-
PDF
- Split View
-
Views
-
Cite
Cite
Molly M Kressler, Sasha R X Dall, Richard B Sherley, A framework for studying ecological energy in the contemporary marine environment, ICES Journal of Marine Science, Volume 80, Issue 6, August 2023, Pages 1580–1593, https://doi.org/10.1093/icesjms/fsad082
- Share Icon Share
Abstract
Historic limitations have resulted in marine ecological studies usually overlooking some well-established concepts from behavioural ecology. This is likely because the methods available were easily overwhelmed by the scale of ecological processes and sampling resolution in the marine environment. Innovations in technology, data management, and statistical modelling now provide the capacity to fully embrace behavioural ecology concepts and study marine ecological interactions from a more holistic perspective. To facilitate this vision, we propose a novel perspective and workflow for marine ecology: the Seascape of Ecological Energy, or SEE-scapes. SEE-scapes contextualizes the accumulated knowledge from marine biology and behavioural ecology research and provides a guide for marine scientists interested in grounding their research in behavioural ecology’s first principles. SEE-scapes specifies relevant considerations for contemporary seascapes, with special attention to relationships between individuals and their conspecifics, competitors, predators, and the abiotic environment. It is formulated to account for how the unique features of marine vertebrates require revisions to standard, terrestrially focused methodological approaches, and offers solutions for how to sample and model the eco-evolutionary drivers of behaviour across the dynamic and hierarchical seascape. Simply put, SEE-scapes provides the guidance to translate a dynamic system and reveal opportunities for collaborative and integrative research.
Introduction
Behavioural ecology—the study of animal behaviour in its ecological and evolutionary context—seeks to distil influences of behaviour and identify how evolution drives dynamics and demography from the individual to the community level. Across the decades, numerous hypotheses have been formulated specifying the ways in which biotic and abiotic ecological factors modulate behaviour over space and time. Some prominent examples include the starvation-predation trade-off (McNamara and Houston, 1990; Brodin and Clark, 2007), dynamic energy budgets (Campos-Candela et al., 2019), the landscape of fear (Laundré et al., 2001; Bleicher, 2017), and energy landscapes (Shepard et al., 2013). These paradigms and others have been widely applied in terrestrial, laboratory, and aquatic studies of the eco-evolutionary drivers of behaviour. These studies often encapsulate multiple aspects and hypotheses of how and why ecological conditions result in behaviour, and are able to assess multiple effects because of technological advances in terrestrial and laboratory-based monitoring equipment available since the early 2000s, e.g. RaspPi cameras (Hereward et al., 2021). By incorporating multiple potential drivers of behaviour into the same studies, researchers can capture more of the natural variation of field systems and provide a better understanding of the causes of the behaviour of free-living animals, along with the consequences of behaviours for the survival of individuals, populations, species, communities, or wider landscapes.
The benefits of embedding first principles from behavioural ecology in field studies have long been realized in terrestrial systems, where technology has been capable of high-resolution tracking and measurement of key ecological conditions and factors for many years (e.g. Farine et al., 2015). However, this approach has not been the default for movement ecology research in marine systems, largely because of a lag in technological innovation and a historically inhospitable and inaccessible environment. The marine environment is dynamic and cryptic, and in moving from a landscape to a seascape, there are marked differences in the spatiotemporal patterns of life. The selective pressures vary hourly through changes in the marine environment and across individual lifetimes on incomparable scales to equivalent pressures in other habitats. At a local and daily level, the tidal regimes dictate spatiotemporal habitat availability and quality, risk, and even resource distribution in coastal environments. Beyond the tides, pelagic waters are subject to currents, flux, and flow. Oceanographic currents drive seasonal and decadal patterns of productivity that support biodiversity at apparently featureless locations, e.g. mid-Atlantic eddies, and at locations with contrasting dramatic underwater features, e.g. seamounts (Yesson et al., 2011; Pittman, 2018; Hosegood et al., 2019; Letessier et al., 2019). And the scales at which vertebrates will act out their life histories under such conditions are unique.
These aspects of life in the marine environment contextualize the difficulties faced by researchers trying to study marine vertebrate behaviour and are why, until recently, incorporating the principles of behavioural ecology into studies of marine animal behaviour has been rare. But the unfortunate reality of this is an understanding of marine systems is limited by an exclusion of the natural variance and the ecological interactions that undoubtedly drive behaviour, and a false confidence in our estimates and predictions. While limitations of the past may have prevented this approach, in the age of rapid technological advancement, there is now more opportunity to incorporate behavioural principles at the centre of marine ecology. The beginnings of this kind of approach in marine vertebrates have started to emerge, particularly in seabirds—where research benefits from the duality of their life histories on both land and sea, resulting in ample airtime for transmission or recovery of archived biologger data (e.g. Rattenborg et al., 2016). And others have identified empirical pathways for the inclusion of energetic expenditure calculations in seabirds (e.g. Shepard et al., 2013) and large teleosts (e.g. Nisbet et al., 2012).
While these recent studies are a step in the right direction, they are exceptions to the general trend of marine ecological research. Outlining the general principles and practises of an approach can kickstart new waves of collaborative, integrative, and comparative research—a notable example is the coining of the “Landscape of Fear” (Laundré et al., 2001; Bleicher, 2017) and the decades of productive research investigating the non-consumptive effects of predators on prey (e.g. Smith et al., 2015). We propose that the field of marine movement ecology could benefit from an explicit framework specifying the relevant principles of behavioural ecology and a general set of recommendations based on contemporary and developing methods. In this review, we will define this perspective, aiming to outline the relevant abiotic and biotic drivers of behaviour at the appropriate scales across marine seascapes.
The framework, or perspective, we propose is called Seascapes of Ecological Energy, or SEE-scapes. Developed for the dynamic marine environment, it outlines the known pathways and influences on the ecology of marine species and demonstrates their hierarchical interdependencies as they affect natural processes and animal behaviour (Figure 1 and Table 1). In the process, we also make some recommendations for how to study these ecological drivers of marine vertebrate behaviour in the marine environment from a more holistic, first-principles, and hierarchical perspective. The SEE-scape has two components: the first is a schematic representation of the relevant behavioural ecology principles for marine life in the seascape; the second is a workflow describing how marine ecologists can integrate the concepts captured by the SEE-scape perspective and design research to capture more of the natural variation of their focal systems and provide a more holistic representation of life as a marine vertebrate. In the rest of this review, to provide guidance on how researchers can use the SEE-scapes framework, we will detail how SEE-scapes best reflects the modern understanding of behavioural ecology principles in the marine environment, and we will outline the innovative technology and sampling methodologies, and contemporary analytic approaches that complement the aims of the SEE-scape perspective and workflow.
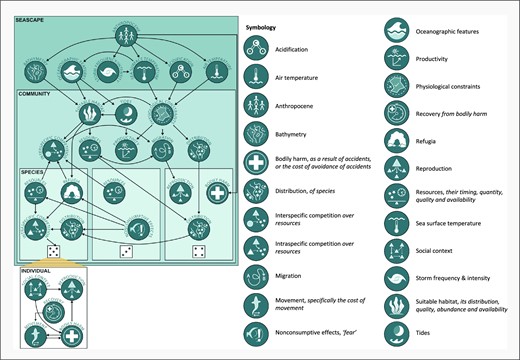
Schematic of the SEE-scapes perspective and symbology key. SEE-scapes is a framework for contextualizing previous and future research from a holistic perspective grounded in behavioural ecology concepts. It attempts to capture the multi-faceted and hierarchical dependencies of the ecological drivers relevant to the lives of a study species, its populations, and its individuals. Ecological drivers are captured by “nodes”, the smaller dark blue circles with iconography. Nodes can be duplicated at the seascape, community, species, or individual levels of the hierarchical SEE-scape and have within- and cross-level impacts on other nodes. These relationships are illustrated by the arrows in the figure. Individual variation within species is represented by the dice faces. The symbology key contains each node and the ecological driver it represents. More information on the relevance of each node in the context of the modern seascape can be found in Table 1.
Evidence of SEE-scape nodes’ relevance in the modern seascape, a brief annotated bibliography for each SEE-scape node.
