-
PDF
- Split View
-
Views
-
Cite
Cite
Y Zhou, S Richard, N J Batchelor, D E Oorschot, G M Anderson, M W Pankhurst, Anti-Müllerian hormone-mediated preantral follicle atresia is a key determinant of antral follicle count in mice, Human Reproduction, Volume 37, Issue 11, November 2022, Pages 2635–2645, https://doi.org/10.1093/humrep/deac204
- Share Icon Share
Abstract
Does anti-Müllerian hormone (AMH) induce preantral follicle atresia in mice?
The present findings suggest that AMH-mediated follicle atresia only occurs in early follicles before they become sensitive to FSH.
Most prior studies have investigated the ability of AMH to inhibit primordial follicle activation. Our previous study showed that AMH-overexpressing mice had fewer preantral follicles than expected after accounting for primordial follicle inhibition but the reason for this was not determined.
Cross-sectional—control versus transgenic/knockout mouse studies were carried out.
Studies were conducted on female wild-type (Amh+/+), AMH-knockout (Amh−/−) and AMH overexpressing (Thy1.2-AMHTg/0) mice on a C57Bl/6J background (age: 42–120 days). The follicle counts were conducted for primordial, transitioning, primary, secondary and antral follicles in Amh−/− and Amh+/+ mice. After confirming that follicle development speeds were identical (proliferating cell nuclear antigen immunohistochemistry), the ratio of follicles surviving beyond each stage of folliculogenesis was determined in both genotypes. Evidence for increased rates of preantral follicle atresia was assessed by active caspase-3 immunohistochemistry in wild-type and Thy1.2-AMHTg/0 mice.
Amh−/− mice at 100–120 days of age had lower primordial follicle counts but higher primordial follicle activation rates compared to Amh+/+ mice. These counteracting effects led to equivalent numbers of primordial follicles transitioning to the primary stage in Amh+/+ and Amh−/− mice. Despite this, Amh+/+ mice had fewer primary, secondary, small antral and medium antral follicles than Amh−/− mice indicating differing rates of developing follicle atresia between genotypes. Cleaved caspase-3 immunohistochemistry in Thy1.2-AMHTg/0 ovaries revealed high rates of granulosa cell and oocyte apoptosis in late primary/early secondary follicles of Thy1.2-AMHTg/0 mice.
N/A.
The findings were shown only in one species and additional research will be required to determine generalizability to other species.
This study is consistent with prior studies showing that Amh−/− mice have increased primordial follicle activation but these new findings demonstrate that AMH-mediated preantral follicle atresia is a predominant cause of the increased small antral follicle counts in Amh−/− mice. This suggests that the role of AMH is not to conserve the ovarian reserve to prolong fertility, but instead to prevent the antral follicle pool from becoming too large. While this study may demonstrate a new function for AMH, the biological purpose of this function requires further investigation, particularly in mono-ovulatory species.
This study was funded by the Health Research Council of New Zealand and the University of Otago. No competing interests to declare.
Introduction
Anti-Müllerian hormone (AMH) is secreted by the granulosa cells of non-atretic, developing ovarian follicles. The secreted AMH acts back on these cells in an autocrine manner to influence sensitivity to FSH and attenuate aromatase synthesis (Grossman et al., 2008; Pellatt et al., 2011; Campbell et al., 2012). AMH also influences follicle survival and growth, in a stage-specific manner (Xu et al., 2016). AMH diffuses out of developing follicles to act on the pre-granulosa cells of primordial follicles to inhibit activation and transition to the primary follicle stage (Durlinger et al., 2002). This action is paracrine, as pre-granulosa cells on primordial follicles express the AMH type 2 receptor (Kano et al., 2017) but do not synthesize AMH while the follicle lies dormant (Baarends et al., 1995; Weenen et al., 2004).
The AMH-mediated inhibition of primordial follicle activation was first demonstrated in Amh−/− mice, which exhibit a more rapid age-related decline in primordial follicle reserves than Amh+/+ females (Durlinger et al., 1999). This effect has been confirmed in multiple species in vitro by administering recombinant AMH to ovary explant or whole-organ cultures (Durlinger et al., 2002; Gigli et al., 2005; Carlsson et al., 2006; Nilsson et al., 2007, 2014; Rocha et al., 2016; Yang et al., 2017). The increased primordial follicle activation rates in Amh−/− mice lead to a larger developing follicle pool, which is presumed to occur due to a greater rate of primordial follicle activation (Durlinger et al., 1999). However, by the preovulatory follicle stage, the follicle counts have equalized in unstimulated Amh−/− and Amh+/+ mice (Visser et al., 2007) and both genotypes experience similar fecundity across their reproductive lifespan (Guo and Pankhurst, 2020). By contrast, gonadotropin stimulation yields higher ovulation rates in young Amh−/− females and lower ovulation rates in ageing Amh−/− females relative to age-matched Amh+/+ females (Visser et al., 2007). This suggests that negative feedback in the hypothalamic–pituitary–gonadal axis and follicle atresia are responsible for maintaining normal ovulation rates in Amh−/− females, despite the larger size of their developing follicle pool.
