-
PDF
- Split View
-
Views
-
Cite
Cite
Thomas M Clarke, Sasha K Whitmarsh, Curtis Champion, Hugh Pederson, Lauren Meyer, Joshua D Dennis, Ross G Dwyer, Charlie Huveneers, Influence of shark tourism on the activity and physiological condition of a non-focal pelagic fish, ICES Journal of Marine Science, Volume 80, Issue 6, August 2023, Pages 1670–1682, https://doi.org/10.1093/icesjms/fsad093
- Share Icon Share
Abstract
Wildlife tourism can have adverse effects on the behaviours and movements of animals, with implications for the health and fitness of individuals and populations. We used acoustic-tracking to show that food-based attractants used in shark-tourism increases activity (15%) and burst behaviours (60%) in yellowtail kingfish (Seriola lalandi, n = 18). Increased activity was restricted to periods when kingfish were on the same side of the island group as berleying tourism vessels, but decreased after operators left the site. Despite the raised activity and frequency of burst swimming events, the physiological condition of kingfish (n = 39, 6 tagged, and 33 untagged) measured using bioelectrical impedance analysis remained consistent with kingfish from control sites not exposed to tourism. This suggests that kingfish were able to compensate raised energy expenditure by feeding on bait and berley used by operators or through natural foraging. We highlight that the effects of provisioning from wildlife tourism can extend beyond changes in behaviours and movements and can additionally influence the energetic condition of non-focal animals through increased activity. However, supplemental food-sources provided through wildlife tourism may be sufficient to compensate for the increased energy expenditure and lessen the effects of tourism on individual fitness and health.
Introduction
Wildlife tourism is a form of human-animal interaction that can lead to behavioural (Laroche et al., 2007; Clua et al., 2010; Heinrich et al., 2021) and physiological (Barnett et al., 2016; Huveneers et al., 2018) effects on both focal (i.e. targeted) and non-focal species at tourism sites. The use of food-based attractant is often required during these operations to aggregate focal species, which are often sparsely distributed in low densities, and undertake large-scale movements, which otherwise leads to challenges to ensure reliable and consistent interactions for tourists (Gallagher & Huveneers, 2018). When provisioning occurs in lesser quantities and at unpredictable times and places, feeding from wildlife tourism can be an efficient technique for tourism operators to increase the frequency of up-close encounters for tourists (Meyer et al., 2021b). However, frequent use of food-based provisioning at tourism sites can have a multitude of detrimental effects on species occupying these areas. For example, direct feeding of nocturnal species can invert diel behaviours, such as southern stingrays Hypanus americanus at Stingray City Sandbar in the Cayman Islands (Corcoran et al., 2013). Similarly, habituation towards supplemental food sources can restrict space use of some marine species due to increased time spent within the vicinity of operators (Huveneers et al., 2013; Rizzari et al., 2017).
Assessment of species movement and residency patterns alone is insufficient to comprehensively assess the impacts of provisioning tourism operations on the health and fitness of animals (Semeniuk et al., 2009; Burgin & Hardiman, 2015; Barnett et al., 2016). Food-based provisioning has also been shown to alter the energetic responses and physiological condition of individuals at tourism sites (Semeniuk et al., 2009). Frequent (i.e. near-daily) supply of supplemental food can increase activity and frequency of burst events associated with pursuing baits and fragmented particles in the water column (Barnett et al., 2016; Huveneers et al., 2018), reflecting changes in metabolic efficacy that ultimately influence critical biological and ecological processes (Brown et al., 2004). Additionally, food used by tourism operators is also often different to naturally occurring prey items, particularly for non-focal species, which are indirectly fed (Meyer et al., 2020), and is often consumed in much higher quantities than regular prey due to ease of access leading to high parasite loads (Semeniuk et al., 2009; Brookhouse et al., 2013), raised stress hormones (Semeniuk et al., 2009), and excessive fat deposits (Brookhouse et al., 2013). While few studies have delved into the energetic and physiological responses of sharks (Barnett et al., 2016; Huveneers et al., 2018) and rays (Semeniuk et al., 2009), the effect of food-based wildlife tourism operations on the energetic responses and physiology of highly mobile large pelagic fish has rarely been quantified (but see Brookhouse et al., 2013).
