-
PDF
- Split View
-
Views
-
Cite
Cite
P Gray, C Garcia, C Robinson, J Bremner, A method for Identifying sensitivity of marine benthic invertebrates to ocean acidification through a biological traits approach, ICES Journal of Marine Science, Volume 79, Issue 7, September 2022, Pages 2117–2125, https://doi.org/10.1093/icesjms/fsac146
- Share Icon Share
Abstract
Ocean acidification poses a major threat to the structure and diversity of marine ecosystems. The marine seabed sustains important ecosystem functions, and so understanding the sensitivity to increased pCO2 within benthic invertebrates is critical for informing future management strategies. Here, we explore a traits-based approach for estimating the sensitivity of benthic taxa to ocean acidification, using data from the western area of the North Sea. We selected 56 taxa across 11 taxonomic groups representative of the various habitats found in the region. Biological traits considered sensitive to elevated pCO2 were identified from literature review, and the taxa were scored for each trait to produce a total relative sensitivity (TRS) index. We investigated differences in sensitivity between the taxa and across habitats and explored whether sensitivity was spatially aggregated. Our analyses indicated that benthic species are sensitive to acidification, with 51% of the taxa scoring in the top three TRS bands overall, and hot spots of sensitivity being more widely distributed across the region than corresponding "cold spots" (low sensitivity). The opportunities and limitations of the approach are discussed.
Introduction
Since the Industrial Revolution, our planet has been transforming with an extent and pace not seen since the Cretaceous–Paleogene mass-extinction (Zeebe et al., 2016). One manifestation of this is the change to ocean chemistry driven by anthropogenic increases in atmospheric CO2, with a 30% increase in surface ocean acidity since the pre-Industrial era, and projections of a pH of between 8.05 and 7.75 by 2100 (EEA, 2019). Such drastic changes to ocean chemistry are expected to cause significant and lasting damage to marine ecosystems, particularly among benthic invertebrates (Kroeker et al., 2011).
Benthic organisms play a vital role in maintaining the marine environment, contributing to sedimentary organic matter cycling through bioturbation (Aller and Cochran, 2019), and inorganic nutrient exchange with the water column (Griffiths et al., 2017). Benthic invertebrates are also a key component of the diet of many pelagic organisms, notably during the juvenile life stage of commercially important fish species (Hüssy et al., 1997). In the event of the realization of ocean acidification (OA) projections, it is likely that the ability of some benthic invertebrates to continue these roles will be compromised, potentially further disrupting marine ecosystems and the vital services they provide (Barbier, 2017). The ecological importance and likely vulnerability of these organisms are why it is critical to determine the spatial distribution of specific groups likely to be most sensitive to OA, to identify areas in need of specific conservation efforts (Degraer et al., 2019). As highlighted by Degraer et al., the North Sea is one such area, which could benefit from further analysis.
Biological traits analysis (BTA) is a well-established methodology for assessing the impact of stressors on organisms, populations, and ecosystems (Bremner, 2008; Foden et al., 2013; Kenny et al., 2018). Organisms are considered according to attributes of their biology and ecology rather than phylogeny, allowing for easier mechanistic links between impacts and traits, rather than impacts and species. The transferrable nature of this analysis is particularly appropriate for first-stage assessments of the sensitivity of marine taxa to OA, since it utilizes available natural history data and does not require the impractical and laborious task of laboratory testing on multiple groups of species. Traits-based approaches have been used by others to estimate sensitivity to climate change (Foden et al., 2013; Hare et al., 2016), though they do not always consider OA in detail or provide empirical justification for the traits selected as measures of sensitivity. Here, we adapted these climate vulnerability approaches to develop a sensitivity metric that considers OA specifically and focuses on traits for which the link between the trait and OA-sensitivity is evidenced in the literature.
To explore how our metric performs in a spatial context, we used a large dataset from the UK North Sea: an aggregation of publicly available benthic data, which have already been utilized in research aimed at informing and influencing management of the North Sea seabed (Cooper and Barry, 2017; Cooper et al., 2019; Cooper and Barry, 2020). Our underlying premise is that some benthic species exhibit traits that make them particularly susceptible to OA, and by evaluating and ranking species across these traits, we can calculate a metric of sensitivity that is applicable across groups and geographies. For the metric to be useful in this context, it should be able to separate species across a sensitivity gradient and to detect spatial variability in sensitivity between benthic communities. We thus explored these two properties, investigating differences in sensitivity between taxonomic groups, across habitats and in terms of spatial clustering.
Material and methods
Species data preparation
The OneBenthic database (https://openscience.cefas.co.uk/ob_obdetgc/) held, at the time of data extraction in April 2020, ∼650000 samples from the seas surrounding the United Kingdom, with collection dates ranging from 1969 to 2015. Many samples within this database were initially intended for use in surveys relating to infrastructure and extraction projects, such as oil and gas exploitation or aggregate extraction. Entries are heavily clustered due to this, leading to a highly variable coverage across the North Sea. Extensive cleaning of the data was required to remove unsuitable or superfluous information. This involved removing samples outside of our study area, ensuring uniformity of collection methods (sieve of 1mm mesh size and quantitative sampling gears), and to further reduce bias in the analysis, we also removed all samples collected before 1 January 2000. The extents of the North Sea were taken as defined by the International Hydrographic Organisation (1953), and a shapefile sourced from Marineregions.org.