SEE-scape node | Reference | Annotation |
Acidification | Hoegh-Guldberg et al. (2007) “Coral reefs under rapid climate change and ocean acidification.”and Jarvis et al. (2022) “Elevated CO2 does not alter behavioural lateralization in free‐swimming juvenile European sea bass (Dicentrarchus labrax) tested in groups” | The impacts on behaviour of ocean acidification is a disputed topic in marine ecological literature. There is some evidence to suggest it will have an impact on the physiology and behaviour of marine species (Hoegh-Guldberg et al., 2007); and some evidence that it will not (Jarvis et al., 2022). |
Air temperature | Moline et al. (2004) “Alteration of the food web along the Antarctic Peninsula in response to a regional warming trend” | Changes in the atmospheric temperature will interact with the mechanisms of the seascape, especially in areas where sea ice plays a large role in the community and species ecology, as was found in phytoplankton community of the Antarctic. |
Anthropocene | Arlinghaus et al. (2021) “Niche overlap among anglers, fishers and cormorants and their removals of fish biomass: A case from brackish lagoon ecosystems in the southern Baltic Sea” | In the Baltic Sea, cormorants Phalacrocorax carbo sinensis and commercial fisheries compete for the same resources in the contemporary seascape. |
Bathymetry | Hosegood et al. (2019) “Internal lee waves and baroclinic bores over a tropical seamount shark ‘hot-spot’” | The sub-surface features of the Chagos archipelago produce favourable conditions for large aggregations of sharks in largely featureless pelagic waters. |
Bodily harm and recovery from bodily harm | Votier et al. (2005) “Oil pollution and climate have wide-scale impacts on seabird demographics” | Oil spills in the North Atlantic had additive effects on the mortality of seabirds relative to baseline survival rates. |
Distribution of species | McMahan and Grabowski (2019) “Nonconsumptive effects of a range-expanding predator on juvenile lobster (Homarus americanus) population dynamics” | The historic distribution of species can play a role in the contemporary response of a species to community changes. The history of spatial overlap between lobsters Homarus americanus and sea bass predicted the contemporary space use of juvenile lobsters as climate change drives range expansion in their predator, sea bass Centropristis striata. |
Interspecific competition | Nash et al. (2012) “Influence of habitat condition and competition on foraging behaviour of parrotfishes” | Competition between two species of parrotfish (Scarus spp.) was found to be a significant predictor of individuals space use, specific to foraging, in a coral reef system. |
Intraspecific competition | Wakefield et al. (2013) “Space Partitioning Without Territoriality in Gannets” | Among-species breeding period foraging areas were spatially explicit between 12 colonies of Northern gannets (Morus bassanus) in the UK. A density-dependent modellign approach suggests these foraging home ranges are driven by intra-specific intercolonial competition for pelagic prey. |
Migration | Mather et al. (2013) “What happens in an estuary doesn’t stay there: patterns of biotic connectivity resulting from long term ecological research” | Variation in a single species migration can have cascading effects into and across multiple different systems. |
Nonconsumptive effects | Breed et al. (2017) “Sustained disruption of narwhal habitat use and behaviour in the presence of Arctic killer whales” | The presence of killer whales Orcinus orca resulted in sustained behavioural modification by narwhales in the Admiralty Inlet, Canada. |
Oceanographic features | Miller et al. (2015) “Basking sharks and oceanographic fronts: quantifying associations in the north-east Atlantic” | Spatial and temporal variation in oceanographic frontal zones correlated with basking shark Cetorhinus maximus space use. |
Productivity | Sabal et al. (2020) “California Current seascape influences juvenile salmon foraging ecology at multiple scales” | The foraging ecology of a juvenile salmon Oncorhynchus spp. shifts based on the productivity of the environment and the scale at which researchers examine the focal behaviours and focal phenomena. |
Physiological constraints | Brownscombe et al. (2017) “Ecology of Exercise in Wild Fish: Integrating Concepts of Individual Physiological Capacity, Behaviour, and Fitness Through Diverse Case Studies” | Bonefish Albula vulpis were found to forage selectively in habitats where the abiotic conditions were within their thermal optima. |
Refugia | Kanno et al. (2019) “Stationary video monitoring reveals habitat use of stingrays in mangroves” | Species may use physical features of the seascape to recuperate energy. In Australia, juvenile mangrove whiprays Urogymnus granulatus and cowtail stingrays Pastinachus ater were found to rest in mangrove fringes depending on the tide and season. |
Reproduction | Cassill (2021) “Multiple maternal risk-management adaptations in the loggerhead sea turtle (Caretta caretta) mitigate clutch failure caused by catastrophic storms and predators” | Female loggerhead turtles on the south eastern coast of Florida (USA) were found to adjust their behaviour to try to mitigate the fitness consequences of clutch loss. |
Resources | Santana-Garcon et al. (2014) “Development and validation of a mid-water baited stereo-video technique for investigating pelagic fish assemblages” | A test of pelagic stereo BRUVS was able to identify the spatial and temporal variation in fish assemblages. |
Sea surface temperature (SST) | Freitas et al. (2021) “Sea temperature effects on depth use and habitat selection in a marine fish community” | In Norway, thermal heterogeneity allowed for fish species to adjust their vertical distribution to stay within their thermal optima. |
Social context | Keller et al. (2017) “The effects of familiarity on the social interactions of juvenile lemon sharks, Negaprion brevirostris” | Social network analysis in semi-captive juvenile lemon sharks identified preference for familiar individuals, over unfamiliar individuals. |
Storm frequency and intensity | Sherley et al. (2012) “Storms and heat limit the nest success of Bank Cormorants: Implications of future climate change for a surface-nesting seabird in southern Africa” | Heat waves, air temperature, and storms, were found to influence the nest success of bank cormorants Phalacrocorax neglectus in the Benguela Upwelling System. |
Suitable habitat | Calich et al. (2018) “Overlap between highly suitable habitats and longline gear management areas reveals vulnerable and protected regions for highly migratory sharks” | Suitable habitat was identified for a group of elasmobranchs using maximum entropy models and overlap of this habitat with fisheries gear was assessed. |
Tides | Trevail et al. (2019) “Environmental heterogeneity amplifies behavioural response to a temporal cycle” | Short scale tidal regimes interact with larger scale environmental variables to driver greater variability in resource availability. For black-legged kittiwakes Rissa trdactyla, this results in amplification of behavioural variation across tidal regimes. |
SEE-scape node | Reference | Annotation |
Acidification | Hoegh-Guldberg et al. (2007) “Coral reefs under rapid climate change and ocean acidification.”and Jarvis et al. (2022) “Elevated CO2 does not alter behavioural lateralization in free‐swimming juvenile European sea bass (Dicentrarchus labrax) tested in groups” | The impacts on behaviour of ocean acidification is a disputed topic in marine ecological literature. There is some evidence to suggest it will have an impact on the physiology and behaviour of marine species (Hoegh-Guldberg et al., 2007); and some evidence that it will not (Jarvis et al., 2022). |
Air temperature | Moline et al. (2004) “Alteration of the food web along the Antarctic Peninsula in response to a regional warming trend” | Changes in the atmospheric temperature will interact with the mechanisms of the seascape, especially in areas where sea ice plays a large role in the community and species ecology, as was found in phytoplankton community of the Antarctic. |
Anthropocene | Arlinghaus et al. (2021) “Niche overlap among anglers, fishers and cormorants and their removals of fish biomass: A case from brackish lagoon ecosystems in the southern Baltic Sea” | In the Baltic Sea, cormorants Phalacrocorax carbo sinensis and commercial fisheries compete for the same resources in the contemporary seascape. |
Bathymetry | Hosegood et al. (2019) “Internal lee waves and baroclinic bores over a tropical seamount shark ‘hot-spot’” | The sub-surface features of the Chagos archipelago produce favourable conditions for large aggregations of sharks in largely featureless pelagic waters. |
Bodily harm and recovery from bodily harm | Votier et al. (2005) “Oil pollution and climate have wide-scale impacts on seabird demographics” | Oil spills in the North Atlantic had additive effects on the mortality of seabirds relative to baseline survival rates. |
Distribution of species | McMahan and Grabowski (2019) “Nonconsumptive effects of a range-expanding predator on juvenile lobster (Homarus americanus) population dynamics” | The historic distribution of species can play a role in the contemporary response of a species to community changes. The history of spatial overlap between lobsters Homarus americanus and sea bass predicted the contemporary space use of juvenile lobsters as climate change drives range expansion in their predator, sea bass Centropristis striata. |
Interspecific competition | Nash et al. (2012) “Influence of habitat condition and competition on foraging behaviour of parrotfishes” | Competition between two species of parrotfish (Scarus spp.) was found to be a significant predictor of individuals space use, specific to foraging, in a coral reef system. |