Knowledge of the activity of free-ranging organisms is essential to understand species’ behavioural ecology and examine how energy expenditure is influenced by abiotic factors (Brown et al., 2004; Payne et al., 2011). The energetic cost associated with different activities can influence growth, reproductive success, and ultimately survival (Brown et al., 2004), and subsequently provides vital information for management and the understanding of ecological processes (Brown et al., 2004; Cooke et al., 2004). Identifying drivers of activity from free-ranging animals therefore contributes to determining energetic expenditure and assessments of how environmental changes or anthropogenic pressures influence animal behaviour at a functional level (Payne et al., 2010). Body condition indices are also broadly used to assess the nutritional and physiological status of fishes (Bolger & Connolly, 1989; Stevenson & Woods, 2006; Brosset et al., 2015) and are often indicative of growth (Critchell & Hoogenboom, 2018), reproductive condition (Trippel, 2003), and mortality (Brosset et al., 2015). Traditional metrics used to measure the body condition of fish are often insensitive (i.e. length-weight relationships; (i.e. length weight relationships; Fulton's K; Nash et al., 2006)), lethal and time-consuming (i.e. hepatosomatic and gonadosomatic indices (Tierney et al., 1996; Lenhardt et al., 2009)), or restricted to laboratory procedures that measure specific physiological parameters (i.e. energy density (Hartman & Brandt, 1995; Johnson et al., 2017)). More recently, sub-lethal methods of measuring body condition of fishes in situ have been established. One such method is bioelectrical impedance analysis (BIA) (Cox & Heintz, 2009), which relies on measures of the resistance and reactance of biological tissue to a weak electrical current. BIA is a rapid assessment method (measurements can be taken in ∼5 seconds) that can be applied in the field to quantify the body condition of live individuals. This metric has been applied to detect differences in body condition of fish at different stages (e.g. fed vs. fasted, and post-mortem; Cox & Heintz, 2009; Champion et al., 2020a) and to assess for changes in response to environmental conditions (Cox & Heintz, 2009) and oceanographic habitat quality (Champion et al., 2020b). Body condition may therefore be used to reveal long-term changes in physiological status due to changes in activity budgets and energy expenditure (Willis & Hobday, 2008), and changed diets due to provisioned food sources.
The white shark cage-diving industry at the Neptune Islands, South Australia, provides an opportunity to assess energetic and physiological responses to provisioning practices on non-focal, mobile pelagic fish. Here, two companies use a food-based attractant (berley; mixture of minced southern bluefin tuna Thunnus maccoyii) and tethered baits (T. maccoyii gills and entrails) to attract sharks into the field of view of tourists (Huveneers & Lloyd, 2017; Huveneers et al., 2017). A third company also operates at the islands, and uses acoustic stimuli (music played via underwater speakers) to attract sharks. While sharks are not fed bait intentionally, small particles from bait and berley plumes in the water column are eaten by smaller, non-focal species (e.g. smooth stingrays Bathytoshia brevicaudata, trevally Pseudocaranx spp., horseshoe leatherjacket Meuschenia hippocrepis (Rizzari et al., 2017; Meyer et al., 2020). This indirect feeding has altered the diets of these fishes, with a shift in fatty acid profiles to more closely resemble the T. maccoyii baits rather than naturally-occurring prey sources (Meyer et al., 2020), as well as contributing to changes in local assemblage abundance (Whitmarsh, 2019), and altered daily movements of some mobile species (Rizzari et al., 2017; Clarke et al., 2022). However, it remains undetermined whether these behavioural changes contribute to physiological effects on the energetic balance and physiological condition of non-focal species, as changes in space use and diet do not necessarily lead to a change in the health or fitness of individuals (Gill et al., 2001; Beale & Monaghan, 2004).
Yellowtail kingfish (Seriola lalandi, hereafter referred to as kingfish) are large-bodied coastal-pelagic fish, frequently occurring at the Neptune Islands Group. Kingfish are typically considered to be mobile piscivores, capable of large-scale movements (i.e. up to 1000s of kilometres; Saul & Holdsworth, 1992; Gillanders et al., 2001), but individuals at the Neptune Islands are resident throughout the year (Clarke et al., 2022) and are often observed feeding on the food-based attractant (Meyer et al., 2020). It is possible that the occurrence of kingfish around cage-diving vessels pursuing baits and feeding on bait and berley could translate to an increase in activity as seen in some shark species (Fitzpatrick et al., 2011; Barnett et al., 2016), and that reliance on an unnatural prey source might limit natural foraging or lack nutritional quality, resulting in poorly-conditioned individuals (Meyer et al., 2020). Kingfish therefore provide a model species to assess the potential effects of shark tourism on the activity and physiological status of a non-focal, large pelagic fish. This study aimed to identify if cage-diving tourism influences the activity and physiological status of kingfish at the Neptune Islands. Specifically, we hypothesised that (a) activity of kingfish increased when food-based cage-diving operators were present at the Neptune Islands, and increased further when kingfish were near tourism vessels, and (b) body condition of kingfish at the Neptune Islands was reduced due to increased activity and diet modification from feeding on an unnatural prey item.
Materials and methods
Site description
This study was undertaken at the North Neptune Islands group (hereafter referred to as Neptune Islands), located ∼30 km from mainland South Australia (35°13.944’S; 135°03.834’E; Figure 1a). An array of 15 VR2-AR acoustic receivers (Innovasea, Nova Scotia, Canada, innovasea.com) was deployed in November 2018 for a 2-year period. Receivers were positioned at least ∼350 m from each other in areas where cage-diving vessels anchor to ensure simultaneous tag detection by 2–3 receivers and enable high-precision estimates of fish position throughout the study period based on range-testing prior to installation. Acoustic receivers also recorded bottom temperature hourly, which was used to calculate the daily mean water temperature.