After these cleaning stages, 2832 taxa remained. BTA can be a time-consuming process for very large taxon lists, particularly when traits are scored for the first time. Such a large number of taxa was not practical for the aims of the study, which was to understand the potential of the metric. We thus focussed on the most abundant taxa. The data were sorted by EUNIS habitat class (https://www.eea.europa.eu/data-and-maps/data/eunis-habitat-classification-1), with the 30 most abundant taxa from each habitat retained. Records that could not be identified to at least the genus level were removed from the dataset (because it is difficult to assign biological traits to mixed groups), and the next most abundant taxon was chosen to replace it. The final database used for further analysis was composed of a list of 56 taxa and 76036 entries.
Biological traits identification, collation, and scoring
Traits that the scientific community consider to be relevant to species’ responses to elevated ocean pCO2 were identified through a literature review, using terms such as “benthic invertebrates”, “OA”, and “sensitivity”, using the Google Scholar search engine. Traits listed or described in the records returned by the literature review were collated, with the criterion that they must be evidentially linked to a response to the stressor, and discrete. We selected six traits that have been found to mediate the species level of risk to a change in pCO2 in the environment, four of which were different from our final list. A major concern at this stage was the likelihood of finding high-quality sources confirming these traits for each taxon catalogued. With this in mind, two traits (lifespan, infaunal/epifaunal adult stage) were removed, and a further two were adapted (length of larval stage > larval stage type and feeding; exoskeleton and chemical composition > acid sensitive structures).
The final traits chosen related to body structural integrity (the presence of acid-sensitive structures), capacity to buffer against elevated internal pCO2 (mobility and reproductive strategy), and the persistence of future generations (reproductive strategy and larval stage) (Table 1; Supplementary Appendix S1). Each trait was divided into three categories that describe the different ways a trait is expressed for a given taxon and the categories were scored from 3 (most sensitive) to 1 (least sensitive) for their relative sensitivity to CO2 based on the evidence from the literature review, giving a relative sensitivity score (RSS) for each trait (Table 1).
Biological trait . | RSS . | Justification . | |
---|---|---|---|
Acid-sensitive structures | External | 3 | Taxa with chitinous (Long et al., 2019) and calcareous (Byrne and Fitzer, 2020) exoskeletons have been found to exhibit severe deformities when exposed to near-future CO2 concentrations, negatively impacting feeding, reproduction, movement, and mechanical protection. Calcification rates decrease in waters with elevated pCO2, leading to reductions in exoskeleton size by as much as 50% (Díaz-Castañeda et al., 2019). Differences in RSS between external and internal acid-sensitive structures were determined by assessing the likelihood of exposure to increased acidity in the surrounding medium. |
Internal | 2 | ||
None | 1 | Theoretical absence of negative effects associated with the absence of acid-sensitive structures. | |
Adult mobility (Quierós et al. scores) | Sessile (*1 and 2) | 3 | Organisms capable of higher levels of activity are subject to more anaerobic respiration than slow-moving or sessile taxa. Organisms that regularly respire anaerobically are more capable of buffering increased pCO2 to prevent acidosis and are more efficient at removing excess internal CO2 (Melzner et al., 2009), with those displaying a more hypometabolic mode of life between and within taxonomic groups capable of less acid-base regulation (Pörnter, 2008). More active taxa have been found to be broadly more resistant to rapid increases in oceanic pCO2 throughout other mass-extinction events (Knoll et al., 1996), and to be less susceptible to ocean conditions projected by the year 2100 (Kelly and Hofmann, 2012). |
Slow (*3) | 2 | ||
Free (*4) | 1 | ||
Reproduction strategy | Broadcast | 3 | Evidence that increased ocean pCO2 can decrease fertilisation success, as a reduction in sperm velocity and intracellular Ca2+ oscillations decrease the probability of gamete fusion per collision (Shi et al., 2017; Colen et al.,2012). |
External brooding | 2 | Brooding species have been found to outcompete non-brooding species in environments with elevated pCO2 and show a higher ability to adapt to environmental changes (Lucey et al., 2015). Brooders are often exposed to elevated pCO2 due to a concentration of respiratory waste, with this fluctuating particularly intensely in internal brood chambers, where parental-stress-induced isolation can result in the pH of the chamber falling as low as 7.46 (Cole et al., 2016). The requirement of brooding organisms to withstand high acidity during this stage is thought to allow for greater tolerance to high pCO2 later in life (Gray et al., 2019). | |
