-
PDF
- Split View
-
Views
-
Cite
Cite
Jaimin S Shah, Denis A Vaughan, Laura E Dodge, Angela Leung, Ann Korkidakis, Denny Sakkas, David A Ryley, Alan S Penzias, Thomas L Toth, Endometrial compaction does not predict live birth in single euploid frozen embryo transfers: a prospective study, Human Reproduction, Volume 37, Issue 5, May 2022, Pages 980–987, https://doi.org/10.1093/humrep/deac060
- Share Icon Share
Abstract
Is there a relationship between endometrial compaction and live birth in euploid frozen embryo transfer (FET) cycles?
Live birth rates (LBRs) were similar in both patients that demonstrated endometrial compaction or no compaction in single euploid FETs.
There has been increasing interest in the correlation between endometrial compaction and clinical outcomes but there has been conflicting evidence from prior investigations.
This was a prospective observational study from 1 September 2020 to 9 April 2021.
This study was performed at a single, academically affiliated fertility center in which patients who had an autologous single euploid FET using a programmed or modified natural cycle protocol were included. All embryos had trophectoderm biopsy for preimplantation genetic testing for aneuploidy followed by vitrification at the blastocyst stage. Two ultrasound measurements of endometrial thickness (EMT) were obtained. The first measurement (T1) was measured transvaginally within 1 day of initiation of progesterone or ovulation trigger injection, and a second EMT (T2) was measured transabdominally at the time of embryo transfer (ET). The primary outcome (LBR) was based on the presence and proportion of compaction (percentage difference in EMT between T1 and T2).
Of the 186 participants included, 54%, 45%, 35%, 28% and 21% of women exhibited >0%, ≥5%, ≥10%, ≥15% and ≥20% endometrial compaction, respectively. Endometrial compaction was not predictive of live birth at any of the defined cutoffs. A sub-analysis stratified by FET protocol type (n = 89 programmed; n = 97 modified natural) showed similar results.
There was the potential for measurement error in the recorded EMTs. The T2 measurement was performed transabdominally, which may cause potential measurement error, as it is generally accepted that transvaginal measurements of EMT are more accurate, though, any bias is expected to be non-differential. The sub-analysis performed looking at FET protocol type was underpowered and should be interpreted with caution. Our study, however, represents a pragmatic approach, as it allowed patients to avoid having to come in for an extra transvaginal ultrasound the day before or on the day of ET.
Assessing endometrial compaction may lead to unnecessary cycle cancellation. However, further studies are needed to determine if routine screening for endometrial compaction would improve clinical outcomes.
No authors report conflicts of interest or disclosures. There was no study funding.
NCT04330066.
Introduction
The window of implantation, defined as the period in which the endometrium is receptive to implantation of an embryo, is thought to occur between Days 20 and 24 of a 28-day menstrual cycle (Bergh and Navot, 1992). The current clinical tools used to evaluate the endometrium include analyzing endometrial tissue directly by endometrial biopsy, or through an indirect modality, such as transvaginal ultrasound, for assessing endometrial thickness (EMT) and pattern (i.e. trilaminar, echogenic) (Grunfeld et al., 1991). All these modalities have been reported to possess limitations. Prior studies assessing EMT at the end of the proliferative phase have demonstrated higher clinical pregnancy rates (CPRs) with thicker endometrial lining measurements in fresh IVF (Kovacs et al., 2003; Zhang et al., 2005; Al-Ghamdi et al., 2008; Kumbak et al., 2009; Wu et al., 2014; Zhao et al., 2014) and frozen embryo transfer (FET) cycles (El-Toukhy et al., 2008; Liu et al., 2018). However, Kasius et al. (2014) performed a systematic review and meta-analysis and reported that EMT has limited capacity for identifying women who have a low chance of conceiving after a fresh IVF cycle. Also, Shakerian et al. (2021) reported that EMT is not predictive of live birth in FET cycles. Endometrial receptivity, as defined by endometrial gene expression profiling, has also been shown to be inconsistent in studies (Simón et al., 2020; Bergin et al., 2021).
The majority of previous studies assessed the endometrium in the pre-ovulatory phase, but only a few studies have looked at EMT in the luteal phase at the time of embryo transfer (ET). No significant differences were seen in CPR (Barker et al., 2009) or ongoing pregnancy rate (OPR) (Griesinger et al., 2018) when assessing EMT and endometrial pattern at the time of ET. Furthermore, Gingold et al. (2015) reported that luteal EMT was not significantly correlated with CPR in euploid fresh or FETs.
