-
PDF
- Split View
-
Views
-
Cite
Cite
Hyoung Woo Chang, Soo Hwan Kim, Kyung-Hwan Kim, Yong Jin Kim, Combined anti-calcification treatment of bovine pericardium with amino compounds and solvents, Interactive CardioVascular and Thoracic Surgery, Volume 12, Issue 6, June 2011, Pages 903–907, https://doi.org/10.1510/icvts.2010.259747
- Share Icon Share
Abstract
The objective of this study was to evaluate the effect of treatment with amino compounds and solvents in vivo on calcifications of glutaraldehyde (GA)-fixed pericardium. Groups of bovine pericardium samples were fixed with 0.5% GA. We used urazole and glutamate to neutralize the free aldehyde and some solvents (ethanol with octanol or octanediol) to reduce the phospholipid content in the bovine pericardial tissue. Tensile strength and thermal stability were evaluated before implantation. Twelve weeks after rat subdermal implantation, the pericardial samples were harvested from eight juvenile rats. Urazole [calcium (Ca2+): 11.86±2.85 μg/mg; inorganic phosphorus (IP): 32.59±7.73 μg/mg] or glutamate (Ca2+: 7.95±1.21 μg/mg; IP: 21.76±3.48 μg/mg) alone significantly decreased the Ca2+ and IP concentrations (without any anti-calcification treatment, Ca2+: 277.85±17.51 μg/mg; IP: 147.07±8.32 μg/mg), but when used with organic solvents, the Ca2+ and IP concentrations were the lowest (Ca2+: 0.05±0.04 μg/mg; IP: 3.36±0.61 μg/mg). After anti-calcification treatment, the calcifications in microscopic images were dramatically decreased. Anti-calcification treatment with glutamate, urazole, and solvents did not worsen the physical properties of bovine pericardium, and significantly prevented in vivo calcifications compared to GA fixation only. There should be additional studies done to understand the other mechanism underlying xenograft tissue calcification.
1. Introduction
Advances in cardiac surgery has led to an increase in surgeries for congenital and acquired heart disease, and redo operations, thus necessitating biologic or synthetic prostheses to re-build cardiovascular structures [1]. Currently, xenograft prosthetic materials are most widely used; however, xenograft prosthetic materials have many drawbacks, such as low durability, degeneration, calcifications, and difficulty in operative manipulation [2]. Recent targets of research in xenograft prosthetic materials are to improve their durability with anti-calcification resistance and to preserve mechanical properties of processed grafts.
The most frequently used fixation material is glutaraldehyde (GA) solution. But several years after GA fixation, xenograft prostheses usually become severely calcified. The mechanism underlying in vivo calcification of bioprostheses is not well understood; however, phospholipid-based cell membranes are known to be a key material for calcification [3]. GA, which is used in tissue fixation, is also known to play a role in calcification [4]. Specifically, the free aldehyde groups that did not form cross-links with tissue collagen are understood to have cytotoxicity and augment tissue calcifications most [5].
In this set of experiments, there were two key features of potential alternative use in anti-calcification treatment: the addition of proposed chemicals (urazole and glutamate) to neutralize the leachable free aldehyde groups in the bioprosthetic tissue; and tissue treatment with organic solvents (ethanol, octanol, and octanediol) to remove residual phospholipid content in the bioprosthesis [6].
A rat subdermal implantation model, which we used, is one of the widely accepted in vivo simulation models. After treating fresh bovine pericardial tissue with several combinations of methods, the physical properties of treated tissue were assessed to confirm that the anti-calcification treatment did not worsen the physical properties. Small (1×1 cm) pieces of treated bovine pericardial tissue were surgically implanted below the dermis in the backs of rats. After 12 weeks of growth, all of the animals were euthanized and the bovine pericardial tissue pieces were extracted. The tissue concentrations of calcium (Ca2+) and inorganic phosphorus (IP) were measured, and the tissues were examined microscopically to determine the Ca2+ accumulation pattern.
2. Materials and methods
2.1. Anti-calcification treatments
Fresh bovine pericardium, which was fixed with 0.5% GA alone, and stored in 0.25% GA solution, was used as the control group. The control group samples were examined for physical properties and implanted in the subdermal space in the backs of rats, and 12 weeks later the samples were harvested. The Ca2+ and IP concentrations were also measured. The number of overall experimental groups was six.