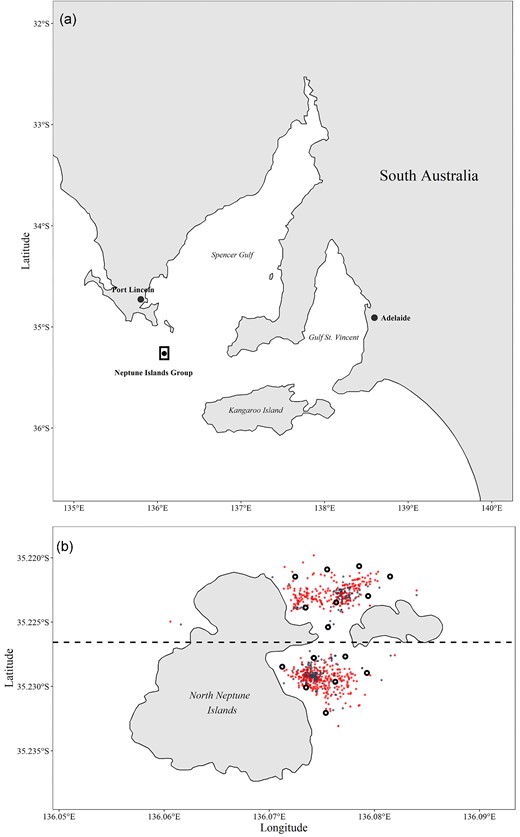
Map of the Neptune Islands showing (a) location within South Australia, and (b) acoustic receiver array detailing V2AR acoustic receivers (black circles), and anchoring locations of food-based (red) and acoustic (blue) cage-diving operators between November 2018 and August 2020.
Capture of kingfish
Between August 2018 and May 2020, 18 mature kingfish (>80 cm total length; Gillanders et al., 1999) were caught via hook and line at the Neptune Islands, and tagged with V16A 98 × 16 mm (17.3 g; 1090 days battery life) or V13A 48 × 13 mm (6.5 g; 368 days battery life) acoustic activity tags (Innovasea, Nova Scotia, Canada, innovasea.com). Fish were inverted upside down in a padded cradle, maintaining water flow over the gills using a hose inserted in the mouth, inducing a tonic immobility reflex (Wells et al., 2005). A small incision was made, and the acoustic tag was inserted in the peritoneal cavity, before being stitched with absorbable sutures (Monosyn 2–0/3–0, B. Braun, Hessen, Germany). Acoustically tagged individuals were also tagged with an external identification tag (Hallprint Plastic Tipped Dart Tags, hallprint.com) to avoid double-tagging, if recaptured. Acoustic tags were equipped with acceleration sensors, which records measurements of acceleration (hereafter referred to as activity), enabling long-term monitoring of relative activity (i.e. 12.5 Hz twenty seconds every 30–90 seconds for V16A, or 5 Hz for 15 seconds every 70–130 seconds for V13A). Acceleration (± 4.9 m/s2 SE range) was sampled for each transmission cycle and converted to a value by the onboard microprocessor using the root mean square value of all three axes (activity = √[X2 + Y2 + Z2], averaged over time). The static contribution to the overall acceleration (g) was filtered out prior to root mean square calculation. Root mean square acceleration resolution was 0.0191 m/s2 (Payne et al., 2011). Overall residency of kingfish at the Neptune Islands was calculated as days detected at the Neptune Islands array/days at liberty.
Kingfish body condition: BIA
Kingfish body condition was quantified using BIA following the protocols detailed by Cox & Heintz et al. (2009) and Champion et al., (2020a). BIA measures two paths of electrical current, resistance (R) and reactance (Xc). Resistance is a reflection of extracellular material (i.e. fat), which is nonconductive and is indicated by higher measurements of R (Cox & Heintz, 2009; Cox et al., 2011) in poorly conditioned individuals. Reactance is measured as the total volume of healthy cells, indicated by the ability of a substance to hold a charge (Gabriel et al., 1996; Hartman et al., 2015). Measurements of R and Xc were used to derive values of electrical phase angle, which is an index of the metabolic condition of fishes (Willis & Hobday, 2008) and has been previously used to assess the body condition of teleost fish (Willis & Hobday, 2008; Hartman et al., 2015; Champion et al., 2020a), including kingfish through the eastern Australian distribution of this species (Champion et al., 2020b):
Phase angle provides a measurement (in degrees) of the angle between the R and Xc vector components of impedance, ranging from 0° to 90°, where higher values represent good body condition as a result of high readings of Xc which are indicative of a high proportion of intact cell membranes (Foster & Lukaski 1996).
Phase angle measurements were taken from kingfish at the Neptune Islands between August 2018 and July 2021. Three replicate BIA measurements were taken along the dorsal musculature of kingfish on a non-conductive surface using the Seafood Analytics Certified Quality Reader (Certified Quality Foods, Inc., United States of America, certifiedqualityseafoods.com). The mean of replicate measurements (n = 3) per individual was calculated for each kingfish. As body length does not influence phase angle measurements taken for kingfish (Champion et al., 2020a), a direct comparison across individuals of different size classes was not necessary. Although variation in reproductive status has the potential to influence phase angle measurements due to increased lipid and moisture content during spawning periods (Jonsson et al., 1997; Domínguez-Petit et al., 2010), previous research has found no effect from reproductive status on Dolly Varden (Salvelinus malma) sampled along the dorsal musculature (Stolarski et al., 2014). Data were compared with phase angle values sampled for wild kingfish that did not interact with provisioning tourism operators throughout the species’ eastern Australian distribution, where values >28° (i.e. the median of 113 fish sampled) reflects individuals within the population that are in relatively good physiological condition (Champion et al., 2020b).