Recent attempts to establish correlations between serum AMH levels and primordial follicle density in human ovary biopsies have failed to find strong associations between the two variables (Liebenthron et al., 2019; Sermondade et al., 2019; von Wolff et al., 2020), which questions whether AMH levels have a substantial impact on primordial follicle numbers. AMH-overexpressing mice do exhibit a reduction in numbers of primary follicles, but also a second proportionately larger reduction in secondary follicles (Pankhurst et al., 2018). Long-term recombinant AMH administration in mice reduces primary follicle numbers by 40–60%, yet no follicles progress to the secondary stage under these conditions (Kano et al., 2017, 2019). Collectively, this suggests that AMH has a more potent effect on small follicle atresia than primordial follicle activation. Owing to the prolonged duration of primary follicle development in humans and mice (Hoage and Cameron, 1976; Hirshfield, 1989; Chen et al., 2021), even very low rates of atresia per day could lead to large cumulative follicle losses over time. Our initial estimates predicted that observations of intact atretic preantral follicles would be extremely rare. To circumvent this issue, we compared preantral follicle survival rates in Amh+/+ and Amh−/− mice. The Amh−/− females were hypothesized to have higher rates of primary and secondary follicles surviving to subsequent stages of folliculogenesis compared to Amh+/+ mice. Cleaved caspase-3 immunohistochemistry was examined in AMH-overexpressing mouse ovaries, as these mice were predicted to provide the greatest chance to observed direct evidence of AMH-mediated preantral follicle atresia.
Materials and methods
Animals
The Amh−/− mice were generated by targeted gene disruption (Behringer et al., 1994). The transgenic mutants were maintained by paring heterozygous (Amh+/−) male and female mice maintained on a C57Bl6/J line. Genotyping was conducted by PCR using a common forward primer (5′- GGAACACAAGCAGAGCTTCC-3′) and one reverse primer directed to wild-type Amh gene (5′-GAGACAGAGTCCATCACGTAC-3′) and a second reverse primer directed to the mutant Amh gene (5′-TCGTGCTTTACGGTATCGC-3′). The Amh−/− and Amh+/+ mice used in the histological follicle-counting experiments were between 100 and 120 days of age. The age range of the mice used in the immunohistochemistry staining of proliferating cell nuclear antigen (PCNA) was slightly broader at 42–120 days of age but, in a reproductive context, this corresponds to the same phase of life as 18- to 25-year-old humans, hence substantial variations in the process of folliculogenesis were not anticipated.
AMH-overexpressing (Thy1.2-AMHTg/0) mice express a human AMH transgene under the control of the Thy1.2 promoter, which drives AMH expression in central nervous system neurons with sufficient secretion to increase AMH levels in circulating blood (McLennan et al., 2018). This line was maintained on a C57Bl6/J genetic background that was congenic to the Amh+/− colony. Wild-type controls were the Thy1.2-AMH0/0 littermates of the Thy1.2-AMHTg/0 mice. The genotypes of hemizygous transgenic mice were determined by PCR using a forward primer directed to the Thy1.2 promoter region (5′-TGTAGCTTTCCCCACCACAGA-3′) and a reverse primer to the human AMH transgene (5′-AGGGCCTCAGTCCCCAGCAGA-3′). The adult Thy1.2-AMHTg/0 and Thy1.2-AMH0/0 mice were 42–120 days of age.
All animals were housed in groups of 3–5 mice in individually ventilated cages (Techniplast, Buguggiate, Italy) with ad libitum access to food and water under a 12:12 h daily light:dark cycle. None of the experimental animals had been a part of any prior experimentation/procedures. All experiments were approved by the University of Otago Animal Ethics Committee.
Follicle counting in Amh+/+ and Amh−/− mouse ovaries
Mouse oestrous cycles were determined by examining cytology of vaginal lavage fluid daily. Lavage fluid was dried on to microscope slides and stained for 1 min with 0.5 mg/ml toluidine blue. The animals were euthanized between at 10:00 and 12:00 h by anaesthetic overdose, immediately prior to ovulation (pro-oestrus), and ovaries were fixed in Bouins’ fixative prior to wax-embedding. Serial sections were cut at 5 µm intervals for hemotoxylin and eosin staining. Primordial, transitioning and primary follicle counts were estimated by physical-disector stereology, sampling every 10th section of one ovary from each mouse (unilateral) according to previously published stereological methods (Myers et al., 2004; Guo and Pankhurst, 2020). Follicles with oocytes surrounded by flattened granulosa cells were classified as primordial. Follicles with oocytes surrounded by a single layer of cuboidal granulosa cells were classified as primary follicles. Follicles were classified as transitioning between the primordial and primary stage when there was a single layer of granulosa cells with a combination of flattened and cuboidal morphology.