Intraspecific competition | Wakefield et al. (2013) “Space Partitioning Without Territoriality in Gannets” | Among-species breeding period foraging areas were spatially explicit between 12 colonies of Northern gannets (Morus bassanus) in the UK. A density-dependent modellign approach suggests these foraging home ranges are driven by intra-specific intercolonial competition for pelagic prey. |
Migration | Mather et al. (2013) “What happens in an estuary doesn’t stay there: patterns of biotic connectivity resulting from long term ecological research” | Variation in a single species migration can have cascading effects into and across multiple different systems. |
Nonconsumptive effects | Breed et al. (2017) “Sustained disruption of narwhal habitat use and behaviour in the presence of Arctic killer whales” | The presence of killer whales Orcinus orca resulted in sustained behavioural modification by narwhales in the Admiralty Inlet, Canada. |
Oceanographic features | Miller et al. (2015) “Basking sharks and oceanographic fronts: quantifying associations in the north-east Atlantic” | Spatial and temporal variation in oceanographic frontal zones correlated with basking shark Cetorhinus maximus space use. |
Productivity | Sabal et al. (2020) “California Current seascape influences juvenile salmon foraging ecology at multiple scales” | The foraging ecology of a juvenile salmon Oncorhynchus spp. shifts based on the productivity of the environment and the scale at which researchers examine the focal behaviours and focal phenomena. |
Physiological constraints | Brownscombe et al. (2017) “Ecology of Exercise in Wild Fish: Integrating Concepts of Individual Physiological Capacity, Behaviour, and Fitness Through Diverse Case Studies” | Bonefish Albula vulpis were found to forage selectively in habitats where the abiotic conditions were within their thermal optima. |
Refugia | Kanno et al. (2019) “Stationary video monitoring reveals habitat use of stingrays in mangroves” | Species may use physical features of the seascape to recuperate energy. In Australia, juvenile mangrove whiprays Urogymnus granulatus and cowtail stingrays Pastinachus ater were found to rest in mangrove fringes depending on the tide and season. |
Reproduction | Cassill (2021) “Multiple maternal risk-management adaptations in the loggerhead sea turtle (Caretta caretta) mitigate clutch failure caused by catastrophic storms and predators” | Female loggerhead turtles on the south eastern coast of Florida (USA) were found to adjust their behaviour to try to mitigate the fitness consequences of clutch loss. |
Resources | Santana-Garcon et al. (2014) “Development and validation of a mid-water baited stereo-video technique for investigating pelagic fish assemblages” | A test of pelagic stereo BRUVS was able to identify the spatial and temporal variation in fish assemblages. |
Sea surface temperature (SST) | Freitas et al. (2021) “Sea temperature effects on depth use and habitat selection in a marine fish community” | In Norway, thermal heterogeneity allowed for fish species to adjust their vertical distribution to stay within their thermal optima. |
Social context | Keller et al. (2017) “The effects of familiarity on the social interactions of juvenile lemon sharks, Negaprion brevirostris” | Social network analysis in semi-captive juvenile lemon sharks identified preference for familiar individuals, over unfamiliar individuals. |
Storm frequency and intensity | Sherley et al. (2012) “Storms and heat limit the nest success of Bank Cormorants: Implications of future climate change for a surface-nesting seabird in southern Africa” | Heat waves, air temperature, and storms, were found to influence the nest success of bank cormorants Phalacrocorax neglectus in the Benguela Upwelling System. |
Suitable habitat | Calich et al. (2018) “Overlap between highly suitable habitats and longline gear management areas reveals vulnerable and protected regions for highly migratory sharks” | Suitable habitat was identified for a group of elasmobranchs using maximum entropy models and overlap of this habitat with fisheries gear was assessed. |
Tides | Trevail et al. (2019) “Environmental heterogeneity amplifies behavioural response to a temporal cycle” | Short scale tidal regimes interact with larger scale environmental variables to driver greater variability in resource availability. For black-legged kittiwakes Rissa trdactyla, this results in amplification of behavioural variation across tidal regimes. |
In the table, for each node, an exemplar from the literature is provided to illustrate the relevance of and importance of measuring the ecological driver in the context of the contemporary seascape and modern ecological studies.
Evidence of SEE-scape nodes’ relevance in the modern seascape, a brief annotated bibliography for each SEE-scape node.
SEE-scape node | Reference | Annotation |
Acidification | Hoegh-Guldberg et al. (2007) “Coral reefs under rapid climate change and ocean acidification.”and Jarvis et al. (2022) “Elevated CO2 does not alter behavioural lateralization in free‐swimming juvenile European sea bass (Dicentrarchus labrax) tested in groups” | The impacts on behaviour of ocean acidification is a disputed topic in marine ecological literature. There is some evidence to suggest it will have an impact on the physiology and behaviour of marine species (Hoegh-Guldberg et al., 2007); and some evidence that it will not (Jarvis et al., 2022). |
Air temperature | Moline et al. (2004) “Alteration of the food web along the Antarctic Peninsula in response to a regional warming trend” | Changes in the atmospheric temperature will interact with the mechanisms of the seascape, especially in areas where sea ice plays a large role in the community and species ecology, as was found in phytoplankton community of the Antarctic. |
Anthropocene | Arlinghaus et al. (2021) “Niche overlap among anglers, fishers and cormorants and their removals of fish biomass: A case from brackish lagoon ecosystems in the southern Baltic Sea” | In the Baltic Sea, cormorants Phalacrocorax carbo sinensis and commercial fisheries compete for the same resources in the contemporary seascape. |
Bathymetry | Hosegood et al. (2019) “Internal lee waves and baroclinic bores over a tropical seamount shark ‘hot-spot’” | The sub-surface features of the Chagos archipelago produce favourable conditions for large aggregations of sharks in largely featureless pelagic waters. |
Bodily harm and recovery from bodily harm | Votier et al. (2005) “Oil pollution and climate have wide-scale impacts on seabird demographics” | Oil spills in the North Atlantic had additive effects on the mortality of seabirds relative to baseline survival rates. |
Distribution of species | McMahan and Grabowski (2019) “Nonconsumptive effects of a range-expanding predator on juvenile lobster (Homarus americanus) population dynamics” | The historic distribution of species can play a role in the contemporary response of a species to community changes. The history of spatial overlap between lobsters Homarus americanus and sea bass predicted the contemporary space use of juvenile lobsters as climate change drives range expansion in their predator, sea bass Centropristis striata. |
Interspecific competition | Nash et al. (2012) “Influence of habitat condition and competition on foraging behaviour of parrotfishes” | Competition between two species of parrotfish (Scarus spp.) was found to be a significant predictor of individuals space use, specific to foraging, in a coral reef system. |
Intraspecific competition | Wakefield et al. (2013) “Space Partitioning Without Territoriality in Gannets” | Among-species breeding period foraging areas were spatially explicit between 12 colonies of Northern gannets (Morus bassanus) in the UK. A density-dependent modellign approach suggests these foraging home ranges are driven by intra-specific intercolonial competition for pelagic prey. |
Migration | Mather et al. (2013) “What happens in an estuary doesn’t stay there: patterns of biotic connectivity resulting from long term ecological research” | Variation in a single species migration can have cascading effects into and across multiple different systems. |
Nonconsumptive effects | Breed et al. (2017) “Sustained disruption of narwhal habitat use and behaviour in the presence of Arctic killer whales” | The presence of killer whales Orcinus orca resulted in sustained behavioural modification by narwhales in the Admiralty Inlet, Canada. |
Oceanographic features | Miller et al. (2015) “Basking sharks and oceanographic fronts: quantifying associations in the north-east Atlantic” | Spatial and temporal variation in oceanographic frontal zones correlated with basking shark Cetorhinus maximus space use. |
Productivity | Sabal et al. (2020) “California Current seascape influences juvenile salmon foraging ecology at multiple scales” | The foraging ecology of a juvenile salmon Oncorhynchus spp. shifts based on the productivity of the environment and the scale at which researchers examine the focal behaviours and focal phenomena. |
Physiological constraints | Brownscombe et al. (2017) “Ecology of Exercise in Wild Fish: Integrating Concepts of Individual Physiological Capacity, Behaviour, and Fitness Through Diverse Case Studies” | Bonefish Albula vulpis were found to forage selectively in habitats where the abiotic conditions were within their thermal optima. |
Refugia | Kanno et al. (2019) “Stationary video monitoring reveals habitat use of stingrays in mangroves” | Species may use physical features of the seascape to recuperate energy. In Australia, juvenile mangrove whiprays Urogymnus granulatus and cowtail stingrays Pastinachus ater were found to rest in mangrove fringes depending on the tide and season. |