Internal brooding | 1 | ||
Larval stage | Planktotrophic | 3 | Evidence that taxa with pelagic larval stages are more sensitive to increases in ocean pCO2 than direct developing counterparts (Lucey et al., 2015), with the transition from pelagic larvae to the benthic adult form being energetically taxing and creating a survivability bottleneck (Díaz-Castañeda et al., 2019). Algae exposed to projected levels of ocean pCO2 have less nutritional value, with lower protein and organic contents, requiring compensatory feeding (Duarte et al., 2016). This increases the risk of larval malnutrition, potentially causing severe latent effects (Pechenik and Tyrell, 2015). |
Lecithotrophic | 2 | Lecithotrophic larvae either derive nutrition from maternal yolk reserves or are non-feeding at this stage (Kempf and Hadfield, 1985), and so are not subject to feeding-related stress. Studies have found lecithotrophs to both benefit (Dupont et al., 2010) and be severely disadvantaged (Verkaik et al., 2016) by exposure to low pH conditions. | |
Direct development or non-pelagic | 1 | Not subject to the stresses of planktonic development. |
Biological trait . | RSS . | Justification . | |
---|---|---|---|
Acid-sensitive structures | External | 3 | Taxa with chitinous (Long et al., 2019) and calcareous (Byrne and Fitzer, 2020) exoskeletons have been found to exhibit severe deformities when exposed to near-future CO2 concentrations, negatively impacting feeding, reproduction, movement, and mechanical protection. Calcification rates decrease in waters with elevated pCO2, leading to reductions in exoskeleton size by as much as 50% (Díaz-Castañeda et al., 2019). Differences in RSS between external and internal acid-sensitive structures were determined by assessing the likelihood of exposure to increased acidity in the surrounding medium. |
Internal | 2 | ||
None | 1 | Theoretical absence of negative effects associated with the absence of acid-sensitive structures. | |
Adult mobility (Quierós et al. scores) | Sessile (*1 and 2) | 3 | Organisms capable of higher levels of activity are subject to more anaerobic respiration than slow-moving or sessile taxa. Organisms that regularly respire anaerobically are more capable of buffering increased pCO2 to prevent acidosis and are more efficient at removing excess internal CO2 (Melzner et al., 2009), with those displaying a more hypometabolic mode of life between and within taxonomic groups capable of less acid-base regulation (Pörnter, 2008). More active taxa have been found to be broadly more resistant to rapid increases in oceanic pCO2 throughout other mass-extinction events (Knoll et al., 1996), and to be less susceptible to ocean conditions projected by the year 2100 (Kelly and Hofmann, 2012). |
Slow (*3) | 2 | ||
Free (*4) | 1 | ||
Reproduction strategy | Broadcast | 3 | Evidence that increased ocean pCO2 can decrease fertilisation success, as a reduction in sperm velocity and intracellular Ca2+ oscillations decrease the probability of gamete fusion per collision (Shi et al., 2017; Colen et al.,2012). |
External brooding | 2 | Brooding species have been found to outcompete non-brooding species in environments with elevated pCO2 and show a higher ability to adapt to environmental changes (Lucey et al., 2015). Brooders are often exposed to elevated pCO2 due to a concentration of respiratory waste, with this fluctuating particularly intensely in internal brood chambers, where parental-stress-induced isolation can result in the pH of the chamber falling as low as 7.46 (Cole et al., 2016). The requirement of brooding organisms to withstand high acidity during this stage is thought to allow for greater tolerance to high pCO2 later in life (Gray et al., 2019). | |
Internal brooding | 1 | ||
Larval stage | Planktotrophic | 3 | Evidence that taxa with pelagic larval stages are more sensitive to increases in ocean pCO2 than direct developing counterparts (Lucey et al., 2015), with the transition from pelagic larvae to the benthic adult form being energetically taxing and creating a survivability bottleneck (Díaz-Castañeda et al., 2019). Algae exposed to projected levels of ocean pCO2 have less nutritional value, with lower protein and organic contents, requiring compensatory feeding (Duarte et al., 2016). This increases the risk of larval malnutrition, potentially causing severe latent effects (Pechenik and Tyrell, 2015). |
Lecithotrophic | 2 | Lecithotrophic larvae either derive nutrition from maternal yolk reserves or are non-feeding at this stage (Kempf and Hadfield, 1985), and so are not subject to feeding-related stress. Studies have found lecithotrophs to both benefit (Dupont et al., 2010) and be severely disadvantaged (Verkaik et al., 2016) by exposure to low pH conditions. | |
Direct development or non-pelagic | 1 | Not subject to the stresses of planktonic development. |
RSS = ranging from more sensitive to increased pCO2 (3) to least sensitive to increased pCO2 (1). *The 1–4 scale within the Adult Mobility category refers to the scale used to define movement of benthic invertebrates by Quierós et al. (2013).