Follicles from the secondary stage and beyond were quantified using absolute counts of serial sections of both ovaries (bilateral) sampled at 25 µm intervals. Follicles were classified as secondary if they had at least two layers of granulosa cells at any part of the circumference of the follicle. The appearance of antral spaces was used to distinguish the smallest antral follicles from secondary follicles. Small antral follicles were <200 µm in diameter, medium antral follicles had diameters of 200–300 µm and follicles >300 µm in diameter were classified as large antral follicles.
Follicle survival ratios were calculated by dividing the number of follicles in each stage by the number of follicles in the preceding stage, for each mouse. The transitioning/primordial, primary/transitioning and secondary/primary follicle survival ratios were calculated using data collected from a single ovary and the small antral/secondary, medium antral/small antral and large antral/medium antral ratios were calculated from data from both ovaries of each mouse.
Immunohistochemistry
Adult wild-type and Thy1.2-AMHTg/0 ovaries were immersion-fixed in Bouins’ fixative, paraffin-embedded and serially sectioned at 5 µm thickness. Paraffin was removed from slides with xylene followed by graded ethanol solutions. Antibodies were applied in PBS with 0.08%w/v Tween20 (Sigma-Aldrich, Cat# P1379, Kenilworth, NY, USA) and 1%w/v bovine serum albumin. Anti-cleaved caspase-3 antibody (Abcam, Cat# ab2302, Cambridge, UK) was applied at 1 µg/ml and anti-PCNA antibody (Santa Cruz, Cat# SC-56, Santa Cruz, CA, USA) was applied at 0.1 µg/ml, overnight at 4°C. Secondary antibodies (4 µg/ml biotinylated donkey anti-rabbit IgG, Abcam, Cat# ab6801 or 1:500 biotinylated donkey anti-mouse IgG, Jackson Immuno Research Lab, Cat# 715-065-151, West Grove, PA, USA) and streptavidin-biotin-HRP complex (1:200, GE Healthcare, Cat# RPN1051, Chicago, IL, USA) were each applied for 1 h at room temperature. Immunoreactivity was visualized with diaminobenzidine (DAB substrate kit, Vector Labs, Cat# SK-4100, Newark, CA, USA) and the sections were counterstained with haematoxylin.
Granulosa cell mitotic index calculation
Follicle development rates were determined by calculating a mitotic index of the granulosa cells based on PCNA immunoreactivity. The ovaries of three Amh+/+ mice and three Amh−/− mice were examined. A total of 12–14 ovary sections were obtained from each mouse for anti-PCNA immunohistochemistry. PCNA-immunoreactive and PCNA-negative granulosa cell counts were obtained for all transitioning, primary and secondary follicles. The distance between the sections examined was at least 20 µm and follicles were excluded if the oocyte nucleus was not visible, to prevent double-counting of follicles. The mitotic index in each follicle was calculated by dividing the PCNA-immunoreactive granuosa cell number by the total granulosa cell number.
Cleaved caspase-3 positive preantral follicle counts
Power calculations determined that at least 80 follicles in total would be needed to compare differences in the percentages of atretic and non-atretic follicles between wild-type and Thy1.2-AMHTg/0 mice. It was determined that 110 sections would need to be sampled in wild-type ovaries, and 138 sections from Thy1.2-AMHTg/0 ovaries to account for the lower abundance of preantral follicles in the AMH-overexpressing mice. The sections were obtained from four wild-type and four Thy1.2-AMHTg/0 mice. All primary and secondary follicles were counted, taking note of whether cleaved caspase-3 was present in any granulosa cells or oocytes. The distance between the sections examined was at least 20 µm and follicles were excluded if the oocyte nucleus was not visible, to prevent double-counting of follicles. Primary and secondary follicles that were structurally intact and showed no caspase-3 immunoreactivity were defined as non-atretic follicles. Cavities in the ovarian stroma containing cleaved caspase-3 degenerating oocytes or granulosa cells were judged to be late-stage atretic preantral follicles if they were within the size range of primary (25–57 µm) or secondary (56–125 µm) follicles. Cavities that contained no cleaved caspase-3 positive degenerating granulosa cells or oocytes were judged to be shrinking cavities derived from atretic follicles that had undergone atresia at a later stage of folliculogenesis and were not counted.