There has been increasing interest in the correlation between endometrial compaction and clinical outcomes but the evidence from prior investigations is conflicting. Endometrial compaction is determined by the difference in EMT from the end of the estrogen-only phase to the day of ET in programmed FET cycles. Two retrospective studies from a single infertility center found positive correlations between endometrial compaction and OPR in programmed FET cycles (Haas et al., 2019; Zilberberg et al., 2020). In contrast, Bu et al. (2019) demonstrated that endometrial compaction was not correlated to CPR in programmed and natural FET cycles with untested blastocysts. Furthermore, Riestenberg et al. (2021) reported that endometrial compaction did not predict live birth rate (LBR) in programmed euploid FET cycles.
The primary objective of this prospective study was to investigate the relation between endometrial compaction and CPR in single euploid FET cycles. A secondary analysis of both programmed and modified natural FET cycles was included to determine whether there was a differential impact based on FET protocol.
Materials and methods
Patient cohort
This prospective observational study included participants 18–43 years old who were undergoing their first or second autologous single euploid FET between 1 September 2020 and 9 April 2021 at a single, academically affiliated fertility clinic. Institutional Review Board approval (2020P000191) was obtained from the Committee on Clinical Investigations at Beth Israel Deaconess Medical Center. This study was registered with Clinicaltrials.gov (NCT04330066). We excluded patients with an EMT <7 mm, BMI >40 or <18.5 kg/m2, those using a gestational carrier, those with a history of ≥2 spontaneous abortions, those with current or prior uterine factors (i.e. polyps, fibroids, adhesions), and those with a prior endometrial receptivity analysis biopsy (Igenomix, Valencia, Spain).
Frozen embryo transfer
Decisions on ovarian stimulation and FET protocols were left to the discretion of the treating physician. Oocyte retrieval was performed 36 h after the trigger injection with either hCG, leuprolide acetate or a combination of both. Our clinic routinely performs standard insemination for fertilization unless ICSI is indicated. Laser-assisted hatching is routinely performed on Day 3 of embryo development in cycles with planned preimplantation genetic testing for aneuploidy (PGT-A). All embryos underwent trophectoderm biopsy at the blastocyst stage followed by vitrification. Only blastocysts reaching 3BB or better, using the Schoolcraft and Gardner criteria (Schoolcraft et al., 1999), were biopsied and frozen. All blastocysts were cryopreserved on either Day 5 or Day 6 of culture and PGT-A was performed using next-generation sequencing.
The research team reviewed the appointment log and medical records in order to identify patients who were eligible, per the study inclusion and exclusion criteria, prior to or during the start of the embryo thaw cycle. Eligible participants were approached by the research team on the day of ET, and informed verbal consent was obtained prior to the ET.
Two groups of thaw cycles were included: programmed hormone replacement thaw cycles (i.e. utilizing both exogenous estrogen and progesterone); and modified natural thaw cycles. For programmed thaw cycles, participants started oral estradiol 3 mg twice daily either on cycle day one or within 3 days of stopping oral contraceptive pills. Participants continued estradiol for a minimum of 14–18 days and then underwent a transvaginal ultrasound for EMT measurement and assessment of serum progesterone level. If the EMT was <7 mm, supplemental estrogen was added per physician discretion. Once the EMT was ≥7 mm and serum progesterone was <1.2 ng/ml, progesterone supplementation was initiated the following day using either daily progesterone in oil (50 mg i.m. daily) or progesterone in oil (50 mg) every third day with daily vaginal progesterone (Crinone® 90 mg twice daily [Merck KGaA, Darmstadt, Germany] or Endometrin® 200 mg twice daily [Ferring Pharmaceuticals, Saint-Prex, Switzerland]). The day of progesterone initiation was considered P + 0. Participants returned for their FET on P + 5 (∼120 h after progesterone initiation). Natural thaw cycles were considered ‘modified’ by the use of hCG for trigger injection (Ovidrel® 250 µg, EMD Serono/Merck, Darmstadt, Germany), the use of supplemental vaginal progesterone (Crinone® 90 mg daily or Endometrin® 100 mg twice daily), and/or the use of oral letrozole for ovarian stimulation in the early follicular phase. Participants underwent a transvaginal ultrasound and serum hormone evaluation on cycle Day 10. Once the EMT was ≥7 mm and a lead follicle was ≥18 mm, an hCG trigger injection (Ovidrel® 250 µg) was administered. The day of hCG trigger was considered Day 0, and participants returned for their FET on hCG + 7 if on the day of hCG trigger their LH was <20 mIU/ml and progesterone was <1.2 ng/ml. If LH was >20 mIU/ml and progesterone was >1.2 ng/ml, participants returned for their FET on hCG + 6. Starting 3 days before the FET, all participants initiated supplemental vaginal progesterone.