Biological trait . | RSS . | Justification . | |
---|---|---|---|
Acid-sensitive structures | External | 3 | Taxa with chitinous (Long et al., 2019) and calcareous (Byrne and Fitzer, 2020) exoskeletons have been found to exhibit severe deformities when exposed to near-future CO2 concentrations, negatively impacting feeding, reproduction, movement, and mechanical protection. Calcification rates decrease in waters with elevated pCO2, leading to reductions in exoskeleton size by as much as 50% (Díaz-Castañeda et al., 2019). Differences in RSS between external and internal acid-sensitive structures were determined by assessing the likelihood of exposure to increased acidity in the surrounding medium. |
Internal | 2 | ||
None | 1 | Theoretical absence of negative effects associated with the absence of acid-sensitive structures. | |
Adult mobility (Quierós et al. scores) | Sessile (*1 and 2) | 3 | Organisms capable of higher levels of activity are subject to more anaerobic respiration than slow-moving or sessile taxa. Organisms that regularly respire anaerobically are more capable of buffering increased pCO2 to prevent acidosis and are more efficient at removing excess internal CO2 (Melzner et al., 2009), with those displaying a more hypometabolic mode of life between and within taxonomic groups capable of less acid-base regulation (Pörnter, 2008). More active taxa have been found to be broadly more resistant to rapid increases in oceanic pCO2 throughout other mass-extinction events (Knoll et al., 1996), and to be less susceptible to ocean conditions projected by the year 2100 (Kelly and Hofmann, 2012). |
Slow (*3) | 2 | ||
Free (*4) | 1 | ||
Reproduction strategy | Broadcast | 3 | Evidence that increased ocean pCO2 can decrease fertilisation success, as a reduction in sperm velocity and intracellular Ca2+ oscillations decrease the probability of gamete fusion per collision (Shi et al., 2017; Colen et al.,2012). |
External brooding | 2 | Brooding species have been found to outcompete non-brooding species in environments with elevated pCO2 and show a higher ability to adapt to environmental changes (Lucey et al., 2015). Brooders are often exposed to elevated pCO2 due to a concentration of respiratory waste, with this fluctuating particularly intensely in internal brood chambers, where parental-stress-induced isolation can result in the pH of the chamber falling as low as 7.46 (Cole et al., 2016). The requirement of brooding organisms to withstand high acidity during this stage is thought to allow for greater tolerance to high pCO2 later in life (Gray et al., 2019). | |
Internal brooding | 1 | ||
Larval stage | Planktotrophic | 3 | Evidence that taxa with pelagic larval stages are more sensitive to increases in ocean pCO2 than direct developing counterparts (Lucey et al., 2015), with the transition from pelagic larvae to the benthic adult form being energetically taxing and creating a survivability bottleneck (Díaz-Castañeda et al., 2019). Algae exposed to projected levels of ocean pCO2 have less nutritional value, with lower protein and organic contents, requiring compensatory feeding (Duarte et al., 2016). This increases the risk of larval malnutrition, potentially causing severe latent effects (Pechenik and Tyrell, 2015). |
Lecithotrophic | 2 | Lecithotrophic larvae either derive nutrition from maternal yolk reserves or are non-feeding at this stage (Kempf and Hadfield, 1985), and so are not subject to feeding-related stress. Studies have found lecithotrophs to both benefit (Dupont et al., 2010) and be severely disadvantaged (Verkaik et al., 2016) by exposure to low pH conditions. | |
Direct development or non-pelagic | 1 | Not subject to the stresses of planktonic development. |
Biological trait . | RSS . | Justification . | |
---|---|---|---|
Acid-sensitive structures | External | 3 | Taxa with chitinous (Long et al., 2019) and calcareous (Byrne and Fitzer, 2020) exoskeletons have been found to exhibit severe deformities when exposed to near-future CO2 concentrations, negatively impacting feeding, reproduction, movement, and mechanical protection. Calcification rates decrease in waters with elevated pCO2, leading to reductions in exoskeleton size by as much as 50% (Díaz-Castañeda et al., 2019). Differences in RSS between external and internal acid-sensitive structures were determined by assessing the likelihood of exposure to increased acidity in the surrounding medium. |
Internal | 2 | ||
None | 1 | Theoretical absence of negative effects associated with the absence of acid-sensitive structures. | |
Adult mobility (Quierós et al. scores) | Sessile (*1 and 2) | 3 | Organisms capable of higher levels of activity are subject to more anaerobic respiration than slow-moving or sessile taxa. Organisms that regularly respire anaerobically are more capable of buffering increased pCO2 to prevent acidosis and are more efficient at removing excess internal CO2 (Melzner et al., 2009), with those displaying a more hypometabolic mode of life between and within taxonomic groups capable of less acid-base regulation (Pörnter, 2008). More active taxa have been found to be broadly more resistant to rapid increases in oceanic pCO2 throughout other mass-extinction events (Knoll et al., 1996), and to be less susceptible to ocean conditions projected by the year 2100 (Kelly and Hofmann, 2012). |
Slow (*3) | 2 | ||
Free (*4) | 1 | ||
Reproduction strategy | Broadcast | 3 | Evidence that increased ocean pCO2 can decrease fertilisation success, as a reduction in sperm velocity and intracellular Ca2+ oscillations decrease the probability of gamete fusion per collision (Shi et al., 2017; Colen et al.,2012). |
External brooding | 2 | Brooding species have been found to outcompete non-brooding species in environments with elevated pCO2 and show a higher ability to adapt to environmental changes (Lucey et al., 2015). Brooders are often exposed to elevated pCO2 due to a concentration of respiratory waste, with this fluctuating particularly intensely in internal brood chambers, where parental-stress-induced isolation can result in the pH of the chamber falling as low as 7.46 (Cole et al., 2016). The requirement of brooding organisms to withstand high acidity during this stage is thought to allow for greater tolerance to high pCO2 later in life (Gray et al., 2019). | |
Internal brooding | 1 | ||
Larval stage | Planktotrophic | 3 | Evidence that taxa with pelagic larval stages are more sensitive to increases in ocean pCO2 than direct developing counterparts (Lucey et al., 2015), with the transition from pelagic larvae to the benthic adult form being energetically taxing and creating a survivability bottleneck (Díaz-Castañeda et al., 2019). Algae exposed to projected levels of ocean pCO2 have less nutritional value, with lower protein and organic contents, requiring compensatory feeding (Duarte et al., 2016). This increases the risk of larval malnutrition, potentially causing severe latent effects (Pechenik and Tyrell, 2015). |
Lecithotrophic | 2 | Lecithotrophic larvae either derive nutrition from maternal yolk reserves or are non-feeding at this stage (Kempf and Hadfield, 1985), and so are not subject to feeding-related stress. Studies have found lecithotrophs to both benefit (Dupont et al., 2010) and be severely disadvantaged (Verkaik et al., 2016) by exposure to low pH conditions. | |
Direct development or non-pelagic | 1 | Not subject to the stresses of planktonic development. |
RSS = ranging from more sensitive to increased pCO2 (3) to least sensitive to increased pCO2 (1). *The 1–4 scale within the Adult Mobility category refers to the scale used to define movement of benthic invertebrates by Quierós et al. (2013).