FSH and cetrorelix injection
To investigate whether FSH can rescue the loss of developing follicles in AMH-overexpressing mice, adult Thy1.2-AMHTg/0 female mice were divided into two groups. One group was given daily injections of 5 IU FSH i.p. (Genway Biotech, Cat# GWB-3EE59E, San Diego, CA, USA) and 4 µg of the GnRH antagonist, cetrorelix (Bachem, Cat# H-6682, Bubendorf, Switzerland) (Rizwan et al., 2012), to prevent the LH surge and ovulation, for 4 days. The control group was given daily saline injections i.p. over the same period to generate a control-group with normal FSH secretion. All injection periods began on the first day of oestrus. Animal welfare was monitored daily during the injection period. The mice were euthanized by anaesthetic overdose 24 h (between 10:00 and 12:00 h) after the final injection and the ovaries were immersion fixed and processed for cell counting according to the methods described above.
Analysis and statistics
Data were analysed using Prism version 9.0 (GraphPad, San Diego, CA, USA) and SPSS version 27 (IBM corporation, Armonk, NY, USA). Animals were allocated to experimental groups according to genotype and littermates with different genotypes were used in each group where possible. Levene’s test was used to determine the equality of variances. The Shapiro–Wilk test and Kolmogorov–Smirnov tests were used to determine the normality. Groups were analysed by Student’s t-test when the assumptions of normal distribution and homoscedasticity were met. In all other cases, Mann–Whitney U tests were used to compare between two groups. The proportions of cleaved caspase-3 positive and negative follicles were analysed by Fisher’s exact test. Differences were considered statistically significant when P < 0.05.
Results
At 100–120 days of age, the primordial follicle counts in Amh−/− mice tended to be lower than Amh+/+ mice but were not significantly different (Fig. 1A, P = 0.055). Similarly, the number of transitioning follicles was not significantly different in the Amh−/− and Amh+/+ mouse ovaries (Fig. 1B, P = 0.911). However, the primary and secondary follicle counts were higher in the Amh−/− mice compared to the Amh+/+ mice (Fig. 1C and D, P = 0.031 and P < 0.001, respectively). Higher follicle counts were also present at the small and medium antral stages in Amh−/− mice when compared with Amh+/+ ovaries (Fig. 1E and F, P = 0.003 and P = 0.015, respectively). However, when the follicles reached the large antral stage, no significant differences were observed between the two genotypes (Fig. 1G, P = 0.551). The large antral follicle counts were sufficient to support normal ovulation rates, which we have previously reported to be 8–9 oocytes in this C57Bl6/J mouse colony (Guo and Pankhurst, 2020; Zhou et al., 2022).
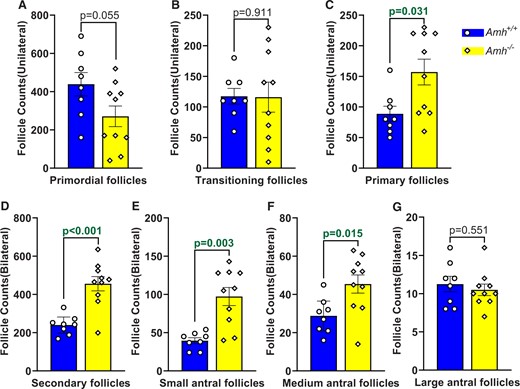
Absolute follicle counts in Amh+/+ and Amh−/− mice at 100–120 days of age. Primordial (A), transitioning (B) and primary follicles (C) were counted in single ovaries per mouse. Secondary (D), small antral (E), medium antral (F) and large antral follicles (G) were counted across both ovaries. Data are expressed as mean ± SEM and data were analysed by Student’s t-test (A, D, F, G) unless the assumptions were not met, in which case Mann–Whitney U tests were used (B, C, E). Amh+/+ mice n = 8, Amh−/− mice n = 10. AMH, anti-Müllerian hormone.
AMH is reported to alter follicle development rates in vitro (Durlinger et al., 2001; McGee et al., 2001; Hayes et al., 2016; Xu et al., 2016, 2018; Baba et al., 2017). Differences in Amh+/+ and Amh−/− follicle development rates could explain why greater numbers of follicles were present at more advanced stages in Amh−/− mice. Mitotic indexes for developing follicles were generated from immunohistochemical staining for the proliferation marker, PCNA (Fig. 2D). No significant differences in follicle development speed were observed in transitioning (P = 0.585), primary (P = 0.176) and secondary (P = 0.422) follicles between Amh−/− and Amh+/+ mice (Fig. 2). This suggests that the differences in the numbers of developing follicles in Amh−/− and Amh+/+ mice are not caused by differences in follicle development speed, which led to further investigation of follicle survival rates.