Reproduction | Cassill (2021) “Multiple maternal risk-management adaptations in the loggerhead sea turtle (Caretta caretta) mitigate clutch failure caused by catastrophic storms and predators” | Female loggerhead turtles on the south eastern coast of Florida (USA) were found to adjust their behaviour to try to mitigate the fitness consequences of clutch loss. |
Resources | Santana-Garcon et al. (2014) “Development and validation of a mid-water baited stereo-video technique for investigating pelagic fish assemblages” | A test of pelagic stereo BRUVS was able to identify the spatial and temporal variation in fish assemblages. |
Sea surface temperature (SST) | Freitas et al. (2021) “Sea temperature effects on depth use and habitat selection in a marine fish community” | In Norway, thermal heterogeneity allowed for fish species to adjust their vertical distribution to stay within their thermal optima. |
Social context | Keller et al. (2017) “The effects of familiarity on the social interactions of juvenile lemon sharks, Negaprion brevirostris” | Social network analysis in semi-captive juvenile lemon sharks identified preference for familiar individuals, over unfamiliar individuals. |
Storm frequency and intensity | Sherley et al. (2012) “Storms and heat limit the nest success of Bank Cormorants: Implications of future climate change for a surface-nesting seabird in southern Africa” | Heat waves, air temperature, and storms, were found to influence the nest success of bank cormorants Phalacrocorax neglectus in the Benguela Upwelling System. |
Suitable habitat | Calich et al. (2018) “Overlap between highly suitable habitats and longline gear management areas reveals vulnerable and protected regions for highly migratory sharks” | Suitable habitat was identified for a group of elasmobranchs using maximum entropy models and overlap of this habitat with fisheries gear was assessed. |
Tides | Trevail et al. (2019) “Environmental heterogeneity amplifies behavioural response to a temporal cycle” | Short scale tidal regimes interact with larger scale environmental variables to driver greater variability in resource availability. For black-legged kittiwakes Rissa trdactyla, this results in amplification of behavioural variation across tidal regimes. |
SEE-scape node | Reference | Annotation |
Acidification | Hoegh-Guldberg et al. (2007) “Coral reefs under rapid climate change and ocean acidification.”and Jarvis et al. (2022) “Elevated CO2 does not alter behavioural lateralization in free‐swimming juvenile European sea bass (Dicentrarchus labrax) tested in groups” | The impacts on behaviour of ocean acidification is a disputed topic in marine ecological literature. There is some evidence to suggest it will have an impact on the physiology and behaviour of marine species (Hoegh-Guldberg et al., 2007); and some evidence that it will not (Jarvis et al., 2022). |
Air temperature | Moline et al. (2004) “Alteration of the food web along the Antarctic Peninsula in response to a regional warming trend” | Changes in the atmospheric temperature will interact with the mechanisms of the seascape, especially in areas where sea ice plays a large role in the community and species ecology, as was found in phytoplankton community of the Antarctic. |
Anthropocene | Arlinghaus et al. (2021) “Niche overlap among anglers, fishers and cormorants and their removals of fish biomass: A case from brackish lagoon ecosystems in the southern Baltic Sea” | In the Baltic Sea, cormorants Phalacrocorax carbo sinensis and commercial fisheries compete for the same resources in the contemporary seascape. |
Bathymetry | Hosegood et al. (2019) “Internal lee waves and baroclinic bores over a tropical seamount shark ‘hot-spot’” | The sub-surface features of the Chagos archipelago produce favourable conditions for large aggregations of sharks in largely featureless pelagic waters. |
Bodily harm and recovery from bodily harm | Votier et al. (2005) “Oil pollution and climate have wide-scale impacts on seabird demographics” | Oil spills in the North Atlantic had additive effects on the mortality of seabirds relative to baseline survival rates. |
Distribution of species | McMahan and Grabowski (2019) “Nonconsumptive effects of a range-expanding predator on juvenile lobster (Homarus americanus) population dynamics” | The historic distribution of species can play a role in the contemporary response of a species to community changes. The history of spatial overlap between lobsters Homarus americanus and sea bass predicted the contemporary space use of juvenile lobsters as climate change drives range expansion in their predator, sea bass Centropristis striata. |
Interspecific competition | Nash et al. (2012) “Influence of habitat condition and competition on foraging behaviour of parrotfishes” | Competition between two species of parrotfish (Scarus spp.) was found to be a significant predictor of individuals space use, specific to foraging, in a coral reef system. |
Intraspecific competition | Wakefield et al. (2013) “Space Partitioning Without Territoriality in Gannets” | Among-species breeding period foraging areas were spatially explicit between 12 colonies of Northern gannets (Morus bassanus) in the UK. A density-dependent modellign approach suggests these foraging home ranges are driven by intra-specific intercolonial competition for pelagic prey. |
Migration | Mather et al. (2013) “What happens in an estuary doesn’t stay there: patterns of biotic connectivity resulting from long term ecological research” | Variation in a single species migration can have cascading effects into and across multiple different systems. |
Nonconsumptive effects | Breed et al. (2017) “Sustained disruption of narwhal habitat use and behaviour in the presence of Arctic killer whales” | The presence of killer whales Orcinus orca resulted in sustained behavioural modification by narwhales in the Admiralty Inlet, Canada. |
Oceanographic features | Miller et al. (2015) “Basking sharks and oceanographic fronts: quantifying associations in the north-east Atlantic” | Spatial and temporal variation in oceanographic frontal zones correlated with basking shark Cetorhinus maximus space use. |
Productivity | Sabal et al. (2020) “California Current seascape influences juvenile salmon foraging ecology at multiple scales” | The foraging ecology of a juvenile salmon Oncorhynchus spp. shifts based on the productivity of the environment and the scale at which researchers examine the focal behaviours and focal phenomena. |
Physiological constraints | Brownscombe et al. (2017) “Ecology of Exercise in Wild Fish: Integrating Concepts of Individual Physiological Capacity, Behaviour, and Fitness Through Diverse Case Studies” | Bonefish Albula vulpis were found to forage selectively in habitats where the abiotic conditions were within their thermal optima. |
Refugia | Kanno et al. (2019) “Stationary video monitoring reveals habitat use of stingrays in mangroves” | Species may use physical features of the seascape to recuperate energy. In Australia, juvenile mangrove whiprays Urogymnus granulatus and cowtail stingrays Pastinachus ater were found to rest in mangrove fringes depending on the tide and season. |
Reproduction | Cassill (2021) “Multiple maternal risk-management adaptations in the loggerhead sea turtle (Caretta caretta) mitigate clutch failure caused by catastrophic storms and predators” | Female loggerhead turtles on the south eastern coast of Florida (USA) were found to adjust their behaviour to try to mitigate the fitness consequences of clutch loss. |
Resources | Santana-Garcon et al. (2014) “Development and validation of a mid-water baited stereo-video technique for investigating pelagic fish assemblages” | A test of pelagic stereo BRUVS was able to identify the spatial and temporal variation in fish assemblages. |
Sea surface temperature (SST) | Freitas et al. (2021) “Sea temperature effects on depth use and habitat selection in a marine fish community” | In Norway, thermal heterogeneity allowed for fish species to adjust their vertical distribution to stay within their thermal optima. |
Social context | Keller et al. (2017) “The effects of familiarity on the social interactions of juvenile lemon sharks, Negaprion brevirostris” | Social network analysis in semi-captive juvenile lemon sharks identified preference for familiar individuals, over unfamiliar individuals. |
Storm frequency and intensity | Sherley et al. (2012) “Storms and heat limit the nest success of Bank Cormorants: Implications of future climate change for a surface-nesting seabird in southern Africa” | Heat waves, air temperature, and storms, were found to influence the nest success of bank cormorants Phalacrocorax neglectus in the Benguela Upwelling System. |
Suitable habitat | Calich et al. (2018) “Overlap between highly suitable habitats and longline gear management areas reveals vulnerable and protected regions for highly migratory sharks” | Suitable habitat was identified for a group of elasmobranchs using maximum entropy models and overlap of this habitat with fisheries gear was assessed. |
Tides | Trevail et al. (2019) “Environmental heterogeneity amplifies behavioural response to a temporal cycle” | Short scale tidal regimes interact with larger scale environmental variables to driver greater variability in resource availability. For black-legged kittiwakes Rissa trdactyla, this results in amplification of behavioural variation across tidal regimes. |
In the table, for each node, an exemplar from the literature is provided to illustrate the relevance of and importance of measuring the ecological driver in the context of the contemporary seascape and modern ecological studies.