Ultrasound measurements
Transvaginal ultrasound measurements of the EMT (T1) were required to be within 1 day of the initiation of progesterone (programmed thaw cycles) or ovulation trigger injection (modified natural thaw cycles). Measurements of the EMT at T1 included a longitudinal view of the endometrium and cervix. The second EMT (T2) was measured transabdominally at the time of ET. All T1 EMT measurements were reviewed by a single physician (J.S.S.) from the research team prior to participant enrollment to minimize bias, and participants were excluded from the study if the EMT measurement was deemed inaccurate (i.e. calipers not appropriately aligned at the edge of the endometrium or measurement was not perpendicular to the endometrium axis). Endometrial pattern (i.e., trilaminar or echogenic) at T1 was recorded. All T2 images were screened by the physician performing the ET at the time of ET to ensure that the recorded EMT measurements were accurate. These physicians and sonographers were blinded to the T1 measurement. Endometrial compaction was calculated as the percentage difference in EMT between T1 and T2 ([T1 − T2]/T1). Measurements from the T2 ultrasound were not shared with the participant, and they were not used to make any clinical decisions.
The primary outcome was LBR (defined as live birth ≥23 weeks gestation). Secondary outcomes included CPR (defined as the presence of at least one gestational sac with a fetal pole with cardiac activity on transvaginal ultrasound between 6 and 9 weeks gestation), biochemical pregnancy rate and spontaneous abortion rate. Patients were discharged to their obstetrician when a clinical pregnancy was confirmed between 6 and 9 weeks gestation. Baseline demographic data were obtained from the electronic medical record (Practice Highways, Irving, TX, USA) and included age, race, height, weight, gravidity and parity. Clinical data were obtained from the electronic medical record and included infertility diagnosis, reason for PGT-A, prior outcome of a fresh ET and prior outcome of a FET. The reason for PGT-A was reported by the treating physician in the electronic medical record. All data were collected and stored in REDCap™ (a secure HIPAA-compliant data storage software) (Harris et al., 2009, 2019).
Sample size and statistical analysis
Our a priori sample size calculation was based on data from a prior study that evaluated compaction and correlations with OPR (Zilberberg et al., 2020). In order to detect a difference of at least 10% in LBR between the compaction and non-compaction groups using a two-sided alpha of 0.05, at least 82 participants in each group were needed, for a total of 164 participants.
Descriptive data are presented as the mean with SD or counts with percentages. LBR and CPR were compared between those with and without compaction at various cutoffs (>0%, ≥5%, ≥10%, ≥15% and ≥20%) using a chi-square test, and we calculated sensitivity, specificity and positive and negative predictive values at each cutoff for the entire cohort and for a sub-analysis by FET protocol type. A binary logistic regression was performed to calculate unadjusted and adjusted risk ratios (RRs) and 95% CI for the risk of live birth by endometrial compaction. Adjusted variables included: maternal age at FET cycle start, BMI, gravidity, parity and infertility diagnosis. An F-test was used to compare trends between initial EMT and percentage compaction. We considered P-values <0.05 to be statistically significant. All data were analyzed with SAS 9.4 (SAS Institute Inc., Cary, NC, USA) and GraphPad Prism for Windows (GraphPad Software, La Jolla, CA, USA).
Results
There were 202 eligible participants with 186 enrolled participants who underwent FET cycles (n = 89 programmed; n = 97 modified natural) with single euploid ETs (Supplementary Fig. S1). Participants on average were 36 years old, had a BMI of 25.8 kg/m2, were mostly nulligravid or nulliparous, and were predominately White (Table I). Most participants were undergoing their first FET (74.2%) and only 10.8% of patients had a prior fresh ET. Most participants (86%) had a trilaminar appearance of the endometrium at T1. The mean EMT measurements in the programmed and modified natural thaw cycles were 10.2 ± 2.3 mm and 9.5 ± 1.7 mm, respectively. For the entire cohort, the mean LBR was 55.9%, CPR was 57%, biochemical pregnancy rate was 8.6% and the spontaneous abortion rate was 2.7%. All clinical pregnancies were single intrauterine pregnancies except for two patients that had twins.