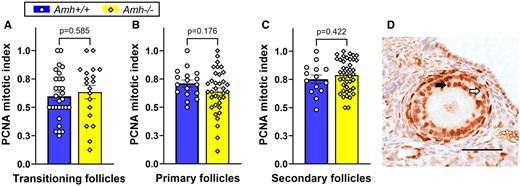
Granulosa cell mitotic index as a measure of follicle development rate in adult Amh+/+ and Amh−/− mice. (A) transitioning follicles (Amh+/+: n = 29, Amh−/−: n = 19), (B) primary follicle (Amh+/+: n = 17, Amh−/−: n = 35) and (C) secondary follicles (Amh+/+: n = 14, Amh−/−: n = 41) showed no significant difference between the genotypes (Student’s t-test). (D) Example of PCNA immunohistochemical staining (black arrow) and unstained (white arrow) granulosa cells in a secondary follicle from an Amh−/− mouse. Data are expressed as mean ± SEM Scale bar: 50 µm. AMH, anti-Müllerian hormone; PCNA, proliferating cell nuclear antigen.
Survival ratios for each follicle stage were calculated to compare the relative rates of progression to successive follicle stages in Amh+/+ and Amh−/− mice. As expected, the transitioning/primordial follicle ratio was higher in Amh−/− mice (Fig. 3A, P = 0.021), which is consistent with a greater rate of primordial follicle activation than Amh+/+ mice. However, Amh−/− mice also had a greater survival rate between the transitioning and primary stages (Fig. 3B, P = 0.009) when compared with Amh+/+ mouse ovaries. This equated to 43% fewer transitioning follicles surviving to the primary stage in Amh+/+ mice. Primary to secondary follicle survival rates were similar between the genotypes (Fig. 3C, P = 0.231) but 24% fewer secondary follicles survived to the small antral stage in Amh+/+ mice relative to Amh−/− mice (Fig. 3D, P = 0.030). This relationship became reversed as the follicles reached the FSH-dependent medium antral stage, with a smaller percentage of small antral follicles surviving to the next stage in Amh−/− mice relative to Amh+/+ mice (Fig. 3E, P = 0.007). This trend continued in the final stages of folliculogenesis with a smaller percentage of medium antral follicles surviving to the large antral stage in Amh−/− mice relative to Amh+/+ mice (Fig. 3F, P = 0.006).
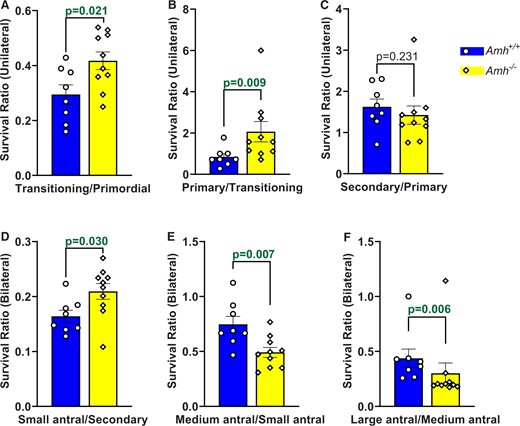
Activation and survival ratios between successive follicle development stages in Amh+/+ and Amh−/− mice. Survival ratios were calculated by dividing the follicle count at a particular stage by the follicle count at the preceding stage for each mouse. Survival ratios are shown for transitioning/primordial (A), primary/transitioning (B), secondary/primary (C), small antral/secondary (D), medium antral/small antral (E) and large antral/medium antral (F) follicles. Data are expressed as mean ± SEM and were analysed by Student’s t-test (A, D, E) unless the assumptions were not met, in which case Mann–Whitney U tests were used (B, C, F). Amh+/+ mice n = 8, Amh−/− mice n = 10. AMH, anti-Müllerian hormone.
The Thy1.2-AMHTg/0 AMH-overexpressing mouse model increases serum AMH concentrations by ∼1 nmol/l (McLennan et al., 2018), which is higher than normal serum AMH concentrations but lower than the concentrations of AMH found in follicular fluid (∼6 nmol/l, Jeppesen et al., 2013). This transgenic line also experiences reduced preantral follicle counts (Pankhurst et al., 2018), which was hypothesized to arise from greater preantral follicle atresia. Cleaved caspase-3 immunohistochemistry was performed to assess apoptosis in preantral follicles. No caspase-positive primordial or transitioning follicles were observed in either Thy1.2-AMHTg/0 or wild-type mice (Fig. 4A). As predicted, intact atretic preantral follicles were very rare, as only 1/64 follicles from 138 ovary sections were found to have active caspase-3 in Thy1.2-AMHTg/0 mice (Fig. 4B) and 0/163 follicles from 110 sections were observed in wild-type mice. However, primary and secondary follicle-sized cavities were frequently observed in the ovaries of both genotypes, with some cavities containing degenerating oocytes or granulosa cells with caspase-3 activity (Fig. 4C and D). Cavities without cleaved caspase-3-positive cellular debris were excluded from further analysis as they were assumed to be older and thus it was not possible to determine when the atresia had occurred or what final size the follicle had attained. The preantral follicle-sized cavities (with caspase-3 positive cellular debris) were more prevalent in Thy1.2-AMHTg/0 ovaries relative to wild-type ovaries follicles (Fig. 4G). Collectively, this confirms that preantral follicle atresia is difficult to detect but AMH-overexpressing mice have higher rates of stromal cavities containing cellular debris, consistent with recent preantral follicle atresia.