The seascape of ecological energy (SEE-scapes)
Concept and practicality
The SEE-scapes framework identifies geographically, biologically, and ecologically relevant considerations for a study of behaviour in the contemporary marine environment (Figure 1), embedding behavioural ecology principles based on decades of published research (Table 1). The SEE-scape has been conceptualized as a tool to enable researchers to place their research questions within the context of the dynamic contemporary marine environment and identify, and hopefully gain controlling insight into, the possible interacting ecological drivers that produce so much “noise” in studies of marine vertebrate behaviour (Figures 1 and 2, Table 1). Creating a near-endless feedback loop across the stages of the workflow (Figure 2), SEE-scapes is situated to be a tool for marine researchers to use and revisit again and again, as they work to come to grips with the dynamic ecological processes of their focal species or system. The more noise we can capture and work towards explaining, the clearer the true signal of behaviour and its drivers will become, and so too will the insight and guidance from marine research; to guide these efforts is the goal of SEE-scapes.
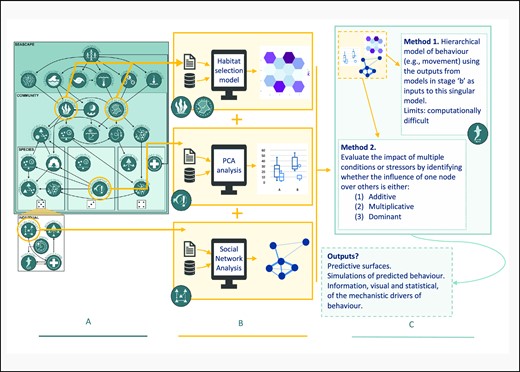
The SEE-scapes workflow: how we envisage the SEE-scapes perspective to be used as a framework for designing and analysing ecological studies in the contemporary marine environment. Each stage (A–C) describes a different stage in the iterative research process. Stage (A) uses the SEE-scapes perspective to illustrate how users should consolidate available knowledge about the mechanistic interactions in the study system and identify nodes that they have or can measure in relation to a focal species behaviour. Stage (B) demonstrates how individual or small groups of nodes can be isolated for studies of a focal behaviour in relation to those nodes. Three examples of how to model behaviour based on measurable aspects of nodes are provided, and possible outputs from these example methods are illustrated. In (B), researchers could consider pruning back inputs to final predictive models for each node, especially if and when it is evident that a node has little to no effect on the focal behaviour. Outputs from (B) are themselves of scientific interest and suitable for publication and dissemination, but they are also used as inputs in stage (C). Stage (C) uses outputs from Stage (B) simultaneously to evaluate the direction and magnitude of each node’s behaviour and/or energetic expenditure. In this stage, researchers can evaluate whether nodes are multiplicative, additive, or dominant and produce predictions based on multiple inputs (models and/or nodes) using hierarchical models or model averaging. Here, two possible methods are described for achieving this outcome in (C): “Method 1” describes a multi-level hierarchical approach, and “Method 2” describes a multi-model model averaging and comparison approach. Stage (C) outputs can be fed back into the start of the SEE-scapes workflow, reinforming the mechanistic perspective of the drivers of behaviour in a given focal system from a more holistic perspective. Fundamental to the SEE-scapes workflow is the propagation of uncertainty and the acknowledgement of the hierarchical dependencies among nodes, and this will produce more realistic descriptions of the signal and noise of wild marine vertebrate ecology, which will be better situated to help address pressing questions in the contemporary seascape.
SEE-scapes begins with a conceptual mechanistic representation of the ecological drivers of behaviour in the contemporary marine environment (Figure 1), and an evaluation of what may or may not be relevant in a given focal system. Within the SEE-scape, ecological drivers will hereafter be called “nodes”. By outlining the interconnectedness of nodes, the SEE-scapes can help prevent oversimplification of the true ecological process in studies of marine animal behaviour at the design, analysis, and presentation stages. This approach complements contemporary statistical-modelling approaches, which incorporate observed probability, uncertainty, and hierarchical dependency—ensuring the viability of the SEE-scape as computing capacity and statistical approaches advance.
Research using the SEE-scape can investigate one or multiple nodes at once, with increasing inclusion providing a more and more precise representation of the true state of a marine system. We think this shift towards greater inclusion of possible drivers, and the subsequent recognition of statistical uncertainty should be the future focus in marine systems. Thinking objectively, the decision-making of individuals is influenced by more than just one factor, or one node, at a time. For example, the decision of where and when to eat does not just consider the tides or the availability of resources; instead, it likely considers the tides, the resources, the degree of risk given the time, the position of the self in relation to refuge and conspecifics, and more, in tandem. That’s not to say that individual, or a subset of, nodes will not dominate the decision, but how can we know whether there is missing variance, missing inputs, in our models of marine life if we do not try to include more information than just, for example, the tides. There are statistical methods available to ecologists now that enable the propagation of uncertainty and the inclusion of multiple potential nodes (Marcot et al., 2006; Hobbs and Hooten, 2015), and the SEE-scape can help ecologists identify which nodes are most relevant at the scale they are studying, and how computationally to handle the uncertainty (Figures 1 and 2). The SEE-scape workflow (Figure 2) is designed to help researchers visualize these suggestions, the types of analyses that can be conducted, and how multiple studies of individual or paired components can come together in either one hierarchical multi-level model of behaviour (Figure 2C. “Method 1”) or be used comparatively to evaluate the directional effects of different nodes (Figure 2C. “Method 2”), before outputs are generated that provide information on the behaviour of individuals, populations, or species based on the nodes considered.
“Ecological energy”, what do we mean? and, why?
From an evolutionary standpoint, individuals make decisions based on the potential resource-allocation consequences of a given action, either on themselves or on their offspring. Energy is often a convenient currency to track evolutionarily relevant resource use in behavioural ecology (see Stephens and Krebs, 1986, for further discussion). Evaluating the near-continuous dynamics of energetic income or expenditure is beyond the capacity of contemporary monitoring methods—however, organizing the various aspects of energy flux into broad ecological terms allows researchers to broadly capture these considerations in studies of tangible decisions and behaviours. While there is active development of empirical methods for measuring marine vertebrate energetics, these analyses are typically limited to kinetic movement and metabolic estimates. However, this field is rapidly developing, so there is value in reorganizing our perception of the marine environment in these terms of “ecological energy”, to prepare for the types of questions we can ask and the behaviours we can investigate once the future of empirical measurement is realized. For the purpose of this reorganization, we categorize ecological energy as those matters, items, or transactions that manifest as physical processes or responses, driving observed behaviour, and often dictating ecological outcomes or traits of individuals, species, populations, communities, or seascapes.