Patient characteristics . | n = 186 . |
---|---|
Age (years) | 36.0 ± 3.6 |
BMI (kg/m2) | 25.8 ± 4.2 |
Nulligravid | 96 (51.6) |
Nulliparous | 122 (65.6) |
Race | |
White | 155 (83.3) |
Asian | 24 (12.9) |
Black or African American | 7 (3.8) |
Hispanic ethnicity | 9 (4.8) |
Cause of infertility* | |
Male factor | 41 (22.0) |
Ovulatory dysfunction | 31 (16.7) |
Female factor | 29 (15.6) |
Unexplained | 76 (40.9) |
Other | 25 (13.4) |
Reason for PGT-A* | |
Elective | 99 (53.2) |
Advanced reproductive age (≥38 years) | 66 (35.5) |
PGT-M‡ | 19 (10.2) |
PGT-SR | 2 (1.1) |
Insemination | |
Conventional | 92 (49.5) |
ICSI | 94 (50.5) |
Prior fresh embryo transfer | 20 (10.8) |
Negative pregnancy test | 7 (35.0) |
Biochemical pregnancy | 3 (15.0) |
Spontaneous abortion | 0 (0) |
Live birth | 10 (50.0) |
Prior single FET | 48 (25.8) |
Negative pregnancy test | 12 (25.0) |
Biochemical pregnancy | 3 (6.3) |
Spontaneous abortion | 4 (8.3) |
Live birth | 29 (60.4) |
Patient characteristics . | n = 186 . |
---|---|
Age (years) | 36.0 ± 3.6 |
BMI (kg/m2) | 25.8 ± 4.2 |
Nulligravid | 96 (51.6) |
Nulliparous | 122 (65.6) |
Race | |
White | 155 (83.3) |
Asian | 24 (12.9) |
Black or African American | 7 (3.8) |
Hispanic ethnicity | 9 (4.8) |
Cause of infertility* | |
Male factor | 41 (22.0) |
Ovulatory dysfunction | 31 (16.7) |
Female factor | 29 (15.6) |
Unexplained | 76 (40.9) |
Other | 25 (13.4) |
Reason for PGT-A* | |
Elective | 99 (53.2) |
Advanced reproductive age (≥38 years) | 66 (35.5) |
PGT-M‡ | 19 (10.2) |
PGT-SR | 2 (1.1) |
Insemination | |
Conventional | 92 (49.5) |
ICSI | 94 (50.5) |
Prior fresh embryo transfer | 20 (10.8) |
Negative pregnancy test | 7 (35.0) |
Biochemical pregnancy | 3 (15.0) |
Spontaneous abortion | 0 (0) |
Live birth | 10 (50.0) |
Prior single FET | 48 (25.8) |
Negative pregnancy test | 12 (25.0) |
Biochemical pregnancy | 3 (6.3) |
Spontaneous abortion | 4 (8.3) |
Live birth | 29 (60.4) |
Cycle characteristics . | |
---|---|
FET protocol | |
Programmed | 89 (47.9) |
Modified natural | 97 (52.2) |
Letrozole | 40 (41.2) |
No Letrozole | 57 (58.8) |
Endometrial lining† | |
Trilaminar | 160 (86.0) |
Echogenic | 26 (14.0) |
Mean endometrial lining measurement (mm) | |
Programmed | 10.2 ± 2.3 |
Modified natural | 9.5 ± 1.7 |
Letrozole | 8.8 ± 1.3 |
No Letrozole | 9.9 ± 1.7 |
Cycle characteristics . | |
---|---|
FET protocol | |
Programmed | 89 (47.9) |
Modified natural | 97 (52.2) |
Letrozole | 40 (41.2) |
No Letrozole | 57 (58.8) |
Endometrial lining† | |
Trilaminar | 160 (86.0) |
Echogenic | 26 (14.0) |
Mean endometrial lining measurement (mm) | |
Programmed | 10.2 ± 2.3 |
Modified natural | 9.5 ± 1.7 |
Letrozole | 8.8 ± 1.3 |
No Letrozole | 9.9 ± 1.7 |
Data are shown as mean ± SD or n (%).
FET, frozen embryo transfer; PGT-A, preimplantation genetic testing for aneuploidy; PGT-M, preimplantation genetic testing for monogenic disorders; PGT-SR, preimplantation genetic testing for structural chromosomal rearrangements.
Can have more than one selection, and thus causes sum to be more than 100%.
Patients who had PGT-M, had PGT-A first.
Transvaginal ultrasound at time of hCG trigger or start of progesterone.