Each taxon was then given a score for each trait according to the RSS scoring system, using traits information generated from a literature search in Google Scholar. Information was obtained from >70 unique sources, the vast majority of which (89%) being peer-reviewed (Supplementary Appendix S2). Where information of this standard was not available for taxa, details were inferred from close relatives or gathered from reputable non-peer-reviewed sources, such as Encyclopaedia Britannica. Each entry in the resulting traits data matrix was prescribed a subjective confidence level to reflect the quality of the evidence (criteria can be found in Supplementary Appendix S2). While these confidence scores were not formally included in our analysis, we suggest they are a useful tool for identifying which entries could be improved in the future.
The scores for each taxon were summed across the four traits to give a total relative sensitivity (TRS). The TRS ranges from a theoretical minimum of 4 (no sensitive structures, free-moving and insensitive reproduction) to a maximum of 12 (sensitive structures, sessile, sensitive reproduction). All traits were weighted equally, as confidence in the differences in sensitivity between traits was not sufficient for different weightings to be considered valid and justified. Data management for taxa and samples were undertaken using the R statistical and programming environment and the package tidyverse (Wickham et al., 2019; R Core Team, 2021). Data were originally split by individual taxa for each sample. To prepare for spatial analysis, single scores were calculated for samples based on the mean TRS of all catalogued taxa included, resulting in a final list of 11845 samples. This has the advantage of producing a single, spatially defined figure for TRS that is easier to process and analyse, however, has the potential to exaggerate differences through its sensitivity to outliers.
Data analysis
Data were imported into ArcMap 10.6.1 and displayed through the creation of a shapefile feature class containing sensitivity information. To identify any clusters of significantly higher or lower sensitivity scores, the ArcMap tool Hot Spot Analysis (Getis-Ord Gi*) was utilized, with the Zone of Indifference method of conceptualization of spatial relationships used (Esri, 2021). This tool analyses features within the context of surrounding features, where the local sum of a feature and its neighbours are compared proportionally to the sum of all features. If the local sum is significantly higher, then the feature is considered to be within a hot spot. Identifying such areas assists in establishing which locations may have a higher proportion of benthic invertebrates that are potentially more vulnerable to increased ocean pCO2.
Results
Sensitivities amongst taxa
The benthic taxa TRS scores ranged from 6 to 12, out of the theoretical scoring range of 4–12. Sensitivity was generally high across taxa, with 55% of the taxa scoring 10 or higher. Amphipods, bivalves, and polychaetes differed in their TRS [Kruskal–Wallis (K–W) H = 19.219, p < 0.05], with Bivalvia having a higher mean TRS than both Polychaeta (Dunn–Bonferroni x = 16.118, p = 0.02) and Amphipoda (Dunn–Bonferroni x = −26.171, p < 0.01), while Amphipoda and Polychaeta were not significantly different (Dunn–Bonferroni x = −10.054, p = 0.19; Figure 1).
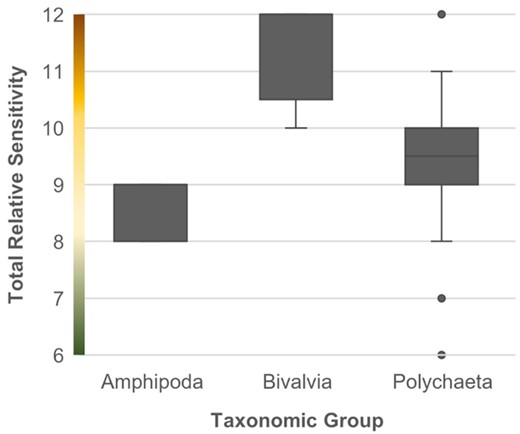
TRS of the three best-represented taxonomic groups: Amphipoda (N = 7), Bivalvia (N = 10), and Polychaeta (N = 28). Graduated colours on the y-axis represent high (brown), medium (yellow), and low (green) relative sensitivity values.