Kingfish activity in response to cage-diving operations
Coordinates of anchored locations and time of cage-diving operations were collected through a customized daily e-logbook (Nazimi et al., 2018). Boat position was allocated to either north-eastern (latitude ≥35°13.68’S) or south-eastern (latitude ≤35°13.68’S) side of the islands based on daily coordinates (Figure 1b), and activity measurements were then assigned as “same side,” or “different sides” based on the time and location of the detection. To reduce the effect from crepuscular patterns influencing the activity of kingfish outside of operating hours, only detections during the day (i.e. after the end of sunrise, before beginning of sunset) were used by filtering for daytime only using the getSunlightTimes function (package “suncalc” version 0.5.0). Activity measurements were also classified based on whether the detection occurred before (360–1 119 minutes, mean = 597), during (7–1 020 minutes, mean = 373), or after (59–958 minutes, mean = 468) cage-diving operations based on time of operation recorded on the e-logbook. For days when operators did not visit the islands, the average arrival (09: 04) and departure (16: 21) times were used. Activity measurements exceeding 4 m/s2 were identified as “burst events,” as this was the threshold expected to correspond with burst feeding events in kingfish of similar size (Clarke et al., 2021). Daily “burst ratios” were calculated for individuals based on the number of burst events divided by the number of activity measurements for that day.
Statistical models
A series of generalized linear mixed models (GLMMs) were used to test the effects of cage-diving operations on the activity of kingfish at the Neptune Islands. All models were constructed using the lmer function in the “lme4” package (version 1.1.23; Bates et al., 2014) in the R statistical environment (version 4.0.2). The first model tested the effect of six different daily operator types (one food-based, two food-based, acoustic only, acoustic + food-based, all operators, no operators) on kingfish activity, while controlling for temperature and unobserved heterogeneity by including temperature and ID to the model as fixed and random effects (slope + intercept), respectively. Study week (i.e. week since the start of the project, when the first fish was tagged, beginning from 15th August 2018) was also included as a random effect in all models to account for temporal autocorrelation. Absolute temperature values collected from receivers were used instead of temperature anomalies, as sea temperature has previously been linked to kingfish spawning behaviours (Moran et al., 2007; Miller et al., 2011), persistence across oceanographic habitats (Champion et al., 2018), and physiological condition (Champion et al., 2020b). The most appropriate statistical family, transformation, and validity of the model were determined by examining the distribution of the response variable and visual inspection of residuals. A gaussian distribution was chosen based on the normal distribution of activity values. Activity values were log-transformed for models measuring effects from operator type, side of the island, and time of the day.
A second model tested for the effect of food-based vs. no provisioning on tagged kingfish activity. Here, no-operator days and days that only acoustic attractant were present were classes as “no provisioning” and any operator type containing food-based attractant as “provisioning.” This model included a before/after effect (i.e. whether activity measurements were taken before, during, or after operators were present, hereafter referred to as “Time”), a second control/impact effect (i.e. if detection occurred on the same “side” of the island as cage-diving), and an interaction between these three terms representing the before after control impact (BACI) effect. Temperature (receiver temperature,°C) and ID were included with a randomized slope and intercept to account for potential lack of independence in behaviour between fish. Activity values were again log-transformed prior to the analysis.
A third model was used to test the effect of berleying operations on the burst ratio of kingfish during hours of operation. This model included only measurements during cage-diving operations, and a control/impact effect (i.e. if food-based or no-food provisioning was used). Only detections during food-based operations on the same side of the island as cage-diving operations were included as provisioning days to assess burst ratio. Temperature and ID were also included as a random effect with randomized slope and intercept. A square-root transformation were deemed necessary for burst ratio model due to the occurrence of 0 values when no burst events exceeding 4 m/s2 was recorded on a particular day.
A fourth model was used to test the effect of ocean bottom temperature (°C) at the time of sampling and body length (total length, cm) on electrical phase angle of kingfish at the Neptune Islands.