Patient characteristics . | n = 186 . |
---|---|
Age (years) | 36.0 ± 3.6 |
BMI (kg/m2) | 25.8 ± 4.2 |
Nulligravid | 96 (51.6) |
Nulliparous | 122 (65.6) |
Race | |
White | 155 (83.3) |
Asian | 24 (12.9) |
Black or African American | 7 (3.8) |
Hispanic ethnicity | 9 (4.8) |
Cause of infertility* | |
Male factor | 41 (22.0) |
Ovulatory dysfunction | 31 (16.7) |
Female factor | 29 (15.6) |
Unexplained | 76 (40.9) |
Other | 25 (13.4) |
Reason for PGT-A* | |
Elective | 99 (53.2) |
Advanced reproductive age (≥38 years) | 66 (35.5) |
PGT-M‡ | 19 (10.2) |
PGT-SR | 2 (1.1) |
Insemination | |
Conventional | 92 (49.5) |
ICSI | 94 (50.5) |
Prior fresh embryo transfer | 20 (10.8) |
Negative pregnancy test | 7 (35.0) |
Biochemical pregnancy | 3 (15.0) |
Spontaneous abortion | 0 (0) |
Live birth | 10 (50.0) |
Prior single FET | 48 (25.8) |
Negative pregnancy test | 12 (25.0) |
Biochemical pregnancy | 3 (6.3) |
Spontaneous abortion | 4 (8.3) |
Live birth | 29 (60.4) |
Patient characteristics . | n = 186 . |
---|---|
Age (years) | 36.0 ± 3.6 |
BMI (kg/m2) | 25.8 ± 4.2 |
Nulligravid | 96 (51.6) |
Nulliparous | 122 (65.6) |
Race | |
White | 155 (83.3) |
Asian | 24 (12.9) |
Black or African American | 7 (3.8) |
Hispanic ethnicity | 9 (4.8) |
Cause of infertility* | |
Male factor | 41 (22.0) |
Ovulatory dysfunction | 31 (16.7) |
Female factor | 29 (15.6) |
Unexplained | 76 (40.9) |
Other | 25 (13.4) |
Reason for PGT-A* | |
Elective | 99 (53.2) |
Advanced reproductive age (≥38 years) | 66 (35.5) |
PGT-M‡ | 19 (10.2) |
PGT-SR | 2 (1.1) |
Insemination | |
Conventional | 92 (49.5) |
ICSI | 94 (50.5) |
Prior fresh embryo transfer | 20 (10.8) |
Negative pregnancy test | 7 (35.0) |
Biochemical pregnancy | 3 (15.0) |
Spontaneous abortion | 0 (0) |
Live birth | 10 (50.0) |
Prior single FET | 48 (25.8) |
Negative pregnancy test | 12 (25.0) |
Biochemical pregnancy | 3 (6.3) |
Spontaneous abortion | 4 (8.3) |
Live birth | 29 (60.4) |
Cycle characteristics . | |
---|---|
FET protocol | |
Programmed | 89 (47.9) |
Modified natural | 97 (52.2) |
Letrozole | 40 (41.2) |
No Letrozole | 57 (58.8) |
Endometrial lining† | |
Trilaminar | 160 (86.0) |
Echogenic | 26 (14.0) |
Mean endometrial lining measurement (mm) | |
Programmed | 10.2 ± 2.3 |
Modified natural | 9.5 ± 1.7 |
Letrozole | 8.8 ± 1.3 |
No Letrozole | 9.9 ± 1.7 |
Cycle characteristics . | |
---|---|
FET protocol | |
Programmed | 89 (47.9) |
Modified natural | 97 (52.2) |
Letrozole | 40 (41.2) |
No Letrozole | 57 (58.8) |
Endometrial lining† | |
Trilaminar | 160 (86.0) |
Echogenic | 26 (14.0) |
Mean endometrial lining measurement (mm) | |
Programmed | 10.2 ± 2.3 |
Modified natural | 9.5 ± 1.7 |
Letrozole | 8.8 ± 1.3 |
No Letrozole | 9.9 ± 1.7 |
Data are shown as mean ± SD or n (%).
FET, frozen embryo transfer; PGT-A, preimplantation genetic testing for aneuploidy; PGT-M, preimplantation genetic testing for monogenic disorders; PGT-SR, preimplantation genetic testing for structural chromosomal rearrangements.
Can have more than one selection, and thus causes sum to be more than 100%.
Patients who had PGT-M, had PGT-A first.
Transvaginal ultrasound at time of hCG trigger or start of progesterone.
For programmed FET cycles, participants on average were taking oral estrogen for 18.3 ± 3.2 days prior to starting progesterone (Supplementary Table SI). In programmed FET cycles, 54% and 46% of patients were using daily i.m. progesterone only or i.m. progesterone every third day with daily vaginal progesterone, respectively (Supplementary Table SI). In modified natural FET cycles, 41.2% of patients took oral letrozole (5 mg daily) between cycle Days 3 and 7 (Supplementary Table SI).
For the entire cohort (n = 186), 54%, 45%, 35%, 28% and 21% of patients had >0%, ≥5%, ≥10%, ≥15% and ≥20% compaction, respectively (Supplementary Table SII). One percent and 45% of participants had no change or had expansion in the EMT, respectively.