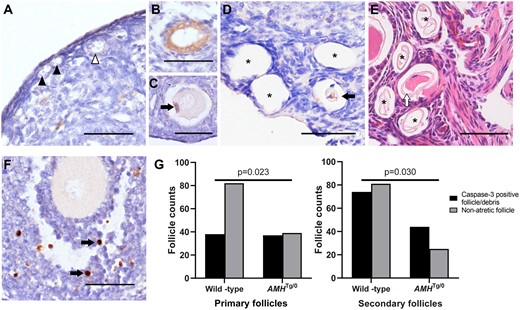
Follicle atresia in AMH-overexpressing (Thy1.2-AMHTg/0) mice. Cleaved (activated) caspase-3 immunoreactivity (brown DAB staining with blue haematoxylin counter stain) was not readily apparent in primordial (black arrowhead) and transitioning (white arrowhead) follicles (A). The cleaved caspase-3 immunostaining was observed in the granulosa cells of primordial follicles (B) and in the granulosa cells (C, black arrow) or oocytes (D, black arrow) of primary/secondary follicles at more-advanced stages of atresia. Numerous primary follicle-sized empty cavities were also observed (D, asterisk) and were shown to contain the remnants of zonae pellucidae via haematoxylin and eosin staining (E, asterisks). Cleaved caspase-3 was commonly found in a small number of granulosa cells (black arrows) in healthy developing follicles, serving as an internal positive control (F). Scale bars: 50 µm. The ratios of non-atretic primary and secondary follicles and similar-sized stromal cavities containing cleaved-caspase-3 positive degenerating oocytes or granulosa cells were assessed by Fisher’s exact test (G), follicle counts were obtained from n = 4 mice per group. AMH, anti-Müllerian hormone; DAB, diaminobenzidine.
Numerous studies have suggested that the actions of FSH and AMH in granulosa cells counteract each other (Grossman et al., 2008; Pellatt et al., 2011; Campbell et al., 2012). To investigate whether FSH can rescue the loss of developing follicles in Thy1.2-AMHTg/0 mice, daily FSH injections were administered for 4 days and compared to saline injections. The FSH-injected mice were also administered the GnRH antagonist cetrorelix to prevent ovulation, as the saline-injected mice were not expected to ovulate within the injection period. There were no statistically significant differences in the primary/primordial or secondary/primary follicle ratios (Fig. 5). These ratios were substantially lower than those observed in wild-type (Amh+/+) mice indicating that the FSH treatment did not restore normal primary follicle survival in Thy1.2-AMHTg/0 ovaries.
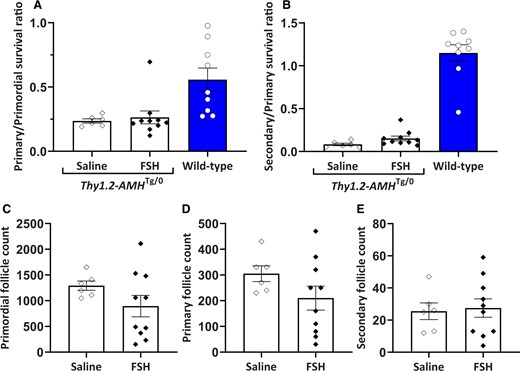
Follicle survival in AMH-overexpressing (Thy1.2-AMHTg/0) mice was not rescued by FSH treatment. Primary (A) and secondary (B) follicle survival rates in Thy1.2-AMHTg/0 mice treated with either FSH and cetrorelix (n = 10) or saline injection (n = 6). Follicle counts for primordial (C), primary (D) and secondary (E) follicles in the saline- and FSH-injected groups were conducted on one ovary from each mouse. Comparisons between the saline and FSH groups were compared with Student’s t-test. No significant differences were detected. Data are expressed as mean ± SEM. Data from untreated Amh+/+ mice are shown for comparison but were not included in the statistical analysis. AMH, anti-Müllerian hormone.