Bovine pericardium which was used in this experiment, was obtained immediately after butchery, and was immersed in a 0.1 M phosphate-buffered saline (PBS) solution (pH 7.4). The sample was preserved in a cooler and delivered to the laboratory. The sample tissue was irrigated with normal saline, and the adherent surface connective tissue and fat material were surgically removed with blunt or sharp dissection.
For GA fixation alone, tissue samples were fixed in PBS buffered 0.5% GA solution (pH 7.4) for five days and were fixed with 0.25% GA solution for an additional week at room temperature. Then, the samples were completely irrigated and sent for the following procedure.
For GA fixation followed by ethanol with octanol or octanediol treatment, the tissue samples were fixed in PBS buffered 0.5% GA solution for five days and immersed in PBS buffered 0.5% GA with 77.5% ethanol and 2.5% octanol or 2.5% octanediol for 48 h. The samples were additionally, fixed in 0.25% GA solution for one week at room temperature. Then, the samples were completely irrigated and sent for the following procedure.
For detoxification with urazole, GA-fixed or fixed-and-solvent-treated tissue samples were treated in PBS buffered 0.1 M urazole solution for one week at room temperature. Then, the samples were immersed in PBS buffered 0.1 M sodium borohydride solution for 48 h at room temperature.
For detoxification with L-glutamic acid (glutamate), GA-fixed or fixed-and-solvent-treated tissue samples were treated in PBS buffered 0.12 M glutamate for 48 hours at room temperature.
In summary, there were seven groups in this set of experiments, as presented in Table 1 .
Abbreviations or | Full name |
acronyms | |
GA | Glutaraldehyde |
GAO | Glutaraldehyde fixation only |
GAU | Glutaraldehyde fixation+treatment with urazole |
GA-OL-U | Glutaraldehyde fixation and treatment with ethanol |
and octanol, followed by urazole detoxification | |
GA-DIOL-U | Glutaraldehyde fixation and treatment with ethanol |
and octanediol, followed by urazole detoxification | |
GAG | Glutaraldehyde fixation+treatment with glutamate |
GA-OL-G | Glutaraldehyde fixation and treatment with ethanol |
and octanol, followed by glutamate detoxification | |
GA-DIOL-G | Glutaraldehyde fixation and treatment with ethanol |
and octanediol, followed by glutamate detoxification | |
IP | Inorganic phosphorus |
PBS | Phosphate buffered saline |
Abbreviations or | Full name |
acronyms | |
GA | Glutaraldehyde |
GAO | Glutaraldehyde fixation only |
GAU | Glutaraldehyde fixation+treatment with urazole |
GA-OL-U | Glutaraldehyde fixation and treatment with ethanol |
and octanol, followed by urazole detoxification | |
GA-DIOL-U | Glutaraldehyde fixation and treatment with ethanol |
and octanediol, followed by urazole detoxification | |
GAG | Glutaraldehyde fixation+treatment with glutamate |
GA-OL-G | Glutaraldehyde fixation and treatment with ethanol |
and octanol, followed by glutamate detoxification | |
GA-DIOL-G | Glutaraldehyde fixation and treatment with ethanol |
and octanediol, followed by glutamate detoxification | |
IP | Inorganic phosphorus |
PBS | Phosphate buffered saline |
Abbreviations or | Full name |
acronyms | |
GA | Glutaraldehyde |
GAO | Glutaraldehyde fixation only |
GAU | Glutaraldehyde fixation+treatment with urazole |
GA-OL-U | Glutaraldehyde fixation and treatment with ethanol |
and octanol, followed by urazole detoxification | |
GA-DIOL-U | Glutaraldehyde fixation and treatment with ethanol |
and octanediol, followed by urazole detoxification | |
GAG | Glutaraldehyde fixation+treatment with glutamate |
GA-OL-G | Glutaraldehyde fixation and treatment with ethanol |
and octanol, followed by glutamate detoxification | |
GA-DIOL-G | Glutaraldehyde fixation and treatment with ethanol |
and octanediol, followed by glutamate detoxification | |
IP | Inorganic phosphorus |
PBS | Phosphate buffered saline |
Abbreviations or | Full name |
acronyms | |
GA | Glutaraldehyde |
GAO | Glutaraldehyde fixation only |
GAU | Glutaraldehyde fixation+treatment with urazole |
GA-OL-U | Glutaraldehyde fixation and treatment with ethanol |
and octanol, followed by urazole detoxification | |
GA-DIOL-U | Glutaraldehyde fixation and treatment with ethanol |
and octanediol, followed by urazole detoxification | |