All combinations of factors were run, with the most parsimonious model selected using Akaike's information criterion (Burnham & Anderson, 2002) via the dredge function from package “MuMIn” (version 1.43.17). Variance explained (% contribution to change in effect size) from all model factors (conditional R2; Rc) and from only fixed-factors (marginal R2; Rm) were then estimated by subtracting the variance explained by the null-model from each alternative model. Null models for models i—iii all included random effects of temporal change (study week) and individual variation (ID). The ggpredict function (package “ggeffects” version 1.0.1) was used to estimate marginal means (predicted values) for fixed effects included in top ranked models, with these values used to calculate percentage difference between observed effects. Full models were:
(i) Type: activity ∼ Operator type + Temperature + StudyWeekrand + IDrand
(ii) Interaction: activity ∼ Provisioning: Side: Time + Temperature + StudyWeekrand + IDrand
(iii) Burst ratio: burst ratio ∼ Provisioning + Temperature + IDrand
(iv) Body condition: Phase angle (°) ∼ Temperature + body length
Results
Operator type
Activity measurements were obtained from 243 440 detections at the Neptune Islands acoustic array. Over 95% of detections (232 928 detections) occurred during daylight hours, with 3% at night and <1% for dawn and dusk. Between August 2018 and November 2020, cage-diving operators were present on 62% of days (397 out of a possible 639 days). Activity of kingfish within the array at the Neptune Islands was influenced by operator type (2% variance explained) and mean daily temperature (2%), with both factors included in the top-ranked model and together explained 4% of the model variance (Table S1). Activity was lowest on days when no operators were present (mean predicted activity 0.96 ± 0.04 m/s2, β = −1.3) and on days with only acoustic attractant (0.97 ± 0.04 m/s2; β = 0.01; Figure 2). Food-based operations led to an increase in kingfish activity, with activity higher on days when one (mean predicted activity 1.06 ± 0.04 m/s2, β = 0.1), or two (1.09 ± 0.04 m/s2, β = 0.12) food-based operators were present (Figure 2; Table S2). The activity of kingfish increased with water temperatures with this effect slightly larger than operator type (β = 0.07; Figure 2, Table S2). Activity also varied between individual kingfish, which explained 7% of the model variance.
Provisioning, time, side of the island
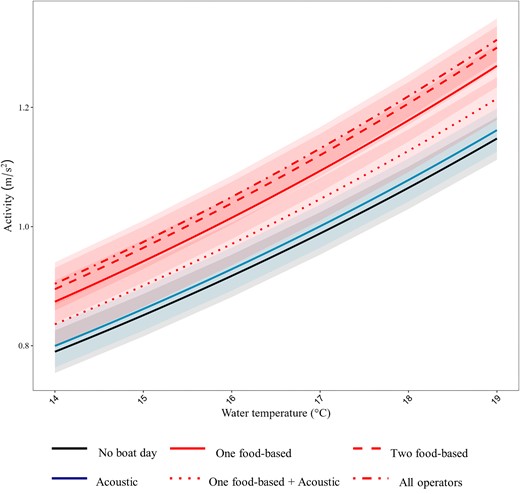
Predicted activity (m/s2; marginal means) of kingfish at the Neptune Islands with different tourism operator types (food-based = red, acoustic attractant = blue, no operator days = black).
The effect of food-based attractant on kingfish activity was influenced by the interactions between provisioning and side of the island (Figure 3a), and time with side of the island (Figure 3b), There was additionally a strong positive relationship of kingfish activity with water temperature (Figure 3c). Interactions explained 4% of the model variation, but there was also high variability between individuals, with random factors explaining 32% of model variation (Table S1). When kingfish were detected on the same side of the island as food-based operators, activity was 15% higher during operations than when non-food-based operators were present (predicted mean activity: 0.89 ± 0.05–1.02 ± 0.05 m/s2; β = 0.1 ± 0.01). There was, however, no effect on kingfish activity when kingfish were on opposing side of the island to the operators, with kingfish similarly active on both operating and non-operating days (0.91 ± 0.05–0.95 ± 0.05 m/s2; Figure 3a). The effect of operators on kingfish activity was also supported by the difference in activity being greatest during tourism operations (12% increase in predicted activity, 0.97 ± 0.05–1.1 ± 0.05 m/s2, β = 0.05 ± 0.01, Figure 3b), with no differences observed prior to the operators arriving (0.1 ± 0.05–0.99 ± 0.06 m/s2; β = -0.09 ± 0.01, Figure 3b, Table S3). After operators left the Neptune Islands kingfish activity decreased, but remained higher on the side of the island that operations occurred, when compared to opposing sides (9.45 ± 0.05–1.02 ± 0.05 m/s2; Figure 3b). Following operators leaving the island, kingfish activity continued to decrease for ∼2 hours until 18: 00, before stabilising and increasing into the evening (Figure S1). Water temperature had the largest influence on kingfish activity of all factors, with a 42% increase in predicted activity as temperatures increased from 14°C–19°C (0.79 ± 0.045–1.12 ± 0.045 m/s2, β = 0.07 ± 0.001).
Burst ratio
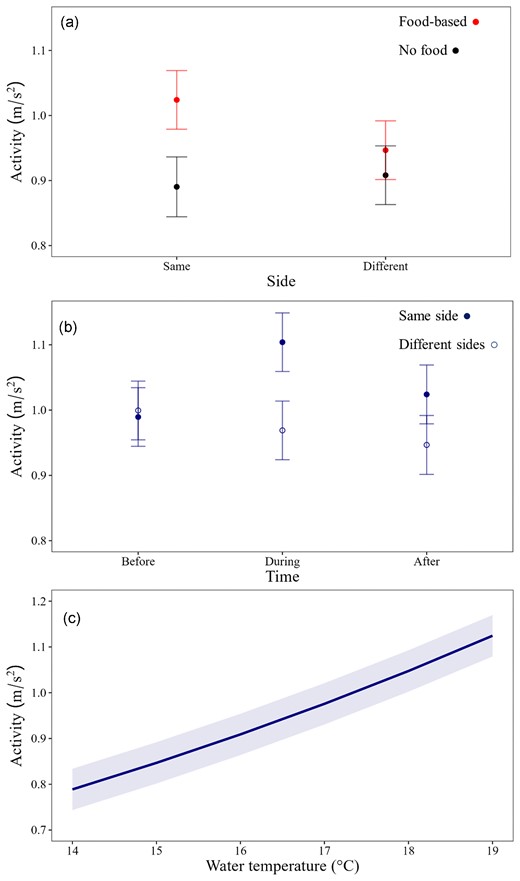
Predicted activity (m/s2; marginal means) of kingfish at the Neptune Islands showing interactions between (a) provisioning and side of the island, (b) time of day and side of the island, and (c) water temperature.