To delve deeper into the drivers of the sensitivity scores, Figure 2 details the spread of RSS across the four traits. High (3) RSS were dominant across all four traits. Both reproduction-based traits (reproduction strategy and larval stage) were similar in the proportions of RSS. Within the adult mobility trait, 98% of taxa catalogued had high and medium (2) RSS, with only one taxon, Scalibregma inflatum, being awarded a low score. The acid-sensitive body structure category was near-evenly split between high (3) and low (1) sensitivity, with similarly only one taxon receiving a medium score. Three groups (Amphipoda, Bivalvia, Polychaeta) were represented to a much greater extent than the others, so much of the information in the figure was driven by these taxa. Further analysis was conducted to reveal which traits drove these differences in sensitivity; there were no significant differences in scoring for the adult mobility trait (K–W H = 0.971, p = 0.615); however, the acid-sensitive structures (K–W H = 25.553, p < 0.01), larval stage (K–W H = 20.708, p < 0.01), and reproduction strategy (K–W H = 18.130, p < 0.01) traits each demonstrated significant differences. The polychaetes were significantly less sensitive than the other two groups in terms of acid-sensitive structures (Dunn–Bonferroni x = 17.679, p < 0.01), and amphipods had a significantly lower sensitivity than polychaetes and bivalves in terms of reproductive strategy (amphipods–polychaetes: Dunn–Bonferroni x = −17.571, p < 0.01) (amphipods–bivalves: Dunn–Bonferroni x = 18.3, p = 0.01) and larval stages (amphipods–polychaetes: Dunn–Bonferroni x = −20.571, p < 0.01) (amphipods–bivalves: Dunn–Bonferroni x = 23.4, p < 0.01; Figure 3).
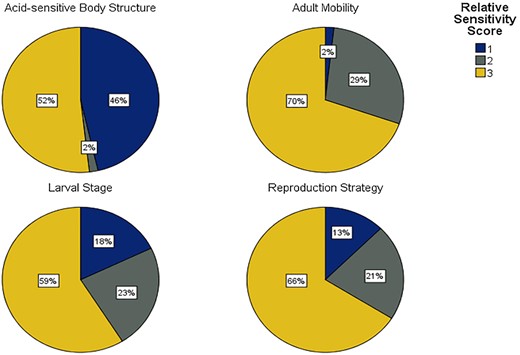
The proportions of relative sensitivity values across all 56 catalogued taxa. 1 = low, 2 = medium, and 3 = high.
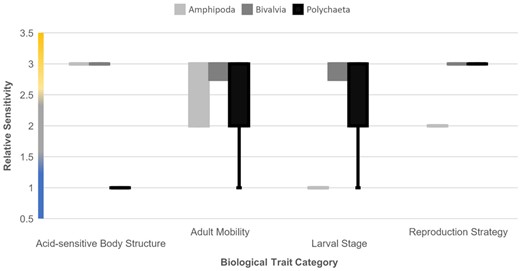
RSS for each biological trait category across the three most well-represented taxonomic groups. Graduated colours on the y-axis represent high (amber), medium (grey), and low (blue) relative sensitivity values.
Differences between habitats
Figure 4 summarizes the spread of mean TRS across EUNIS habitat types. There was a significant difference in mean TRS between many of the habitats (K–W H = 663.055, p < 0.01), with Dunn–Bonferroni pairwise comparisons showing that A5.37 (deep circalittoral mud) had significantly higher sensitivity than 8 of the other 11 EUNIS habitats (Figure 1). Notably, none of the significant pairings included the two least represented EUNIS habitat types, A5.33/34 and A5.43 (infralittoral sandy mud and infralittoral mixed sediments, respectively), possibly due to the few samples available for analysis between these and the better-represented habitat types; the most and least represented habitat types, A5.15 (deep circalittoral coarse sediments) at 3120 samples and A5.33/34 at 22 samples, respectively, differed by two orders of magnitude.
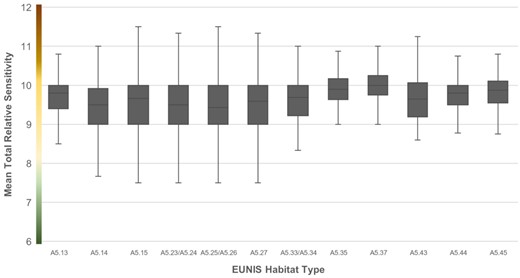
Boxplot of Mean Total Relative Sen4: Boxplot of Mean Total Types. Graduated colours on the y-axis represent high (amber), medium (grey), and low (blue) relative sensitivity values.