GAG | Glutaraldehyde fixation+treatment with glutamate |
GA-OL-G | Glutaraldehyde fixation and treatment with ethanol |
and octanol, followed by glutamate detoxification | |
GA-DIOL-G | Glutaraldehyde fixation and treatment with ethanol |
and octanediol, followed by glutamate detoxification | |
IP | Inorganic phosphorus |
PBS | Phosphate buffered saline |
2.2. Tensile strength test
Ten pericardial pieces of each experimental group were prepared by cutting the pericardium into 0.5×5 cm pieces. The tensile strength was measured for the cut surface area (5 mm×thickness), and the average value for each group was calculated. The reference value was obtained by measuring the tensile strength of fresh bovine pericardium in the same way. Tissue pieces were pulled at each end by a specialized machine (Pushpull Electronic Stand; M-tech, Seoul, Korea). The force which was applied to the tissue ends were measured with a digital force gauge (model DS2-50N; IMADA, Tokyo, Japan). The load speed was 100 mm/min. Drawing a graph which delineated tissue length and tissue strain (tension force), we determined the acute decrement of tissue strain, and the force value at the point of acute decrement was considered to indicate the tensile strength. Tissue thickness was measured at three discrete points using an Electronic Micrometer (Quick-Mini 700-117; Mitutoyo, Tokyo, Japan). Thus, the force which was applied to the each cut surface area (5 mm×thickness) had MPa unit.
2.3. Thermal stability test
Both fresh bovine pericardium and pericardium treated in various ways were cut as 8×30 mm pieces and a 95 g weight was applied to every pericardial piece to give continuous tensile strength. Then, these pieces were immersed in 55 °C distilled water and the temperature of the water was gradually increased by 2 °C/min and the temperature points at which each pericardial piece shrank abruptly were recorded and compared.
2.4. Rat subcutaneous implantation
The entire experimental procedure was undertaken under the approval of the International Animal Care and Use Committee (IACUC) at the Clinical Research Institute of Seoul National University Hospital (IACUC No. 07-0214). Eight three-week-old female Sprague–Dawley rats were prepared. The average weight of the rats was 97.2±5.0 g. Zoletil and Rompun were injected into the peritoneal cavity for anesthesia. Hair on the back was removed and the back skin was sterilized with ethyl alcohol. Prophylactic antibiotics (20 mg/kg of cefazolin) were injected intradermally. Six separate incisions were made on the back dermis, and subdermal loose connective tissue was dissected. Saline-irrigated pericardial pieces (1×1 cm) were implanted into each subdermal space. In each treatment method group, eight pericardial pieces were implanted. After the implantation, the incisions were sutured with 3-0 nylon interrupt stitches. The rats were raised for 12 weeks, then painlessly sacrificed with carbon dioxide gas. Each rat was weighed, and the hair on the back was removed. Through the same incision formed during the implantation, the implanted pericardial pieces were extracted. Subdermal connective tissue was carefully removed from the pericardial pieces, and the pieces were irrigated with normal saline. The pieces were stored in 2% benzyl alcohol solution for the next procedure (Ca2+ and IP quantification).
2.5. Measurement of calcium concentration
The Ca2+ concentration was measured in eight pericardial pieces for each treatment method group. The harvested pericardial pieces were irrigated with normal saline and dried at 90 °C for 48 h, and weighed. Dry pieces were pre-treated with hydrochloric acid (5 N HCl solution) and immersed in 1 M PBS. The o-cresolphthalein complexone (OCPC) method was used to measure the concentration of Ca2+ (Automatic Biochemistry Analyzer Hitachi 7070; Hitachi, Tokyo, Japan). And the Ca2+ concentrations of each treatment method group were compared.
2.6. Measurement of IP concentration
The IP concentration was also measured in eight pericardial pieces for each treatment method group. The harvested pericardial pieces were irrigated with normal saline and dried at 90 °C for 48 h, and weighed. The Fiske Subbarow method (Automatic Biochemistry Analyzer Hitachi 7070) was used.