Across the sampling period, 3 366 burst events exceeding 4 m/s2 were detected, ranging between 1 and 26 events per day by individual kingfish. Burst ratio (number of burst/number of detections) for each day ranged from 0 to 0.5 (mean = 0.02 ± 0.001). Food-based operations (3% of variance) and temperature (2% of variance) affected the burst ratio, however, there was also a large amount of variation in burst events amongst individuals (14.1% of the variance by individual ID; Table S1). There was a significant increase in burst ratio on days that food-based operators were present at the Neptune Islands (t-test; t = −2.629, p = <0.01). The ratio of burst behaviours increased by ∼60% with the presence of food-based operators (predicted mean burst ratio 0.003 ± 0.01 vs. 0.005 ± 0.01, β = 0.2 ± 0.004, Figure 4; Table S4). Water temperature had a strong positive relationship with burst ratio (β = 0.01 ± 0.001; Table S4), with burst ratio increasing with temperature for both operating and non-operating days at the Neptune Islands.
Kingfish body condition
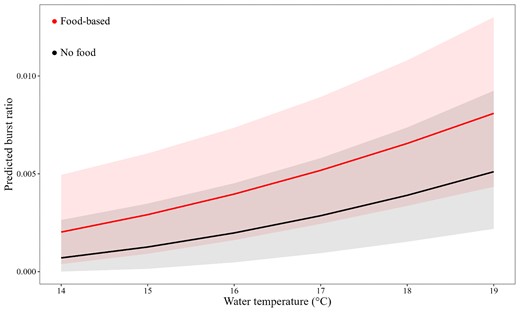
Predicted burst ratio (events exceeding 4 m/s2/total daily detections) of kingfish.
All acoustically-tagged kingfish showed high levels of residency at the Neptune Islands throughout the sampling period (0.42–0.69 residency index [days detected/days at liberty], mean = 0.55 ± 0.04; Figure5a), with individuals being detected at all acoustic receivers. Body condition was calculated from 39 kingfish caught at the Neptune Islands. Kingfish ranged between 52 and 165.5 cm total length (mean = 73.92 cm ± 4.1 SE) and were sampled from water temperatures ranging between 16°C and 19.8°C. Phase angle values ranged from 26.17° to 35.83° (mean = 30.2 ± 0.38°, Figure 5b) and were not influenced by temperature at the time of sampling or body length, with the top-ranked model not including any fixed effects (wAICc = 0.53). Of the 39 fish sampled, 77% (including three acoustically-tagged individuals) had phase angle values similar to wild kingfish determined to be in good body condition (i.e. >28° as per Champion et al., 2020a). There was no significant difference in the body condition of kingfish exposed to provisioning at the Neptune Islands, when compared to control sites (t-test values; t = 1.8786, p = 0.0631).
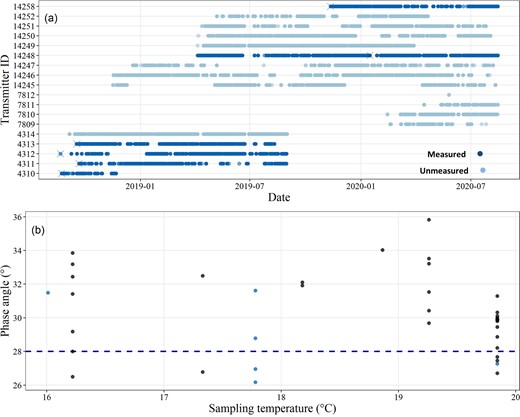
(a) Residency of acoustically-tagged kingfish at the Neptune Islands, with individuals sampled using BIA. Crosses represent dates on which body condition measurements were taken. (b) Body condition (electrical phase angle) of kingfish sampled at the Neptune Islands during food-based provisioning operations. Blue circles represent acoustically-tagged individuals, black circles are untagged kingfish. The horizontal dashed line (y = 28°) represents the value above which kingfish are considered in good body condition (Champion et al., 2020b).
Discussion
This study revealed that the use of bait and berley during white shark cage-diving increased the activity and frequency of burst-swimming events of kingfish, a non-focal pelagic fish species that is often associated with shark tourism vessels. Activity of tagged kingfish increased on days when food-based operators were present, but not when sound was the only type of attractant used. Activity only increased when fish were on the same side of the island as operators using food-based attractant, suggesting that the effects from these operators were restricted to within ∼500 m to vessels. Activity of kingfish also fluctuated throughout the day, being at their highest during berleying activities when operators were on the same side of the island. In comparison, activity remained consistent and lower throughout the day when operators using food products were not present, or when they were on opposite sides of the island to the kingfish at the time of the detection. However, the observed activity increase was not associated with a reduction in electrical phase angle in the tissues of individuals sampled from the same area, suggesting that the behavioural changes in response to cage-diving tourism does not translate to reduced metabolic condition.
