar valve leaflets or small PAs. Longer follow-up and larger clinical experience are needed to better understand the mechanism of cell and tissue regeneration and to assess which is the optimal surgical indication for the SIS–ECM scaffold.
SUPPLEMENTARY MATERIAL
Supplementary material is available at ICVTS online.
Conflict of interest: none declared.
ACKNOWLEDGEMENTS
We wish to thank Fabio Zucchetta (Section of Cardiac Surgery, Department of Cardiac, Thoracic and Vascular Sciences, University of Padova, Italy) for diagrams in Fig. 1, and Marny Fedrigo (Section of Cardiovascular Pathology, Department of Cardiac, Thoracic and Vascular Sciences, University of Padova, Italy) for histologic images in Fig. 3.
REFERENCES
APPENDIX. CONFERENCE DISCUSSION
Dr T. Karl(Brisbane, Australia): This is convincing analysis of a large clinical experience now with approximately 200 patient years' follow-up time. As I understand it, the striking findings included a somewhat suboptimal performance of this patch material for aortic valve leaflet extension and pulmonary artery reconstruction, which are two frequently performed surgical procedures in any pediatric cardiac unit. I was wondering how patients were recruited for this study, and whether other patch materials were used concurrently in the same institutions contributing?
Dr Padalino: This is a multicentric prospective study, and actually there was no selection of patients. The only selection we made was the decision of recruiting patients in which the potential of regeneration could be highly desirable in decreasing the potential for reoperation. As far as other materials used in our centre, we routinely use pulmonary homograft and saphenous vein for reconstruction of arterial wall, heterologous pericardium for septal defects but we don't have any detailed information about late outcomes of these materials in our hands since we have not studied these cases prospectively.
Dr Karl: So your conclusion was that this patch material performed not better or not worse than other patch materials used in aortic cusp extension?
Dr Padalino: It doesn't perform as well as expected. It disappointed our expectations, because actually we have re-operated most of repaired aortic valves after about two years. Being our aim to provide the patients with a definitive or at least long lasting solution, in light of these results, in our opinion the outcomes on valve repair are not satisfactory and thus we are not incline to use it since it does not perform better.
Dr Karl: You presented some histologic analysis from one patient, which I believe is a postmortem study. Do you have any information on explanted tissue from patients who had failure of the patch material either in cusp extension or any other application? I would think that would be the most critical part of a study like this.
Dr Padalino: Histologic analyses are not from post mortem study, but after explanation for redo surgery on aortic valve.
In Padova we have another patient who had aortic valve replacement and we have similar findings as far as fibrosis and inflammatory cells of native repaired leaflets. I know that also in Bologna they have similar findings on aortic valve leaflets repaired with Cormatrix. I don't know exactly in Ancona.
Dr J. Kluin(Utrecht, Netherlands): I just want to make one comment. We are in the midst of an animal study in which we put in valved conduits in the pulmonary position in sheep, and the results for the pulmonary wall seem to be good, but we have great concerns about using this material for the leaflets. So this is consistent with your findings.
Dr Padalino: Well, I actually know there are some other surgeons around the world who are using reconstruction of the pulmonary leaflet, and they claim to have better results than we have. But I suspect there is also a different technique, so I guess that we also have to evaluate the impact of different surgical techniques on outcomes.
Dr Kluin: I do not think it is a technical point, I think it is the material.
Dr Padalino: I believe that this may be possible, but I think that surgical technique may have a role.
Dr H. Dave(Zurich, Switzerland): I just wanted to reinforce what others just reported. We have retreated from using this patch material for aortic valve reconstruction because while the reconstruction looks very good immediate postoperatively, in a significant percentage of patients, it degenerated within six months and we had to repair these valves again. Considering this, it is a little bit scary to think of using it for RVOT or for VSDs, because the scaffold disappears, and the problems of RVOT would become apparent much later. So what do you think is a safe indication to use this material?
Dr Padalino: Well, actually I am aware of the fact that this scaffold has been used a lot of times for reconstruction of myocardial wall in adult cardiac surgery for left ventricular wall reconstruction in LV aneurysms; in addition, there are some unpublished yet experimental data from Dr Morales when he was in Texas, who was reporting very good results with reconstruction of myocardial wall in experimental animal models, also on contractility of the patch.
My personal belief is that this patch may well repopulate, so I would not be scared of residual shunts for VSDs due to reabsorption of the ECM patch; however it is not clear, at least not to me, what the actual origin of the cells that are repopulating the scaffold, in other words if they are coming from surrounding tissues or from blood circulating stem cells.
Author notes
Presented at the 28th Annual Meeting of the European Association for Cardio-Thoracic Surgery, Milan, Italy, 11–15 October 2014.