A new potential: the state of the art in marine sampling technologies and innovations
After identifying the relevant components of a study species’ SEE-scape, researchers need to identify the spatiotemporal scale at which these nodes should be measured. The scale at which we sample a focal species must be ecologically relevant both in a spatial and temporal sense—behaviour may not always be optimal, and individuals may opt for a heuristic approach that is undetectable except at the biologically and ecologically relevant scale. This idea is akin to the developments at the turn of the 21st century in laboratory studies of foraging behaviour, which identified that protocols must be ecologically informed to isolate the implications of context on behaviour (Dall et al., 1997). We propose this same consideration now for wild studies of marine species because the methodologies for studying a transient-ranging species will be entirely different from those to study a resident species; likewise, for those of “fast” versus “slow” life histories on temporal scales. Identifying the appropriate scale for a node requires balancing the specific research question(s) and the range of spatiotemporal resolutions accessible based on the technology available. One approach towards addressing relevant scale is to consider the associated SEE-scape level of the node of interest, i.e. the individual, species, community, or seascape level. Using this approach, we will now review and summarize when, how, and why some technologies will be appropriate for sampling SEE-scape nodes and focal behaviours based on the type of node and its hierarchical level in the SEE-scape (Figure 1).
Seascape and community level sampling
There are many factors to consider when picking a method for measuring upper-level nodes, such as habitat and bathymetry (Figure 1). Budget, ethics, and feasibility are central and will be site- and study-specific. But several recommendations can be made to develop the field of marine science and seascape ecology, as it applies to studies of animal behaviour at these higher levels of seascape and community (Figure 1).
For shallow water habitats, quadrat sampling (Balestri et al., 2003), sonar (Yesson et al., 2011), satellite imagery, and surveys using unoccupied aerial vehicles (UAVs) (Mancini et al., 2013) have shown great success in quantifying below-surface habitat types and vegetation composition (nodes: suitable habitat and refugia, Figure 1 and Table 1). In pelagic seascapes, tangible features like oceanographic currents, frontal zones, and the physical topography of the seafloor provide context against which marine animals act and can be identified via Earth observation and remote sensing (Miller, 2009). Researchers can use remote sensing to identify how community-level topographic features, like seamounts, interact with global seascape features to create “hotspots” of productivity. The predictability of these features offers an opportunity to make “elusive” pelagic species far more predictable; individuals, species, and communities can anticipate them, underlining their role as important seascape-level and community-level nodes (Figure 1 and Table 1) (Miller et al., 2015; Cox et al., 2016).
Below surface waters, innovations like lithium-powered autonomous underwater vehicles (e.g. Autosub Long Range) will offer novel perspectives and data on the bathymetric, physical, and oceanographic characteristics of deeper waters, which have historically been beyond the scope of other methodologies, e.g. sonar (Roper et al., 2021; Salavasidis et al., 2021) (nodes: bathymetry and oceanographic features, Figure 1 and Table 1).
A pressing consideration for seascape- and/or community-level nodes is how to measure the expanding influence of the Anthropocene. Exacerbated by climate change and a growing global need for marine resources, human–wildlife interactions are increasingly common and therefore relevant in assessing animal behaviour. Borrowing from terrestrial research, the “Accident’s paradigm” describes one of the most considerable ways in which the Anthropocene and climate change will affect behaviour (Wheatley et al., 2021). Consistent with paradigms like “Energy Landscapes” (Shepard et al., 2013; Gallagher et al., 2017) and the “Landscape of Fear” (Bleicher, 2017), “Accident Landscapes” describe how individuals’ will factor in the likelihood of an interaction resulting in harm, expensive avoidance, and/or death (Wheatley et al., 2021). Wheatley et al. (2021) identify (terrestrial) landscape features that could cause physical harm but contend that there is little evidence that accident landscapes are relevant to the marine environment. However, if viewed in the context of the Anthropocene, accidents are probable and highly relevant for marine vertebrates. A brief consideration suggests a few regular and likely sources: storms (Sherley et al., 2012; Sepúlveda et al., 2020); entanglement in lost, discarded, and active fisheries gear (Lewison et al., 2004, 2014; Crawford et al., 2017); ship strikes (Laist et al., 2001); extreme vertical and or horizontal displacement (e.g. in fishing equipment or ship ballast tanks); seismic surveys (McCauley et al., 2003; Nelms et al., 2016); collisions with renewable energy infrastructure (Inger et al., 2009; Fox et al., 2018); and anthropogenic disasters such as oil spills (Crawford et al., 2000; Votier et al., 2005).
As climate change continues to shift the global oceanographic and atmospheric patterns, natural phenomena, and human–wildlife interactions are likely to increase in frequency and intensity and may present new challenges to marine animals in the form of accidents and accident-avoidance (Sydeman et al., 2017; Piatt et al., 2020; Orgeret et al., 2021; Pacoureau et al., 2021; Herbert-Read et al., 2022). We recommend accidents as a relevant node for future studies of marine vertebrate behaviour, and researchers can account for accident nodes by identifying the distribution and likelihood of sources of potential accidents at the scale of the Seascape or Community, e.g. fisheries overlap identified via vessel monitoring systems cross-checked to study species distributions (Calich et al., 2018; Kroodsma et al., 2018; Queiroz et al., 2019; White et al., 2019) (nodes: Anthropocene, storm frequency and intensity, and bodily harm, Figure 1 and Table 1).
Species and individual level sampling
Technological advancements of the past decade have revolutionized the potential for sampling relevant nodes at the “Species” and “Individual” levels of the SEE-scape. In sampling nodes such as resources, refugia, distribution of species, and interspecific competition, the three-dimensional nature of the marine seascape requires the use of novel technologies developed or adapted specifically to meet the range of physiological and physical adaptations of species to the flux of the marine environment.
One such challenge of the seascape is our inability to immediately see below the surface. Baited remote underwater video systems (BRUVS), the marine parallel to terrestrial camera traps, are excellent for resolving this challenge. BRUVS have wide applicability in shallow, mid-pelagic, and deep water to evaluate the presence, absence, diversity, and distribution of species and resources (nodes: distribution of species and resources, Figure 1 and Table 1) and potentially to qualify space use, e.g. identifying reproductive habitat via observation of mating behaviour (Brooks et al., 2011; Clarke et al., 2019). Most commonly, BRUVS are deployed in shallow water habitats to define community composition as well as species-specific resources, their distributions, displacement, and inter-community connectivity (De Vos et al., 2015; Grimmel et al., 2020). For describing large “apex” predator presence and abundance, BRUVS are useful (Santana-Garcon et al., 2014; Clarke et al., 2019; Letessier et al., 2019). Particularly relevant for pelagic species, mid-pelagic BRUVS are the first approach of their kind to provide such a perspective and represent a fundamental methodology for applying the SEE-scapes perspective and workflow to cryptic marine species.
In the upper pelagic, aerial or satellite surveillance is relevant, albeit limited to applications targeting surface-breathing marine vertebrates and other pelagic species that migrate between the surface and deep water. Classically, aerial surveys were from piloted aircraft (Stewart et al., 2021), but UAV use is increasing and, as their application expands, guidelines for their use in marine research are beginning to appear (e.g. Brisson-Curadeau et al., 2017; Barnas et al., 2020; Raoult et al., 2020). Above and beyond flights by scientific organizations, the wide-spread and growing use of UAVs by hobbyists is already beginning to reveal previously unrecorded behaviours in coastal waters (Sims et al., 2022; Towner et al., 2022) and could offer new potential avenues for marine-facing citizen science projects (Hodgson et al., 2013; Garcia-Soto et al., 2021; Wood et al., 2021). Aerial or satellite images can allow researchers to monitor the occurrence, abundance, and distribution of species, resources, habitat, and refugia (Fretwell and Trathan, 2009; Fretwell et al., 2014) (Figure 1 and Table 1), and even assess physiological changes in larger species (Durban et al., 2021; Stewart et al., 2021).