Kingfish activity was 15% higher during operations on days when food-based operators were at the Neptune Islands compared to days when these operators were not present. In comparison, no change was observed when the acoustic operator was present, suggesting that the increased activity is associated with the use of bait and berley rather than the mere presence of the cage-diving vessels. Changes in activity reflect a raised energetic expenditure linked to shifts in metabolic rate (Wilson et al., 2006; Gleiss et al., 2011; Lear et al., 2017). Raised activity cost during cage-diving operations might raise kingfish energy expenditure above that incurred during naturally occurring behaviours, which can lead to ongoing effects on growth, reproductive health, and ultimately fitness and survival (Videler & Weihs, 1982). Mobile pelagic fish such as kingfish are capable of high-speed burst behaviours to capture fast-swimming prey and avoid predation (Block et al., 1992), but the high frequency of bursts of activity during cage-diving activities (60% increase) might further affect kingfish energetic budgets, as well as increases risk of physical injury though collisions with vessels, cages, or interspecific species (Brookhouse et al., 2013). These high-acceleration behaviours likely occur when freshly tethered baits are thrown in the water, resulting in individuals dashing towards the food-source. Such burst events in pelagic fish require anaerobic effort and can result in decreased blood pH and oxygen uptake for several hours (Jones, 1978; Randall et al., 1987). As a result, increased frequency of burst swimming behaviours has the potential to disrupt essential biological functions that require oxygen (e.g. respiration, feeding, predatory avoidance, reproduction). Additionally, kingfish are highly residential to the Neptune Islands (residency of some individuals reaching 80%, Clarke et al., 2022), with food-based tourism practices further increasing the number of hours spent at the tourism site. These compounding effects could lead to kingfish exhausting anaerobic energy stores, which are required to capture prey items, reproduce, or avoid predators (Payne et al., 2011), and in the long-term limit growth and reproductive success of individuals and populations (Brown et al., 2004).
The increase in kingfish activity when food-based operators were present was limited to occasions when kingfish were detected on the same side of the Neptune Islands as operators. The geological formation of the Islands essentially segregates the north- and south-eastern sides, with a small gap in-between (∼250 m in width). The bait plume and berley particles might therefore be restricted to the side of the Neptune Islands where it is used and disbursed, without being transported to the other side and influencing kingfish there. While one might suggest restricting cage-diving operations to one side of the Neptune Islands to minimize the impact of shark tourism on kingfish, mobile pelagic species like kingfish (Clarke et al., 2022), trevally (J Dennis, unpublished data), and white sharks (C Huveneers, pers obs.) can move between the north- and south-eastern sides of the Neptune Islands and have been observed to interact with vessels on both sides on the same day. Therefore, restricting operators to one side of the Neptune Islands is unlikely to be an effective approach to reduce the impact of the cage-diving industry on kingfish activity. Management efforts should instead focus on temporal restrictions (i.e. daily operating hours, number of days of operation) or limiting bait and berley quantities, both of which are already in effect (DEW, 2016).
Some wildlife tourism ventures strive to combat negative effects from operations by enforcing strict temporal limitations to reduce the degree of interaction of wildlife with companies (Trave et al., 2017). However, behavioural shifts from provisional feeding can additionally extend beyond operating hours, inverting naturally occurring diel activity patterns (Corcoran et al., 2013). Our study suggests that kingfish activity remained for hours after operators have left the islands, regardless of whether a food-based or non-food-based attractant was used. This poses additional difficulties when quantifying effects of wildlife tourism on the health of animals, given that physiological effects can extend beyond temporal periods that fish are interacting with tourism operators.