Spatial clustering
Figure 5 presents the geographic spread of mean TRS across the North Sea case study region, giving an overview of the distribution of samples (i.e. benthic communities) and their respective mean TRS across the community at each location. Some areas were relatively homogenous in terms of sensitivity (e.g. the moderately sensitive areas off the northeast English coast), while other regions such as England's southeast coast hosted a heterogenous mix of high-, medium-, and low-sensitivity
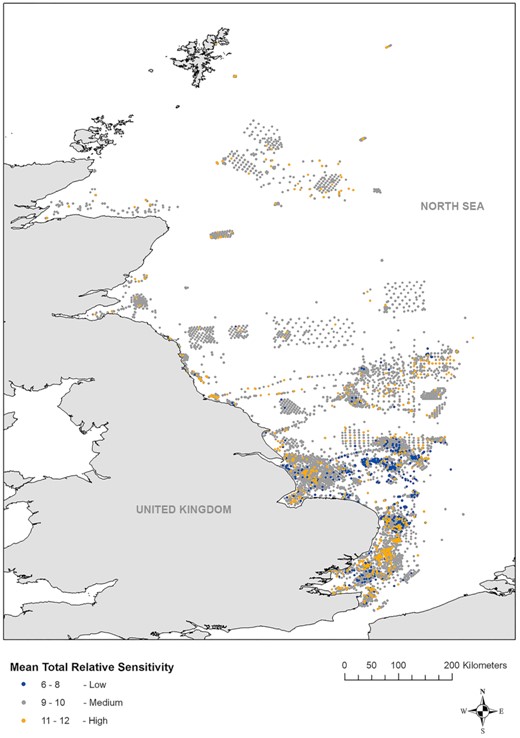
Samples plotted within the North Sea, with symbol colours indicating benthic invertebrate mean TRS scores.
communities. There was evidence of spatial clustering of sensitivity; Hot Spot Analysis (Getis-Ord Gi*) identified a large clustering of sensitivity hotspots along the Essex and Suffolk coasts (Figure 6a), the Humber Estuary (Figure 6b), the eastern part of Dogger Bank (Figure 6c), south of the Shetland Islands (Figure 6d), and across the east coast of Northern England and Scotland (Figure 6). Statistically significant cold spots (low-sensitivity communities) were largely confined to the Norfolk coast seawards (Figure 6e), extending as far as the south of Dogger Bank. There were several scattered cold spots at the western edge of Dogger Bank, but none in the northern UK North Sea.
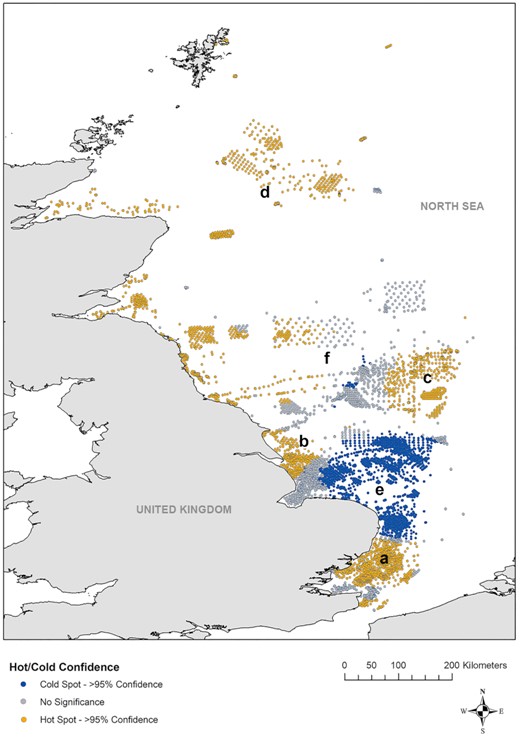
Output of a Hot Spot Analysis (Getis-Ord Gi*) of mean relative sensitivity of samples within the North Sea, displaying the locations of statistically significant clusters of high and low mean relative sensitivity values (p < 0.05).
Discussion
Using a biological traits-driven sensitivity assessment methodology has allowed us to estimate vulnerability to OA across a range of benthic invertebrate taxa within a spatial framework that identifies locations of increased or decreased sensitivity. Application to a subset of taxa from the western North Sea has shown the potential of the approach for identifying risks. Within our dataset, sensitivity was estimated to be highest in bivalves and in communities inhabiting deep circalittoral muds—a habitat typically dominated by polychaetes, with some bivalves also present (EEA, 2022). Sensitivity was often spatially heterogenous at a local scale, with locations of high, medium, and low sensitivity found in proximity to each other, but larger-scale homogeneity (hotspots and coldspots) was suggested across the region. The case study application used a subset of the total invertebrate complement of North Sea benthos communities and as such is not being proposed as a definitve answer on the sensitivity of the North Sea benthos; here it serves to illustate the power of the approach.
A biological traits-based approach to assessing sensitivity across a large and diverse set of taxa provides a way of simplifying the complexities that come with ecological analysis on such a scale. This method strikes a good compromise between practicality and the applicability of results to real-world ecological communities. However, this kind of analysis is heavily dependent upon high-quality, detailed traits information being available for many species (Degen et al., 2018). This was mitigated somewhat by studying a subset of taxa, a strategy used in similar research (Hare et al., 2016). More obscure taxa may lack a precise description of the target attributes, as was found while conducting this study. In cases such as these, biological traits may be inferred through the traits of closely related species, such as within the same genus or family. This presents its own set of challenges, however, as some traits may be inconsistent between even closely related species. While the uncertainty of the precision or accuracy of sources can be quantified somewhat through confidence scores, there is yet no clear method for incorporating these into analyses. Despite this, it is a way of dealing with the use of biological traits, which is well established (Bolam et al., 2014).