Analyses of the data show there were no significant differences in the LBR (57.5 versus 46.2%, P = 0.28), CPR (58.8 versus 46.2%, P = 0.23), biochemical pregnancy rate (8.8 versus 11.5%, P = 0.65) or spontaneous abortion rate (3.8 versus 3.9%, P = 0.98) when comparing participants with trilaminar (n = 160) versus echogenic (n = 26) endometrial pattern at T1, respectively. There were no significant differences in the LBR (52.9 versus 58.4%, P = 0.45), CPR (55.3 versus 58.4%, P = 0.67), biochemical pregnancy rate (11.8 versus 6.9%, P = 0.25) or spontaneous abortion rate (3.5 versus 4.0%, P = 0.88) when comparing participants who had no compaction (n = 85) versus endometrial compaction (n = 101, >0%), respectively. When comparing programmed (n = 89) versus modified natural (n = 97) FETs, there were no differences in the biochemical pregnancy rate (11.2 versus 6.2%, P = 0.22) or spontaneous abortion rate (4.5 versus 1.0%, P = 0.20), however, there were significant differences in LBR (46.1% versus 65%, P = 0.01) and CPR (48.3 versus 65%, P = 0.02), respectively. When subdividing modified natural thaws to letrozole (n = 40) versus no letrozole (n = 57), there were no significant differences in LBR (55 versus 71.9%, P = 0.09), CPR (55 versus 71.9%, P = 0.09), biochemical pregnancy rate (5.0 versus 7.0%, P = 1.00) or spontaneous abortion rate (0 versus 1.8%, P = 1.00), respectively.
Figure 1 shows that endometrial compaction was not predictive of live birth at any of the defined cutoffs when comparing the compacted versus non-compacted group, as the LBR was similar between groups. Similar findings were seen when evaluating clinical pregnancy (Supplementary Table SII). In a sub-analysis stratified by programmed and modified natural FET cycles, endometrial compaction was similarly not predictive of live birth at any of the defined cutoffs (Supplementary Table SIII). Further sub-analysis was performed by stratifying the modified natural thaws into letrozole or no letrozole, which demonstrated that endometrial compaction was not predictive of live birth at any of the defined cutoffs (Supplementary Table SIV).
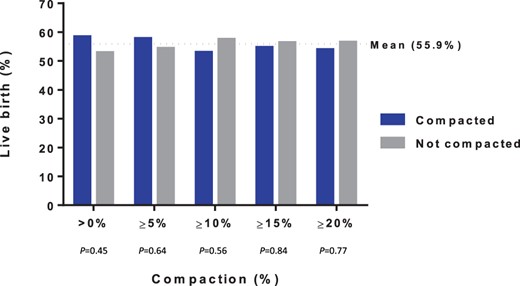
Live birth by percentage compaction among all participants (n = 186).
When performing the logistic regression, there was no increased risk of live birth between the no compaction versus compaction groups (Table II). However, the modified natural versus the programmed thaw group had an increased risk of live birth (adjusted RR 1.38, 95% CI 1.03, 1.85) (Table II). Furthermore, when analyzing letrozole versus no letrozole modified natural thaws, there was no increased risk of live birth between groups (Table II).
Logistic regression analysis for live birth, by endometrial compaction and protocol group.
Patient characteristics . | Unadjusted . | Adjusted* . |
---|---|---|
Endometrial change | ||
No compaction** | Reference | Reference |
Compaction | 1.10 (0.85–1.43) | 1.12 (0.86–1.44) |
FET protocol | ||
Programmed | Reference | Reference |
Modified natural | 1.41 (1.08–1.84) | 1.38 (1.03–1.85) |
Modified natural protocol† | ||
Letrozole | Reference | Reference |
No Letrozole | 1.31 (0.95–1.81) | 1.16 (0.91–1.48) |
Patient characteristics . | Unadjusted . | Adjusted* . |
---|---|---|
Endometrial change | ||
No compaction** | Reference | Reference |
Compaction | 1.10 (0.85–1.43) | 1.12 (0.86–1.44) |
FET protocol | ||
Programmed | Reference | Reference |
Modified natural | 1.41 (1.08–1.84) | 1.38 (1.03–1.85) |
Modified natural protocol† | ||
Letrozole | Reference | Reference |
No Letrozole | 1.31 (0.95–1.81) | 1.16 (0.91–1.48) |
Unadjusted and adjusted risk ratios and 95% CIs are presented.
FET, frozen embryo transfer.
Adjusted for age, BMI, gravidity, parity and infertility diagnosis (male factor, female factor, ovulatory dysfunction, unexplained and other).
No compaction includes patients with no change in endometrial thickness and patients with endometrial expansion.
Adjusted model did not include ovulatory dysfunction and other owing to small numbers.
Logistic regression analysis for live birth, by endometrial compaction and protocol group.
Patient characteristics . | Unadjusted . | Adjusted* . |
---|---|---|
Endometrial change | ||
No compaction** | Reference | Reference |
Compaction | 1.10 (0.85–1.43) | 1.12 (0.86–1.44) |
FET protocol | ||
Programmed | Reference | Reference |
Modified natural | 1.41 (1.08–1.84) | 1.38 (1.03–1.85) |
Modified natural protocol† | ||
Letrozole | Reference | Reference |
No Letrozole | 1.31 (0.95–1.81) | 1.16 (0.91–1.48) |
Patient characteristics . | Unadjusted . | Adjusted* . |
---|---|---|
Endometrial change | ||
No compaction** | Reference | Reference |
Compaction | 1.10 (0.85–1.43) | 1.12 (0.86–1.44) |
FET protocol | ||
Programmed | Reference | Reference |
Modified natural | 1.41 (1.08–1.84) | 1.38 (1.03–1.85) |
Modified natural protocol† | ||
Letrozole | Reference | Reference |
No Letrozole | 1.31 (0.95–1.81) | 1.16 (0.91–1.48) |
Unadjusted and adjusted risk ratios and 95% CIs are presented.