Although food-based provisioning increased the activity and burst events of kingfish, our study found that these changes did not translate to a decrease in physiological condition. Based on body condition of kingfish in throughout eastern Australia, individuals at the Neptune Islands were of relatively good physiological status (Cox & Heintz, 2009; Champion et al., 2020a) and of similar condition to kingfish from highly suitable environmental habitats (Champion et al., 2020b). Body condition is inherently linked to the composition of healthy cells and permeability of cell membranes, and has previously been used to detect unfavourable environmental conditions (Champion et al., 2020b), predation risk (Hoey & McCormick, 2004), and effects from anthropogenic stressors (Cavraro et al., 2019). Lack of evidence for reduced body condition despite increased activity might be due to the provisioned food source provided by the food-based operators (bait and berley), which is fed upon by kingfish (Meyer et al., 2020) and is of high nutritional value. The T. maccoyii used as bait and berley by cage-diving operators are acquired locally from aquaculture operations in Port Lincoln, less than 50 km from the Neptune Islands. Tuna are fed locally sourced baitfish i.e. sardines Sardinops spp., that are high in natural proteins and oils, and as a result contain high levels of nutritional fatty acids, including omega-3 fatty acids (Gregory et al., 2010; Pethybridge et al., 2015). While not a naturally-occurring prey item for kingfish, this dietary shift to T. maccoyii, therefore, be comparable, if not more nutritious than the common prey items of kingfish (e.g. small pelagic fish, squid, crustaceans; Gomon et al., 2008; Meyer et al., 2020). This subsidized food source may be compensating for increasing the energy cost during and following cage-diving operations (Barnett et al., 2016), reflected by a healthy body condition. The consumption of food-based attractant could in fact be necessary to compensate for the increased energy expenditure during interactions with cage-diving vessels. A similar instance of food-based wildlife tourism influencing activity budgets has been described, whereby the activity and associated metabolic rate of whitetip reef sharks (Triaenodon obesus) increased by 6.37% on feeding days, with sharks required to increase food consumption (e.g. baits) to compensate and maintain constant body mass (Barnett et al., 2016). However, reliance on tuna as a food source may also decrease kingfish dietary diversity, which can influence gut microbiome communities and disease control and resultingly affect health and reproductive output. There may also be other health impacts for kingfish that are not measured through BIA. For example, kingfish fed with bread and aquaculture pellets at a fish-feeding site at Lord Howe Island (NSW, Australia) demonstrated dependency on provisioned food, raised aggression, frequent skin lesions and stomach ulcers, and heightened parasite loading (Brookhouse et al., 2013). Additionally, these individuals had excessive fat deposits, which would be expected to be represented by poorly conditioned fish indicated through low phase angle values. The sensitivity of BIA to detect subtle changes in physical condition of fishes in response to varying food intake and environmental conditions, suggests that this method is suitable for detecting physiological change due to unfavourable dietary sources. Previous research has suggested that sampling along dorsal musculature can mitigate the influence of reproductive status on body condition (Stolarski et al., 2014). However, it may be possible that kingfish sampled at the Neptune Islands were reproductively mature, contributing to high body condition measurement and possibly compensating for any negative effects from interactions with cage-diving operations. Kingfish sampled at the Neptune Islands were on average larger than control sites (mean total length = 74 cm, compared to 58 cm). As female kingfish first begin to mature at 69 cm fork length, it is possible that some kingfish sampled at control sites were reproductively immature, compared to mature fish sampled at the Neptune Islands. While we believe this is unlikely, and strived to combat reproductive effects by sampling along the dorsal musculature, this is a consideration for future studies using BIA techniques. Future research into physiological shifts in response to provisioning wildlife tourism would benefit from more sensitive measurements of physiological changes (e.g. increased stress hormones; Iwama et al., 1998, changes in blood glucose, lactate build up; Barton et al., 2002) to be paired with energetics inferred through activity metrics, but these methods are more intrusive and time-consuming for field-based studies.
Findings from this study identified impacts from white shark cage-diving tourism on the physiological responses of kingfish, highlighting potential implications for daily energy budgets and resulting consequences for the health and fitness. These results reveal that impacts on activity from food-based wildlife tourism can extend beyond focal-species, and additionally have effects on non-focal individuals, which is particularly pervasive for highly mobile and active individuals such as mobile pelagic fish (Meyer et al., 2021a). Future research would benefit from quantifying the calorific value and quantity of bait and berley consumed by kingfish in combination with respirometry data (Brodie et al., 2016) to help provide a clearer estimation of metabolic processes reflected through the energetic status of effected individuals (Brunnschweiler et al., 2018), but these practices are often expensive and logistically difficult to execute on mobile pelagic fish. Such information of these implications is important for the development of adequate management wildlife tourism frameworks which often largely focus only on impacts to focal-species (Higginbottom et al., 2003; Meyer et al., 2021a), with little consideration for highly mobile non-focal animals such as pelagic fish.
Acknowledgement
Data were sourced from Australia’s Integrated Marine Observing System (IMOS) — IMOS is enabled by the National Collaborative Research Infrastructure Strategy (NCRIS). Body Condition measurements were facilitated by Curtis Champion, Alistair Hobday, and CSIRO coasts and Ocean Research team by loaning the BIA tool. We thank crew and staff from white shark cage-diving operators: Rodney Fox Shark Expeditions, Calypso Star Charters, and Adventure Bay Charters who assisted with sample collection.
Data that supports the findings of this study are available from the corresponding author upon reasonable request.
Author contributions
T.C., S.W., and C.H. were involved with the conception and acquisition of field data. C.C., H.P., L.M., J.D., and R.D. substantially contributed to data collection and the analysis and interpretation of the data. All authors were involved in drafting and revising drafts of the manuscript and give final approval for the final version to be published.
Data availability statement
The data underlying this article is currently held under embargo (available 2025) in the Australian Animal Acoustic Telemetry Database (https://animaltracking.aodn.org.au/). Data will be shared on reasonable request to the corresponding author.
Conflict of interest statement
Authors declare no financial or non-financial competing interests. Sampling took place on board cage-diving operator vessels, which were chartered for research activities.
Funding sources
This study was funded by the Holsworth Wildlife Research Endowment (Project Number 3000010613), and the Biology Society of South Australia (2018 Annual Field Grant).
Statement of inclusion
Our study connects researchers from several different institutions and regions to maximize geographical scope of the data. We engaged with stakeholders in the shark tourism industry during project design and execution of field work. All relevant permissions to reproduce third party material have been obtained prior to acceptance.