Discussion
The results of the present study are consistent with prior studies; Amh−/− mice have increased rates of primordial follicle activation, increased developing follicle numbers and normal preovulatory follicle counts (Durlinger et al., 1999; Visser et al., 2007). At young ages, transitioning follicle numbers are greater in Amh−/− mice owing to higher rates of primordial follicle activation (Durlinger et al., 1999) but at older ages, the Amh−/− mouse ovarian reserve becomes so depleted that Amh+/+ mice have a greater number of transitioning follicles (Guo and Pankhurst, 2020). In the present study, we appear to have discovered the point of crossover where Amh−/− and Amh+/+ mice have the same number of transitioning follicles initiating folliculogenesis over a given timeframe (100–120 days of age). Despite this, the numbers of preantral follicles were still greater in the Amh−/− mice relative to Amh+/+ mice, indicating that the difference must arise during early folliculogenesis, after the primordial follicle transition. This finding was further supported by the increased rates of preantral follicle atresia observed in Thy1.2-AMHTg/0 mice. The increased numbers of follicles surviving to the antral stage in Amh−/− mice creates a requirement for a larger amount of antral follicle atresia at the FSH-dependent phase, as the Amh−/− and Amh+/+ mice had the same number of preovulatory follicles.
The majority of follicle atresia is thought to occur at the antral stage, as this is where atresia is most frequently observed (McGee and Hsueh, 2000). However, antral follicles are large structures where apoptosis affects the cells gradually, and requires substantial phagocytosis to clear away the post-apoptotic cellular remnants and, thus, is likely a slow process. We observed that intact atretic preantral follicles are rare but the cavities left behind are common, albeit with very few apoptotic cells remaining within. This suggests that preantral follicles are removed quickly, making them difficult to observe. The exact timescales remain unclear, preventing precise quantification of atresia rates. However, a young female mouse is expected to activate ∼90 follicles per day (Faddy et al., 1987) and the primary follicle phase is estimated to last ∼12 days (the midpoint of estimates of the duration of the primary follicle phase, Hoage and Cameron, 1976; Zheng et al., 2014). Multiplying these two numbers indicates that a young mouse ovary should contain ∼1080 transitioning/primary follicles at any given time. The present data suggest that AMH-mediated atresia will remove ∼43% (464) of these follicles before they reach the next stage, representing 39 follicles a day. In a typical follicle-counting experiment a researcher might obtain around 800 sections (5 µm thick) from an ovary and perform primary follicle counts on about 40. If the 39 atretic primary follicles are evenly distributed throughout the ovary then, probabilistically, the researcher is likely to observe one intact atretic follicle in one of the 40 sections that they perform follicle counts on. The frequency of these observations was substantially lower in this study suggesting that atretic preantral follicles are removed rapidly. This indicates that direct observation is not a viable method for studying preantral follicle atresia. However, the deficit in preantral follicle numbers in the Amh+/+ mice relative to Amh−/− mice suggests that preantral follicle atresia is prevalent.
Knockout and transgenic studies are not possible in humans but sufficient data exist to conduct preliminary calculations of preantral follicle loss in humans. The median 20- to 25-year-old woman loses ∼5400 primordial follicles year (∼450/month) (Wallace and Kelsey, 2010) but an average of ∼15 reach the 2–10 mm antral follicle stage at the beginning of the follicular phase of each cycle (Haadsma et al., 2007; Almog et al., 2011). This simple calculation suggests that >90% of the primary follicles activated each month will undergo atresia before reaching the gonadotropin-dependent antral stage. If preantral follicle atresia is as prevalent in humans as it is in mice, prevention of atresia may be a novel avenue to increase the number of antral follicles available for stimulation in ART. This has not been considered because of the prevailing view that preantral follicle atresia does not occur, but this study indicates that this should be readdressed. FSH stimulation did not appear to be capable of rescuing preantral follicles from the effects of AMH but there are numerous paracrine factors in the ovary that support preantral follicle growth (McLaughlin and McIver, 2009) that could be investigated further.
Experiments utilizing the treatment of developing ovarian follicle cultures with AMH have shown both inhibition of follicle growth (Durlinger et al., 2001; Hayes et al., 2016) and growth-promoting effects (McGee et al., 2001; Xu et al., 2016, 2018, 2021; Baba et al., 2017) but none has shown an increase in follicle atresia. However, these studies all used follicles from the late-secondary stages onwards. The current study suggests that follicles are susceptible to AMH-mediated atresia at the late primary and early secondary stages. Ovary explant or organ cultures treated with AMH generally lead to decreases in the ratio of primary follicles relative to primordial follicles (Durlinger et al., 2002; Gigli et al., 2005; Carlsson et al., 2006; Nilsson et al., 2007, 2014). All of these studies concluded that the change in the ratio occurred due to inhibition of primordial follicle activation but increased primary follicle atresia could cause the same result. More recently, studies have shown that AMH can reduce small follicle survival in vitro (Rocha et al., 2016) and can slow the rate of primary follicle growth, both in vivo and in vitro (Yang et al., 2017; Meinsohn et al., 2021). Therefore, there is increasing evidence that AMH affects preantral follicle growth, with this study providing evidence that it also affects preantral follicle survival.