Resources often need to be studied at these lower levels, and this can present a challenge in marine systems when a focal species may rely on mid-pelagic prey species that are not bound to coastal regions, e.g. forage fish or krill aggregations, for which BRUVS are not appropriate. The evolution of sonar technologies from vertical echo sounding to multibeam sonar has enabled a new era of pelagic research, where the technology can be effective at identifying fine-scale information and behavioural responses (Handegard et al., 2012; Rieucau et al., 2014). Multibeam sonar is effective in quantifying biomass, abundance, and behaviour of forage species (e.g. Nøttestad, 2002; Brierley and Cox, 2015) and has been applied to observe a range of marine predators as they attack aggregations of forage fish and krill (Nøttestad and Axelsen, 1999; Axelsen et al., 2001; Cox et al., 2009; Handegard et al., 2012). It’s necessary to note that different sonar technologies and different deployment arrangements can achieve different levels of resolution; for example, pelagic survey sonars cannot produce the time-series high-resolution imagery that set-ups like the stationary MARS echosounder deployed in California can, which has the capacity to identify the fine-scale diel movements of pelagic prey taxa and their responses to marine mammal predators over long time series (Urmy and Benoit-Bird, 2021). Logistic constraints mean these studies deploying high-resolution imaging sonar are either smaller in spatial or temporal scale, resulting in a rarity of studies that use sonar to characterize prey abundance at scales relevant to predator foraging behaviour (Cox et al., 2009; Campbell et al., 2019; McInnes et al., 2019). Nonetheless, and particularly as technology continues to advance, these sonar methods can still provide unique and informative data on cryptic mid-trophic species and pelagic species interactions.
Autonomous surface vehicles (ASVs) are poised to revolutionize pelagic sampling methodologies by overcoming the limitations of classic sonar deployment protocols (Gordon et al., 2020). Newer generations of these ASVs can be outfitted with solar panels to run fuel-free without crew for long periods (up to a year), continuously sampling. Current battery limitations mean they still carry standard echosounders, which can detect and measure aggregations of forage fish relevant to predators (e.g. Campbell et al., 2019), and progress is moving towards deploying ASVs with multibeam sonar to collect data on bathymetry, forage fish assemblages, and other ecological data, depending on the objective of the deployment (Kapetanović et al., 2020; Zube et al., 2022).
Another critical phenomenon to consider at this resolution is inter- and intra-specific competition (nodes: inter- and intra-specific competition, Figure 1 and Table 1). Competition—over resources, mates, refugia, and habitat—can shape the distribution of animals based on the density-dependent relationship between one species need for a resource and how many species or individuals are trying to exploit it, a concept famously described by the ideal free distribution theory (Fretwell and Lucas, 1969). In conjunction with density-dependent effects, factors dictating competition can include environmental conditions (typically modelled as the “suitability” of one space over another), the degree of species freedom to make decisions on their spatial distribution, proclivity, or dependence upon a geographic or oceanographic feature, interactions at the level of the species or individual, the species-specific capacity for memory, and population demography (see Planque et al., 2011 for a comprehensive review of these topics). While these factors are likely underpinning the observable patterns of inter- and intra-specific competition, personality and social context are fields of behavioural research that suggest competition, especially at the individual level, is also influenced by a species’ local and global rules for social behaviour (node: social context, Figure 1 and Table 1). Research suggests that at the group-level, animals will distribute themselves relative to their social context (Milinski, 1979; Atton et al., 2014), and at the individual level, the animal can gain advantages from social associations in the form of eco-evolutionary currency, e.g. information (Laland and Williams, 1997; Ward and Webster, 2019). Furthermore, personality-driven spatial behaviour can shift the predictions of density-dependent theorems, such as the ideal free distribution, via the cumulative effect of individuals personality-driven variation, thereby modulating the patterns of competition or resource harvesting outside the “ideal” (DiNuzzo and Griffen, 2020). In the Anthropocene, social context, personality, and the canonical drivers of competition will be increasingly relevant as human interests expand to overlap with marine species requirements, for example, in the form of fisheries-wildlife interactions (Riet-Sapriza et al., 2013; Sydeman et al., 2017; Dias et al., 2019; Arlinghaus et al., 2021).
Social context in a wild seascape can be studied by identifying overlap in the distribution of resources, refugia, mates, and species using methods that describe the spatiotemporal overlap of competitors, be that fish, birds, or mammals, including humans; and/or by monitoring multiple individuals and quantifying the associations between individuals and individuals “personalities” or behavioural suites. To do this, it will require local-scale deployments of video surveillance and/or sonar coupled with unique individual surveying techniques like colour bar tagging and biologging.
For sampling individuals’ movements, species migrations, or a population’s connectedness, aka a social network, biologging offers a range of opportunities: from passive to active, acoustic to satellite, there are a growing number of ways to track a marine vertebrate in situ. Biologging—“the use of miniaturized animal-attached tags for logging and/or relaying of data about an animal’s movements, behaviour, physiology and/or environment” (Rutz and Hays, 2009)—has revolutionized behavioural sampling, providing observations of otherwise hidden wild behaviour and giving researchers insight into ecological interactions occurring at previously unobservable spatiotemporal resolutions (e.g. Mourier et al., 2016). The application of biologging has grown and will continue to do so, with a key development being the (further) miniaturization and extended battery life of biologgers (Crossin et al., 2014; Wilson et al., 2015, 2015ab). The data from these tags can now inform on energy expenditure, social affiliations (e.g. Guttridge et al., 2010), internal states (e.g. Goldbogen et al., 2019), and in some cases, prey capture (Viviant et al., 2010; Watanabe and Takahashi, 2013). The latest generation of animal-borne cameras are also beginning to reveal previously unobserved behaviours, including predator–prey interactions (e.g. Thiebot et al., 2017), intraspecific communication (Thiebault et al., 2019; McInnes et al., 2020), breaching behaviour (Rudd et al., 2021), and courtship/mating behaviours (Rudd et al., 2021; Sims et al., 2022). The parallel development of machine learning and computer vision techniques to streamline video analysis (Okuyama et al., 2015; Manco et al., 2022) will likely lead to more discoveries of this nature in the future and make it easier still to directly sample the behaviour of some marine vertebrates. In some instances, biologgers are deployed with the intention to use the marine vertebrates natural behaviour to sample and survey their environment instead of the behaviour of the animal (Harcourt et al., 2019; Watanabe and Papastamatiou, 2023). This has been particularly valuable in regions like the Arctic or Southern Ocean, where seasonal oceanographic conditions like sea ice expansion limit accessibility for larger traditional oceanographic equipment, e.g. biologgers like Conductivity–Temperature–Depth sensors (CTDS), which have revolutionized the capacity of researchers to collect environmental data in these hard-to-reach areas (Lydersen et al., 2002; Roquet et al., 2013). Whether deployed to study behaviour or to use behaviour to survey a larger seascape, biologgers are a powerful tool for relating animals’ behaviour to “real-world” fitness consequences, connecting nodes across the SEEscape (Mosser et al., 2014; Pagano and Williams, 2019; Courbin et al., 2022).
Where circumstances do not permit biologging methods, the use of observational methodologies is an appropriate replacement to gather at a coarse scale the spatiotemporal distribution of a species (Yuan et al., 2017; Becker et al., 2019). These methods would be more limited than, for example, acoustic tracking because observational methods rarely provide the ability to identify individuals and, furthermore, may invite (more) observer bias. However, they can still achieve a version of the goal and provide a measure of species behaviour and distribution (Heim et al., 2021). Camera traps are a common terrestrial methodology towards this end (e.g. Bersacola et al., 2021), and in the marine environment, BRUVS can be widely applied as described above. ASVs and UAVs are other observational methods that are already implemented in the marine environment (e.g. Meinig et al., 2019), and where appropriate, ASV objectives can be altered to enable observation of species of interest while also conducting their typical objectives, usually environmental sampling. Furthermore, as ethical concerns of biologging become a standardized consideration in the marine environment, innovations like the Autosub (Roper et al., 2021; Salavasidis et al., 2021) have the potential to complement studies of animal behaviour where tagging is not an option, reaching areas of the ocean and species that are historically beyond the scope of other observational, sonar, and telemetric methodologies.
From any of these possible methods for collecting information on the drivers of the SEE-scapes, the double-edged sword of “big data” emerges. Sampling methods associated with marine ecology incur a time and expertise cost associated with the collation, handling, filtration, and extraction of data. And these steps must be undertaken before any analysis can be performed. Towards facilitating big data handling, novice-friendly graphical user interfaces [e.g. Langley et al., (2023)] and R packages (e.g. Flávio and Baktoft, 2021) are in near constant development to help researchers manage and parse the large data volumes associated with modern marine science. While a full review of the approaches being developed for data management is beyond the scope of this paper, there are some resources to help guide researchers through this tedious part of research, particularly as it relates to the management of marine big data (Grémillet et al., 2022).