FET, frozen embryo transfer.
Adjusted for age, BMI, gravidity, parity and infertility diagnosis (male factor, female factor, ovulatory dysfunction, unexplained and other).
No compaction includes patients with no change in endometrial thickness and patients with endometrial expansion.
Adjusted model did not include ovulatory dysfunction and other owing to small numbers.
There was a significant positive correlation between initial EMT and percentage of participants with endometrial compaction of at least 10% (P = 0.02) but a non-significant correlation when looking at participants with endometrial compaction of at least 5% (P = 0.24) (Fig. 2). No pattern was visualized when plotting the clinical outcome per participant based on initial EMT and endometrial compaction of participants with at least 10% and 5% compaction (Supplementary Fig. S2).
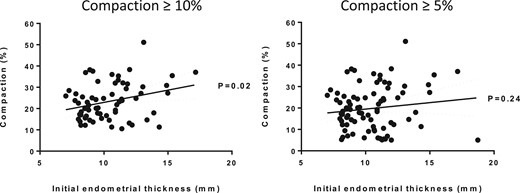
Correlation between initial endometrial thickness and endometrial compaction.Left panel: Participants (n = 66) with at least 10% endometrial compaction. Right panel: Participants (n = 83) with at least 5% endometrial compaction.
Discussion
In this prospective study, endometrial compaction was not found to be predictive of live birth in the overall cohort or in the sub-analysis evaluating correlations with a single euploid FET protocol type. To date, four prior studies have reported conflicting data regarding the association between endometrial compaction and CPR (Bu et al., 2019), OPR (Haas et al., 2019; Zilberberg et al., 2020) and LBR (Riestenberg et al., 2021).
Endometrial physiology changes in relation to the different stages of endometrial development in the proliferative and secretory phases. In the proliferative phase, the endometrium is exposed to high estrogen levels that stimulate growth of endometrial glands and blood vessels leading to increasing EMT and a multilayered appearance on ultrasound (Haas et al., 2019). Endometrial proliferation ends ∼3 days after ovulation in association with rising progesterone levels (Tabibzadeh, 1990). EMT is generally fixed at this time, and further vascular and glandular development increases endometrial density rather than volume, giving the secretory endometrium a homogenous hyperechoic appearance on ultrasound (Riestenberg et al., 2021). Continued endometrial growth in the secretory phase may be suggestive of progesterone resistance and potentially creates a suboptimal environment for implantation (Riestenberg et al., 2021). Although further investigation is needed on progesterone resistance, potential etiologic factors contributing to progesterone resistance have been proposed including overexpression of B-cell lymphoma 6 and Sirtuin 1, chronic endometrial inflammation, progesterone receptor gene polymorphisms, altered microRNA expression and epigenetic modifications to the progesterone receptor (Patel et al., 2017; Yoo et al., 2017; Hu et al., 2018; Haas et al., 2019).
In the retrospective study by Haas et al. (2019), the investigators included a combination of patients with euploid (23%) and untested blastocysts (77%) and found higher OPRs in patients with a programmed single embryo FET cycle with ≥5% up to ≥15% endometrial compaction. Haas et al. (2019) reported 42% and 31% of patients with 5% and 10% compaction, respectively, which was similar to our study with 45% and 35% of patients with 5% and 10% compaction, respectively. Haas et al. (2019) also demonstrated that patients with an initial EMT of <8 mm had a lower percentage of 10% compaction compared to patients with an initial EMT of >8 mm or >11 mm. Similarly, in our study, we demonstrated that as the initial EMT increased there was a positive correlation to the percentage of compaction >10%. We speculate that patients with a lower initial EMT with less compaction may have an altered estrogen-progesterone ratio, causing less compaction (Haas et al., 2019), however further research is needed. In a subsequent retrospective study from the same group by Zilberberg et al. (2020), where only Day 5 or Day 6 euploid embryos were included (n = 225), higher OPRs in patients were reported with a programmed single embryo FET cycle when 5–20% endometrial compaction was noted. Similarly, Zilberberg et al. (2020) found 43% and 38% of patients with 5% and 10% compaction, respectively. As with our study, an abdominal ultrasound was utilized at the time of ET for EMT evaluation in all of the aforementioned investigations. However, Zilberberg et al. (2020) included patients with recurrent pregnancy loss and recurrent implantation failure, and these patients were excluded in our study to create a more homogenous cohort.