AMH has been shown to inhibit the downstream effects of FSH signalling in granulosa cells in culture (Grossman et al., 2008; Pellatt et al., 2011; Campbell et al., 2012). However, in the present study, follicle atresia in the Amh−/− mouse largely occurred at the gonadotropin-dependent antral stages. The opposite effect is seen in the antral follicle population in Thy1.2-AMHTg/0 mice, where follicle survival is highest in the antral stages (Pankhurst et al., 2018). This indicates that elevated AMH does not induce atresia in the antral stages and that withdrawal of AMH does not have a measurable effect on normal dominant follicle selection mechanisms. FSH injections failed to rescue preantral follicles from atresia in Thy1.2-AMHTg/0 mice, which is consistent with findings showing that follicles <100 µm in diameter have minimal sensitivity to FSH when compared with larger follicles (Hardy et al., 2017). The present findings suggest that AMH-mediated follicle atresia only occurs in early follicles before they become sensitive to FSH. The present study provides no evidence that AMH and FSH interact in vivo, with regard to rates of atresia.
The widely accepted theory to explain the role of AMH on small follicles is that it conserves the ovarian reserve and extends the reproductive phase of life in females. However, no evidence for this was observed in fertility experiments in Amh−/− mice (Guo and Pankhurst, 2020). Even at supraphysiological doses, AMH could only partially inhibit primordial follicle activation, whereas primary follicle development was completely abolished at the same doses (Kano et al., 2017, 2019; Meinsohn et al., 2021). Thus, AMH has a stronger effect on developing follicles that have already been lost from the dormant primordial follicle pool, which is not consistent with conservation of ovarian reserve. Instead, we hypothesize that AMH-mediated inhibition of primordial follicle activation and primary follicle atresia are two distinct mechanisms that contribute a single function of paracrine AMH; to prevent the developing follicle pool from becoming too large. This may be required for normal follicle selection, as AMH-neutralizing antibodies were shown to increase the chances of poly-ovulation in macaques, a mono-ovulatory species (Xu et al., 2016). Increased numbers of preovulatory follicles were not observed in the Amh−/− females but this may be a limitation related to the polyovulatory cycles that occur in mice.
Large antral follicle counts are typically perceived to be advantageous, as peak fertility coincides with peak antral follicle counts. However, there may be diminishing returns beyond a certain number and possibly some disadvantages to maintaining large antral follicle counts within the ovaries. Recent studies in mice show that long-term hormonal contraceptives delay age-related infertility and aneuploidy (Isono et al., 2018; Chatzidaki et al., 2021). It is not clear how suppressing development of antral follicles affects fertility, but ovulation and atresia require constant tissue modelling in the ovary. Scarring and inflammation have been shown to accumulate with ageing (Briley et al., 2016; Amargant et al., 2020) but the amount of tissue remodelling required would be reduced if more follicles could be diverted to atresia at earlier stages of folliculogenesis, when the follicles are still relatively small. Currently, these concepts remain hypotheses and, while this study may demonstrate a new function for AMH, the biological purpose of this function requires further investigation, particularly in mono-ovulatory species.
This study demonstrates that AMH constantly induces primary follicle atresia in the normal functioning ovary. In 100- to 120-day-old Amh−/− mice, the absence of AMH-mediated primary follicle atresia is a larger determinant of the small antral follicle count than the absence of inhibition of primordial follicle activation. These findings suggest that AMH acts on small ovarian follicles to generate an upper limit to the size of the developing follicle pool.
Data Availability
The data underlying this article will be shared on reasonable request to the corresponding author.
Acknowledgements
We thank the staff of the Biomedical Resource Facility at the University of Otago for their technical assistance.
Authors’ roles
Y.Z., S.R. and N.J.B. contributed to acquisition of data, analysis and interpretation of data. M.W.P., D.E.O. and G.M.A. contributed to study conception and design and analysis and interpretation of data. All authors contributed to drafting the article or revising it critically for important intellectual content, final approval of the version to be published and all agree to be accountable for all aspects of the work in ensuring that questions related to the accuracy or integrity of any part of the work are appropriately investigated and resolved.
Funding
This research was funded by a Health Research Council of New Zealand Sir Charles Hercus Fellowship (grant #18-027). Y.Z. and S.R. were supported by a University of Otago Doctoral Scholarship.
Conflict of interest
The authors have no conflicts to declare.