2.7. Light microscopic examination
Pericardial tissues from each treatment group were examined under light microscopy before the implantation and after the harvest. The pericardial pieces were cut in 2–3 mm width and immersed in Dubosqc-brasil solution for 1 h, and fixed in 10% formaldehyde solution. Paraffin-embedded samples were prepared and cut in 2–4 μm thicknesses and stained. Preimplantation pericardial pieces were stained with hematoxylin and eosin (H&E), and postharvest pieces were stained with both H&E and the von Kossa method. Calcification of pericardial tissue is usually detectable with H&E stain, but the von Kossa method helps non-specialists in pathology distinguish calcification more easily because Ca2+ is stained as a brown or black color.
2.8. Statistical analysis
Comparing more than two groups, we used one-way analysis of variance (ANOVA), and the Tukey b test as a post-hoc test to rank the values and evaluate the significance of differences. We always considered a P-value <0.05 to indicate statistical significance. SPSS (SPSS 12.0 for Windows; SPSS Inc, Chicago, IL, USA) was used for statistical analyses.
3. Results
The results of the tensile strength and thermal stability test are summarized in Table 2 , and the Ca2+ and IP concentrations of harvested pericardial tissues are summarized in Table 3 .
Tensile stress at break (MPa) (min-max) | Sharp deflection point (°C) (min-max) | |
Fresh bovine pericardium | 13.2±6.1 (3.4–27.9), n=60 | 69.4±1.5 (66.3–71.3), n=15 |
(1) GAO | 16.1±6.3 (7.8–34.7), n=50 | 86.8±1.1 (86.3–88.8), n=5 |
(2) GAU | 16.9±2.5 (12.9–21.2), n=10 | 81.3±1.8 (78.8–83.8), n=5 |
(3) GA-OL-U | 17.0±6.4 (9.1–25.6), n=10 | 83.8±0, n=5 |
(4) GA-DIOL-U | 18.5±12.5 (4.6–30.7), n=10 | 83.3±1.1 (81.3–83.8), n=5 |
*ANOVA of (1), (2), (3), and (4) | P=0.786 | P=0.000 |
(5) GAG | 18.0±14.9 (3.8–35.5), n=10 | 84.8±1.4 (83.8–86.3), n=5 |
(6) GA-OL-G | 15.1±4.8 (11.0–25.3), n=10 | 85.3±1.4 (83.8–86.3), n=5 |
(7) GA-DIOL-G | 16.5±9.5 (6.3–32.1), n=10 | 86.3±0, n=5 |
*ANOVA of (1), (5), (6), and (7) | P=0.873 | P=0.046 |
Tensile stress at break (MPa) (min-max) | Sharp deflection point (°C) (min-max) | |
Fresh bovine pericardium | 13.2±6.1 (3.4–27.9), n=60 | 69.4±1.5 (66.3–71.3), n=15 |
(1) GAO | 16.1±6.3 (7.8–34.7), n=50 | 86.8±1.1 (86.3–88.8), n=5 |
(2) GAU | 16.9±2.5 (12.9–21.2), n=10 | 81.3±1.8 (78.8–83.8), n=5 |
(3) GA-OL-U | 17.0±6.4 (9.1–25.6), n=10 | 83.8±0, n=5 |
(4) GA-DIOL-U | 18.5±12.5 (4.6–30.7), n=10 | 83.3±1.1 (81.3–83.8), n=5 |
*ANOVA of (1), (2), (3), and (4) | P=0.786 | P=0.000 |
(5) GAG | 18.0±14.9 (3.8–35.5), n=10 | 84.8±1.4 (83.8–86.3), n=5 |
(6) GA-OL-G | 15.1±4.8 (11.0–25.3), n=10 | 85.3±1.4 (83.8–86.3), n=5 |
(7) GA-DIOL-G | 16.5±9.5 (6.3–32.1), n=10 | 86.3±0, n=5 |
*ANOVA of (1), (5), (6), and (7) | P=0.873 | P=0.046 |
When four groups related to urazole were compared (GAO, GAU, GA-OL-U, and GA-DIOL-U), there was no significant difference in tensile strength (MPa). Similarly, the GAO, GAG, GA-OL-G, and GA-DIOL-G groups were compared; there was no significant difference in tensile strength. The results of the thermal stability test are summarized in Table 3. Comparing the GAO, GAU, GA-OL-U, and GA-DIOL-U groups, the temperature points of acute shrinkage revealed some statistically significant differences. The GAU group showed the lowest sharp deflection point (mean, 81.3 °C), and the GA-OL-U and GA-DIOL-U groups were in the middle (mean, 83.3 °C for GA-OL-U and 83.8 °C for GA-DIOL-U), and the GAO group showed the highest sharp deflection point (mean, 86.8 °C). The difference was statistically significant. All of the temperature points of acute deflection are not assumed to exist under in vivo circumstances. Thus, we reasoned that these differences in sharp deflection points had no clinical significance. In the same manner, the GAO, GAG, GA-OL-G, and GA-DIOL-G groups were compared. A post-hoc test (Tukey b test) showed no significant differences among the values of the sharp deflection points. ANOVA, analysis of variance; acronyms for group names were described in Table 1.