In contrast to the prior two studies, Bu et al. (2019) evaluated programmed and natural single embryo (high quality, untested) FET cycles and did not show a correlation between endometrial compaction and CPR but actually found that endometrial expansion was correlated with higher CPR. Bu et al. (2019) reported 22% of patients with 5% endometrial compaction, which was much lower than our study (45%). The investigators utilized a transvaginal ultrasound on the day of ET to record the EMT. Finally, Riestenberg et al. (2021) included 259 patients who had a single euploid FET in a programmed thaw cycle and reported that there was no difference in LBR for patients with compaction, expansion or in those without a change in EMT. Riestenberg et al. (2021) reported 16.6% of patients with 5% endometrial compaction, which was much lower than our study (45%). Of note, the investigators evaluated EMT by transvaginal ultrasound the day before the ET rather than on the day of ET.
Other studies have found conflicting evidence regarding correlations between FET clinical outcomes and EMT and endometrial pattern. Studies have shown that an EMT of <7 mm is associated with a lower CPR and LBR (Liu et al., 2018) and an EMT of >8 mm is associated with higher CPRs (Shalom-Paz et al., 2021). Also, El-Toukhy et al. (2008) reported that an EMT of 9–14 mm is associated with higher CPR and LBRs. In contrast, other studies have reported that EMT is not predictive of LBR (Yang et al., 2018; Shakerian et al., 2021) or CPR (Gingold et al., 2015). When assessing endometrial pattern, a prior study reported that a mid-late secretory (homogeneous hyperechoic functionalis extending from the basalis to the lumen) endometrial pattern was associated with a lower implantation rate (Gingold et al., 2015), while another study showed that an endometrial trilaminar pattern was positively associated with CPR but not LBR or spontaneous abortion rate (Yang et al., 2018).
Our study has several notable strengths. We collected data prospectively, and our study was appropriately powered to detect the specified difference in the primary outcome, LBR. Additionally, we included both programmed and modified natural FET protocol types, which adds to the limited body of literature regarding natural FETs. We included only single euploid blastocyst transfers to limit any confounding factors related to aneuploidy status. Our strict inclusion criteria eliminated other uterine factors that may have contributed to the clinical outcome. Furthermore, a majority of patients were having their first FET (74%) and did not have a prior fresh ET (89%), which limited heterogeneity.
Several limitations, however, need to be acknowledged. Although experienced sonographers performed ultrasounds at T1 and T2, there is the potential for measurement error in the recorded EMTs. While the second EMT was recorded in real time by various physicians who were performing the ET, this might introduce inter-observer variability. The T2 measurement was performed transabdominally, which may cause potential measurement error, as it is generally accepted that transvaginal measurements of EMT are more accurate, although any bias is expected to be non-differential. The sub-analysis performed looking at FET protocol type was underpowered and should be interpreted with caution. Different luteal progesterone protocols are used at our fertility center for both programmed and modified natural thaw cycles. However, a prior study showed progesterone in oil (50 mg) every third day with daily vaginal progesterone had non-inferior LBRs to daily progesterone in oil (50 mg i.m.) in programmed FET cycles (Devine et al., 2021). The different vaginal progesterone protocols in modified natural thaw cycles used at our clinic are dictated by insurance requirements, however, this should have limited impact on clinical outcomes given the endogenous luteal support. The inclusion of only euploid blastocyst transfers may create a more homogeneous set of embryos, while the status of endometrial compaction may be more important with blastocysts of more heterogeneous quality. Our study, however, represents a pragmatic approach, as it allowed patients to avoid having to come in for an extra transvaginal ultrasound the day before or on the day of ET.
The LBRs were similar in participants that demonstrated endometrial compaction or no compaction. Assessing endometrial compaction may lead to unnecessary cycle cancellation; however, future studies are needed to determine if routine screening for endometrial compaction would improve clinical outcomes.
Data availability
The data underlying this article are available in the article and in its online supplementary material.
Acknowledgements
The authors would like to acknowledge: Michelle Merrick, Luciana Scimemi and Jamie Sokolski for consenting participants and performing study ultrasound measurements; Sonia Cruz, Nicole Fugere, Teresa Bell and Vicky Gresham for consenting participants and assisting in study logistics; Brian French for assisting with logistics and paperwork; and all Boston IVF physicians who assisted with this study.
Authors’ roles
All authors contributed to the conceptualization of the idea and experiment, critical revision of the manuscript, data interpretation and approved the final draft of the manuscript. J.S.S. screened eligible participants. L.E.D. performed the analysis and J.S.S. drafted the manuscript.
Funding
No funding was obtained.
Conflict of interest
No conflicts of interest exist for any author.
References
Author notes
Present address for Angela Leung: Reproductive Medicine Associates (RMA) of New Jersey, Basking Ridge, NJ, USA