Statistical approaches
We believe the most intuitive statistical approach for modelling and interpreting the data collected in Stages “A”, “B”, and “C” of the SEE-scapes workflow (Figure 2) is a Bayesian approach. Bayesian statistics is forgiving of the imprecision of ecological sampling yet still powerful enough to translate massive data inputs into comprehensive representations and predictions of ecological processes (Marcot et al., 2006; Hobbs and Hooten, 2015). Not only does a Bayesian hierarchical structure allow for inter-level feedback, mimicking natural processes, it incorporates prior knowledge, and most importantly, it permits the propagation of error. The latter is important because ecological data is likely to carry a large amount of measurement error, or uncertainty. By incorporating prior knowledge, each step builds upon the understanding of what preceded it, and the propagation of error allows final estimates of the true natural state to be representative of both observational and process error. This makes intuitive sense for modelling an ecological process, where behaviour is the reflection of integrated prior knowledge and a black box of decision-making (McNamara et al., 2006).
A statistical method that is highly compatible with the SEE-scapes framework, and can sit in either a frequentist or Bayesian approach, are multi-layer networks (MLNs,Silk et al., 2018). MLNs evolved from network analytical approaches and are fundamentally looking to determine the connections between nodes and “layers”, or the organization of many nodes into one network (Finn et al., 2019). Because MLNs integrate the interdependencies between “nodes” across and within levels of the animals’ ecology, the questions for future research with MLNs from a community ecology perspective are well aligned with the SEE-scapes objectives (Pilosof et al., 2017; Silk et al., 2018). Community ecology has already started to realize the potential of MLNs both in terrestrial social network analyses (e.g. Albery et al., 2021) and, with increasing volumes of “big data” being harvested from the marine environment, this approach is likely to be widely applicable in studies of complex marine seascapes.
A further statistical approach that is highly compatible with SEE-scapes are Bayesian structural equation models. Some work on the conditions for breeding success in colonial seabirds is a great example of the approach defined in stage “C” of the SEE-scapes framework and illustrates elegantly how this modelling approach can investigate multiple nodes of animal ecology and elucidate the combination of nodes that holds the most mathematical support in explaining an ecological phenomenon (Bennett et al., 2022).
Where a Bayesian method is not viable, there are many statistical approaches within a frequentist framework that can achieve great results within the SEE-scapes workflow. Methods such as path analysis and mixed modelling allow for comparison of individuals’ decision-making against risk and energy metrics (Miller et al., 2015), capturing the influence of large-scale atmospheric and oceanographic patterns. Optimal path, habitat, and resource selection models can also provide insight into what drives an individual’s selection of one habitat in one unit of time over all other habitats available in that unit of time (Muff et al., 2020; Trevail et al., 2021), modelling the spatiotemporal variance in the value of resources. Hidden Markov Models (HMMs) differentiate the activity levels of individuals as they move across an environment and are potentially very useful in incorporating internal state metrics into consideration of “costly times” and the daily partitioning of critical behaviours (Leos-Barajas et al., 2017; Quick et al., 2017; Trevail et al., 2019; Byrnes et al., 2021). HMMs are particularly interesting when measurements of oxygen consumption and metabolic demand, such as overall or vectorial dynamic body acceleration, are available to researchers, as these models incorporate information on physiological constraints and the in situ cost of movement, reflecting the kinetic constraints of decision-making.
And finally, isoenergy polygons (IEPs) use allometric equations to map the cost of space use and movement in relation to the landscape and conditions (Shepard et al., 2013) and can accommodate other nodes of ecological energy such as accident landscapes and refugia. For IEPs, movement costs are calculated using the most relevant metric, e.g. dive depth for cormorants (Leucocarbo atriceps) or incline in humans (Homo sapiens) (Shepard et al., 2013). IEPs allow the definition of expenditure relative to incomes across a whole study region based on the energy use and cost per unit time at a given spatial location in interaction with other relevant identified energetic nodes (Shepard et al., 2013). A further step available when using IEPs is integrating an optimal path analysis; this will identify the most likely shortest path based on “stressors” and “attractors” identified by life history traits, or, in this context, relevant nodes (Shepard et al., 2013; Niella et al., 2020).
Whether using Bayesian or frequentist methods, the goal of these statistical recommendations is the same: model individual behaviour in relation to the SEE-scape nodes in a method that is hierarchically and spatiotemporally dynamic. In this way, the statistical approach will fundamentally reflect the natural processes of the marine environment. There will certainly be existing or developing methods appropriate to studying the SEE-scape that we have not discussed here, but those we have highlighted are (for us) exemplar methods for detecting features that are visible or identifiable using contemporary technology and statistical approaches. In acknowledging the ever-evolving field of seascape ecology, an important point to highlight is that there are an unfathomable number of other sensory features that technology cannot yet access or understand (e.g. Gordon et al., 2020). Appearing on the horizon, initiatives like ICARUS (International Cooperation for Animal Research Using Space) and “WildFi” provide an example of how collaborations will drive technological advancement, particularly where biologging and population ecology are concerned (Wild et al., 2022). And, as organizations work together to optimize the value of novel technology and “big data” approaches (e.g. Grémillet et al., 2022), innovations like environmental DNA (eDNA) (Beng and Corlett, 2020) and “The Internet of Animals” (Wild et al., 2022) will revolutionize non-captive studies of animal behaviour. Nonetheless, the SEE-scapes framework has been designed to be relevant as technologies evolve and new ones are developed. In this way, it provides a long-lasting framework for marine ecologists when considering individuals in the context of their SEE-scape and in deciding on a methodological approach.
Summary
Historically, the marine environment has been beyond the technological capacity of ecological studies of behaviour and movement. With technological innovations, there is less and less reason why researchers cannot dive deeper into the marine realm and incorporate behavioural principles into studies of marine vertebrates. We have established that technology has advanced significantly and is on track to continue to meet researchers’ imaginations; that the marine habitat is dynamic and complex, and modern statistics for ecology can now provide the tools to handle such spatiotemporal variability; and finally, that individuals, species, communities, and seascapes are very complex, and approaches to studying any level of the seascape need to account for this complexity. Furthermore, we have introduced SEE-scapes, a novel framework for studying behaviour in the marine environment.
Especially in the marine environment, there is an unparalleled and immediate need for holistic and insightful research into the behaviour and interaction of species and the resilience of habitats, as so summarily described by a 2022 “horizon scanning” report conducted by 30 transdisciplinary experts (Herbert-Read et al., 2022). With awareness of the growing attention towards marine sciences and the apparent need for research, our ultimate goals with the SEE-scapes framework are: (1) to redirect marine research—from microbiologists to megafauna behaviouralists—to the issues of the modern seascape; (2) to facilitate the design of methodologies that capture the dynamism of their seascapes; and (3) to hopefully enable researchers to produce impactful, collaborative, and comparable outputs and outcomes for the scientific community and the longevity of the global ocean’s health. By capturing spatiotemporal variation and the interconnectedness of the seas, SEE-scapes hopes to eliminate outcomes in research where the inference is confounded or confused by a lack of representation of the natural variation of the seascape, resulting in prolonged controversial debate. As ecologists race against a climate crisis and look to protect the remaining biodiversity of the global oceans, we offer SEE-scapes to provide the necessary new perspective.
Acknowledgement
We are grateful to the Marine Predator Ecology and Conservation Research Group at the University of Exeter for their consultation and discussion of the work. Thank you to Stephen Lang, Liam Langley, Alice Trevail, and Steven Votier for discussion and comments on manuscript drafts. Thank you to Illustrative Science and the women behind the artwork for the SEE-scapes framework.
Funding
RBS was supported by the Pew Fellows Programme in Marine Conservation at the Pew Charitable Trusts. The views expressed in this paper are those of the authors and do not necessarily reflect the views of the Pew Charitable Trusts.
Conflicts of Interest
The authors declare no conflicts of interest.
Author contributions
MMK conceived the review with input from co-authors RBS and SXD. MMK wrote the manuscript. All co-authors commented on the manuscript and approved the final version.
Data availability statement
No datasets were used in this review.