Tensile stress at break (MPa) (min-max) | Sharp deflection point (°C) (min-max) | |
Fresh bovine pericardium | 13.2±6.1 (3.4–27.9), n=60 | 69.4±1.5 (66.3–71.3), n=15 |
(1) GAO | 16.1±6.3 (7.8–34.7), n=50 | 86.8±1.1 (86.3–88.8), n=5 |
(2) GAU | 16.9±2.5 (12.9–21.2), n=10 | 81.3±1.8 (78.8–83.8), n=5 |
(3) GA-OL-U | 17.0±6.4 (9.1–25.6), n=10 | 83.8±0, n=5 |
(4) GA-DIOL-U | 18.5±12.5 (4.6–30.7), n=10 | 83.3±1.1 (81.3–83.8), n=5 |
*ANOVA of (1), (2), (3), and (4) | P=0.786 | P=0.000 |
(5) GAG | 18.0±14.9 (3.8–35.5), n=10 | 84.8±1.4 (83.8–86.3), n=5 |
(6) GA-OL-G | 15.1±4.8 (11.0–25.3), n=10 | 85.3±1.4 (83.8–86.3), n=5 |
(7) GA-DIOL-G | 16.5±9.5 (6.3–32.1), n=10 | 86.3±0, n=5 |
*ANOVA of (1), (5), (6), and (7) | P=0.873 | P=0.046 |
Tensile stress at break (MPa) (min-max) | Sharp deflection point (°C) (min-max) | |
Fresh bovine pericardium | 13.2±6.1 (3.4–27.9), n=60 | 69.4±1.5 (66.3–71.3), n=15 |
(1) GAO | 16.1±6.3 (7.8–34.7), n=50 | 86.8±1.1 (86.3–88.8), n=5 |
(2) GAU | 16.9±2.5 (12.9–21.2), n=10 | 81.3±1.8 (78.8–83.8), n=5 |
(3) GA-OL-U | 17.0±6.4 (9.1–25.6), n=10 | 83.8±0, n=5 |
(4) GA-DIOL-U | 18.5±12.5 (4.6–30.7), n=10 | 83.3±1.1 (81.3–83.8), n=5 |
*ANOVA of (1), (2), (3), and (4) | P=0.786 | P=0.000 |
(5) GAG | 18.0±14.9 (3.8–35.5), n=10 | 84.8±1.4 (83.8–86.3), n=5 |
(6) GA-OL-G | 15.1±4.8 (11.0–25.3), n=10 | 85.3±1.4 (83.8–86.3), n=5 |
(7) GA-DIOL-G | 16.5±9.5 (6.3–32.1), n=10 | 86.3±0, n=5 |
*ANOVA of (1), (5), (6), and (7) | P=0.873 | P=0.046 |
When four groups related to urazole were compared (GAO, GAU, GA-OL-U, and GA-DIOL-U), there was no significant difference in tensile strength (MPa). Similarly, the GAO, GAG, GA-OL-G, and GA-DIOL-G groups were compared; there was no significant difference in tensile strength. The results of the thermal stability test are summarized in Table 3. Comparing the GAO, GAU, GA-OL-U, and GA-DIOL-U groups, the temperature points of acute shrinkage revealed some statistically significant differences. The GAU group showed the lowest sharp deflection point (mean, 81.3 °C), and the GA-OL-U and GA-DIOL-U groups were in the middle (mean, 83.3 °C for GA-OL-U and 83.8 °C for GA-DIOL-U), and the GAO group showed the highest sharp deflection point (mean, 86.8 °C). The difference was statistically significant. All of the temperature points of acute deflection are not assumed to exist under in vivo circumstances. Thus, we reasoned that these differences in sharp deflection points had no clinical significance. In the same manner, the GAO, GAG, GA-OL-G, and GA-DIOL-G groups were compared. A post-hoc test (Tukey b test) showed no significant differences among the values of the sharp deflection points. ANOVA, analysis of variance; acronyms for group names were described in Table 1.