One of the key drivers for choosing the biological traits categories was to attempt to capture the nuances of sensitivity to increased ocean pCO2, by cataloguing and scoring a diverse set of biological attributes linked to sensitivity to this change (see Table 1). The adult mobility trait, while being of sound scientific reasoning, did not in the end assist in capturing this nuance. Compared to other traits used, it is relatively homogeneous, with a large majority (70%) of catalogued taxa falling into the sessile category, and only one taxon assessed to be completely free moving (Figure 2). The link between mobility of a marine organism and their ability to maintain internal pCO2 is established (Knoll et al., 1996; Melzner et al., 2009; Table 1). However, as demonstrated by the results of this study, levels of mobility within some benthic invertebrates are not particularly diverse, and so may not be subject to many of the benefits theorized from high levels of exercise-induced anaerobic respiration. This could, however, be a result of the particular cross-section of taxa found for this study: almost half of which were polychaetes—annelid worms often confined within tube structures. With a wider selection of taxa, and better representation within other taxonomic groups, it is possible that a more even spread would be seen for this trait category, yielding results that are more useful in quantifying overall sensitivity. We propose that future studies based upon this general methodology would benefit from investigating a larger pool of biological traits, and incorporating a “traits estimation” stage for selected taxa, where expected traits for each selected taxon are compiled to assess diversity at an earlier stage.
Of the 11 taxonomic groups catalogued and included in wider analysis, 8 were represented by 3 or fewer taxa, with 6 of these including only one taxon each (Supplementary Appendix S1). While the array of taxa was chosen through analysing abundance data for each EUNIS habitat classification, the final group was lacking in diversity. Although this still may be representative of these habitats, it allows for only a superficial understanding of taxonomic groups other than the three most represented here. Analysis of the three most catalogued taxonomic groups found that bivalves were likely to have the highest sensitivity to increases in ocean pCO2. Knowledge that this group could be subject to more-extreme deleterious effects than other benthic groups could inform management strategies; while individual management actions at the local scale cannot mitigate widespread OA, there may be opportunities to take actions to reduce other pressures acting on the species that can be locally controlled or mitigated.
In our study dataset, a deep circalittoral muddy habitat was estimated to contain a significantly larger proportion of taxa with biological traits sensitive to increases in ocean pCO2. According to the European Environment Agency (2022), this habitat is usually dominated by polychaetes, and home to a high number of bivalves; in our dataset, the top 10 taxa were predominantly polychaetes. This prevalence of sensitive taxa gives an indication of what the future could bring for these habitats. Evidence is emerging on the importance of muddy sediments as carbon stores and of disruption to the seabed as a potential source of further CO2 emissions (Legge et al., 2020); OA impacts on sensitive species have the potential to disrupt ecological functioning in the deep muds since benthic invertebrates are important contributors to carbon cycling through bioturbation and other activities (Aller and Cochran, 2019). OA thus has the potential to alter how carbon is incorporated into or released from seabed sediments, the nature of which requires further investigation into its wider impacts.
Conclusion
We believe that the calculation of the TRS methodology shows strong potential for application in future studies of a similar nature. Clear separation of likely sensitive and non-sensitive taxa can be achieved through the selection of traits that are relatively evenly shared through selected species—an aspect for improvement in this study, which could be remedied by a “traits estimation” stage before the finalization of trait selection. Clusters of significantly higher and lower relative sensitivity were found. Knowledge of the locations of hotspots could be used to inform future conservation efforts, with the aims of managing other anthropogenic stressors that could exacerbate acidity-related impacts on the ecosystem. High overall sensitivity among catalogued taxa indicates a high risk of impact on the most abundant benthic organisms if projections of future ocean pCO2 are realized. A useful next step in the research would be to acquire a deeper view of sensitivity within different taxonomic groups in marine benthic invertebrate communities through an expansion of the number and diversity of taxa catalogued, enabling enhanced accuracy of sensitivity estimation. This methodology is well placed for performing similar analyses for different stressors, areas, and populations, which could include comparisons of locations that already experience higher levels of acidification such as upwelling zones, methane seeps, or hydrothermal vents.
Data availability
The species data underlying this article were derived from the OneBenthic tool, available at https://rconnect.cefas.co.uk/onebenthic_portal/.
Author contributions
GP carried out the traits research, data manipulation, and data analysis, with support from BJ, RC, and GC. GP lead writing the manuscript, with major contributions from BJ, and supervision from RC. The original idea and methodology for the study was conceived by BJ, with later adaptations contributed by all authors.
Conflict of interest
All authors declare that they have no conflict of interest.
ACKNOWLEDGEMENTS
The study was conducted during PG's MSc research at the University of East Anglia. The authors would like to thank Keith Cooper of Cefas for providing advice on accessing and using the OneBenthic data and the three anonymous reviewers whose comments helped to significantly improve the message and clarity of the manuscript.