Concentrations of calcium and inorganic phosphorus in the tissue; presented with post-hoc test results (Tukey b test). Unit: μgmg (min-max)
Group | n | Subgroups by significance level of 0.05 | ||
1 | 2 | 3 | ||
Calcium | ||||
GAO | 5 | 277.85±17.51 (261.86–304.70) | ||
GA-OL-G | 6 | 0.05±0.04 (0.00–0.10) | ||
GA-OL-U | 6 | 0.18±0.23 (0.04–0.65) | ||
GA-DIOL-G | 6 | 0.35±0.61 (0.00–1.59) | ||
GA-DIOL-U | 6 | 5.24±7.97 (0.05–17.09) | 5.24±7.97 (0.05–17.09) | |
GAG | 6 | 7.95±1.21 (6.44–9.60) | 7.95±1.21 (6.44–9.60) | |
GAU | 6 | 11.86±2.85 (7.72–15.40) | ||
Inorganic phosphorus | ||||
GAO | 5 | 147.07±8.32 (135.31–157.21) | ||
GA-OL-G | 6 | 3.36±0.83 (2.08–4.27) | ||
GA-DIOL-G | 6 | 3.58±0.63 (2.68–4.27) | ||
GA-OL-U | 6 | 3.63±0.61 (2.97–4.44) | ||
GA-DIOL-U | 6 | 14.32±16.95 (2.74–37.33) | 14.32±16.95 (2.74–37.33) | |
GAG | 6 | 21.76±3.48 (17.21–26.32) | 21.76±3.48 (17.21–26.32) | |
GAU | 6 | 32.59±7.73 (21.55–42.26) |
Group | n | Subgroups by significance level of 0.05 | ||
1 | 2 | 3 | ||
Calcium | ||||
GAO | 5 | 277.85±17.51 (261.86–304.70) | ||
GA-OL-G | 6 | 0.05±0.04 (0.00–0.10) | ||
GA-OL-U | 6 | 0.18±0.23 (0.04–0.65) | ||
GA-DIOL-G | 6 | 0.35±0.61 (0.00–1.59) | ||
GA-DIOL-U | 6 | 5.24±7.97 (0.05–17.09) | 5.24±7.97 (0.05–17.09) | |
GAG | 6 | 7.95±1.21 (6.44–9.60) | 7.95±1.21 (6.44–9.60) | |
GAU | 6 | 11.86±2.85 (7.72–15.40) | ||
Inorganic phosphorus | ||||
GAO | 5 | 147.07±8.32 (135.31–157.21) | ||
GA-OL-G | 6 | 3.36±0.83 (2.08–4.27) | ||
GA-DIOL-G | 6 | 3.58±0.63 (2.68–4.27) | ||
GA-OL-U | 6 | 3.63±0.61 (2.97–4.44) | ||
GA-DIOL-U | 6 | 14.32±16.95 (2.74–37.33) | 14.32±16.95 (2.74–37.33) | |
GAG | 6 | 21.76±3.48 (17.21–26.32) | 21.76±3.48 (17.21–26.32) | |
GAU | 6 | 32.59±7.73 (21.55–42.26) |
The Ca2+ concentration of the GAO group was much higher than the others; it was statistically significant. The GAU or GAG group clearly showed a reduced Ca2+ concentration. In the GA-OL-U, GA-DIOL-U, GA-OL-G, and GA-DIOL-G groups, the Ca2+ concentrations were even lower. In the GAO group, the mean IP concentration was 147.07±8.32 μg/mg. When comparing the GAO, GAU, GA-OL-U, GA-DIOL-U groups, the GAO group had the highest IP concentration the GAU group had the second highest IP concentration, and the GA-OL-U, and GA-DIOL-U groups had the lowest concentrations. Similarly, the IP concentration was definitely higher in the GAO group when compared to the GAG, GA-OL-G, and GA-DIOL-G groups. The GAG group had the second lowest concentration, and the GA-OL-G and GA-DIOL-G groups had the lowest concentrations. Acronyms for group names were described in Table 1. IP, inorganic phosphorus.
Concentrations of calcium and inorganic phosphorus in the tissue; presented with post-hoc test results (Tukey b test). Unit: μgmg (min-max)
Group | n | Subgroups by significance level of 0.05 | ||
1 | 2 | 3 | ||
Calcium | ||||
GAO | 5 | 277.85±17.51 (261.86–304.70) | ||
GA-OL-G | 6 | 0.05±0.04 (0.00–0.10) | ||
GA-OL-U | 6 | 0.18±0.23 (0.04–0.65) | ||
GA-DIOL-G | 6 | 0.35±0.61 (0.00–1.59) | ||
GA-DIOL-U | 6 | 5.24±7.97 (0.05–17.09) | 5.24±7.97 (0.05–17.09) | |
GAG | 6 | 7.95±1.21 (6.44–9.60) | 7.95±1.21 (6.44–9.60) | |
GAU | 6 | 11.86±2.85 (7.72–15.40) | ||
Inorganic phosphorus | ||||
GAO | 5 | 147.07±8.32 (135.31–157.21) | ||
GA-OL-G | 6 | 3.36±0.83 (2.08–4.27) | ||
GA-DIOL-G | 6 | 3.58±0.63 (2.68–4.27) | ||
GA-OL-U | 6 | 3.63±0.61 (2.97–4.44) | ||
GA-DIOL-U | 6 | 14.32±16.95 (2.74–37.33) | 14.32±16.95 (2.74–37.33) | |
GAG | 6 | 21.76±3.48 (17.21–26.32) | 21.76±3.48 (17.21–26.32) | |
GAU | 6 | 32.59±7.73 (21.55–42.26) |
Group | n | Subgroups by significance level of 0.05 | ||
1 | 2 | 3 | ||
Calcium | ||||
GAO | 5 | 277.85±17.51 (261.86–304.70) | ||
GA-OL-G | 6 | 0.05±0.04 (0.00–0.10) | ||
GA-OL-U | 6 | 0.18±0.23 (0.04–0.65) | ||
GA-DIOL-G | 6 | 0.35±0.61 (0.00–1.59) | ||
GA-DIOL-U | 6 | 5.24±7.97 (0.05–17.09) | 5.24±7.97 (0.05–17.09) | |
GAG | 6 | 7.95±1.21 (6.44–9.60) | 7.95±1.21 (6.44–9.60) | |
GAU | 6 | 11.86±2.85 (7.72–15.40) | ||
Inorganic phosphorus | ||||
GAO | 5 | 147.07±8.32 (135.31–157.21) | ||
GA-OL-G | 6 | 3.36±0.83 (2.08–4.27) | ||
GA-DIOL-G | 6 | 3.58±0.63 (2.68–4.27) | ||
GA-OL-U | 6 | 3.63±0.61 (2.97–4.44) | ||
GA-DIOL-U | 6 | 14.32±16.95 (2.74–37.33) | 14.32±16.95 (2.74–37.33) | |
GAG | 6 | 21.76±3.48 (17.21–26.32) | 21.76±3.48 (17.21–26.32) | |
GAU | 6 | 32.59±7.73 (21.55–42.26) |
The Ca2+ concentration of the GAO group was much higher than the others; it was statistically significant. The GAU or GAG group clearly showed a reduced Ca2+ concentration. In the GA-OL-U, GA-DIOL-U, GA-OL-G, and GA-DIOL-G groups, the Ca2+ concentrations were even lower. In the GAO group, the mean IP concentration was 147.07±8.32 μg/mg. When comparing the GAO, GAU, GA-OL-U, GA-DIOL-U groups, the GAO group had the highest IP concentration the GAU group had the second highest IP concentration, and the GA-OL-U, and GA-DIOL-U groups had the lowest concentrations. Similarly, the IP concentration was definitely higher in the GAO group when compared to the GAG, GA-OL-G, and GA-DIOL-G groups. The GAG group had the second lowest concentration, and the GA-OL-G and GA-DIOL-G groups had the lowest concentrations. Acronyms for group names were described in Table 1. IP, inorganic phosphorus.
The microscopic images are presented in Fig. 1 . The GAO group had very heavy calcifications, as expected. Most notably, we could see substantial calcifications, even in the GAU and GAG groups. Using urazole or glutamate as an anti-calcification treatment, the concentrations were dramatically reduced from 1/50 to 1/15 in the GAU and GAG groups compared to the GAO group. Nevertheless, under microscopy the tissues were still substantially calcified and the effect of anti-calcification treatment was at least questionable or even unsatisfactory. But, in contrast, in the GA-OL-U, GA-DIOL-U, GA-OL-G, and GA-DIOL-G groups, the calcifications were difficult to detect under microscopy. The tissue structure and density had no detectable inferiority after anti-calcification treatment.
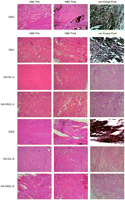
Microscopic images before implantation and after harvest (×40). The GAO group had very heavy calcifications, as expected. Most notably, we could see substantial calcifications, even in the GAU and GAG groups even if the calcium concentrations were dramatically reduced from 1/50 to 1/15 in the GAU and GAG groups compared to the GAO group. H&E, hematoxylin and eosin staining; von Kossa, von Kossa staining; Pre, before implantation; Post, after harvest; acronyms for group names were described in Table 1.
We also compared six anti-calcification groups (GAU, GAG, GA-OL-U, GA-DIOL-U, GA-OL-G, and GA-DIOL-G) and the post-hoc test (Tukey b test) showed distinctly higher calcium concentrations in the GAU group, and distinctly lower calcium concentrations in the GA-DIOL-G group with statistical significance (Table 3). For the IP concentration, the GAU group ranked the highest, and the GA-OL-G group ranked the lowest.
4. Discussion
Free aldehyde molecules which did not take part in cross-linking easily combine with serum Ca2+, and thus the bovine pericardial tissue fixed only with GA is far more susceptible to calcification when implanted in an animal [7]. One of the most well-known methods for removing free aldehyde molecules is detoxification with some organic molecules, such as amino acids. Amino groups in the organic molecule are assumed to combine with residual free aldehyde molecules in the pericardial tissue, and prevent in vivo calcification. Several organic molecules are promising candidates.
In addition, phospholipid is known to play an important role in bovine pericardium in in vivo calcification. The Ca2+ molecules in extracellular fluid are assumed to combine with phosphorus molecules in the phospholipid, which is abundant in dead pericardial cell membranes and forms calcium phosphate crystals [3, 8]. This means that the phospholipid material can be a nidus for calcification.
Treatment with ethanol, butanol, and methanol, or long chain alcohols, such as octanol and octanediol, are known to reduce the amount of phospholipid content in the tissues, and prevent calcium phosphate nucleation, decrease the size and number of collagen, and also interfere with the GA-calcium combination. Furthermore, longer chain alcohols have a more identical structure with phospholipids and they are known to replace phospholipid molecules, thus removing phospholid molecules more effectively [9].
In the experiments presented herein, we attempted to combine both anti-calcification mechanisms (removal of the free aldehyde group and phospholipid content) together to maximize the anti-calcification effect in vivo. Amino-compound treatment alone resulted in a dramatic decrease in the Ca2+ and IP concentration, but the combination of organic solvents and amino compounds showed even less concentration. Although the amino compound only treatment showed substantial amount of microscopic calcification in the tissue, combination treatment resulted in a scant amount of microscopic calcification.
In conclusion, our anti-calcification treatment methods with glutamate, urazole, and solvent treatment showed noticeable effects in the prevention of in vivo calcification without any deterioration of physical properties. Further studies for complementary anti-calcification treatment methods using different mechanisms are necessary in the future to achieve the best anti-calcification effect and to develop bioprosthetic materials with ultra-durability.
Funding: This study was supported by a grant of the Korea Health 21 Research and Development Project, Ministry for Health, Welfare, and Family Affairs, Republic of Korea (Project no.: A040004-006).