-
PDF
- Split View
-
Views
-
Cite
Cite
Guillaume Martinez, Zine-Eddine Kherraf, Raoudha Zouari, Selima Fourati Ben Mustapha, Antoine Saut, Karin Pernet-Gallay, Anne Bertrand, Marie Bidart, Jean Pascal Hograindleur, Amir Amiri-Yekta, Mahmoud Kharouf, Thomas Karaouzène, Nicolas Thierry-Mieg, Denis Dacheux-Deschamps, Véronique Satre, Mélanie Bonhivers, Aminata Touré, Christophe Arnoult, Pierre F Ray, Charles Coutton, Whole-exome sequencing identifies mutations in FSIP2 as a recurrent cause of multiple morphological abnormalities of the sperm flagella, Human Reproduction, Volume 33, Issue 10, October 2018, Pages 1973–1984, https://doi.org/10.1093/humrep/dey264
- Share Icon Share
Abstract
Can whole-exome sequencing (WES) of infertile patients identify new genes responsible for multiple morphological abnormalities of the sperm flagella (MMAF)?
WES analysis of 78 infertile men with a MMAF phenotype permitted the identification of four homozygous mutations in the fibrous sheath (FS) interacting protein 2 (FSIP2) gene in four unrelated individuals.
The use of high-throughput sequencing techniques revealed that mutations in the dynein axonemal heavy chain 1 (DNAH1) gene, and in the cilia and flagella associated protein 43 (CFAP43) and 44 (CFAP44) genes account for approximately one-third of MMAF cases thus indicating that other relevant genes await identification.
This was a retrospective genetics study of 78 patients presenting a MMAF phenotype who were recruited in three fertility clinics between 2008 and 2015. Control sperm samples were obtained from normospermic donors. Allelic frequency for control subjects was derived from large public databases.
WES was performed for all 78 subjects. All identified variants were confirmed by Sanger sequencing. Relative mRNA expression levels for the selected candidate gene (FSIP2) was assessed by quantitative RT-PCR in a panel of normal human and mouse tissues. To characterize the structural and ultrastructural anomalies present in patients’ sperm, immunofluorescence (IF) was performed on sperm samples from two subjects with a mutation and one control and transmission electron microscopy (TEM) analyses was performed on sperm samples from one subject with a mutation and one control.
We identified four unrelated patients (4/78, 5.1%) with homozygous loss of function mutations in the FSIP2 gene, which encodes a protein of the sperm FS and is specifically expressed in human and mouse testis. None of these mutations were reported in control sequence databases. TEM analyses showed a complete disorganization of the FS associated with axonemal defects. IF analyses confirmed that the central-pair microtubules and the inner and outer dynein arms of the axoneme were abnormal in all four patients carrying FSIP2 mutations. Importantly, and in contrast to what was observed in patients with MMAF and mutations in other MMAF-related genes (DNAH1, CFAP43 and CFAP44), mutations in FSIP2 led to the absence of A-kinase anchoring protein 4 (AKAP4).
The low number of biological samples and the absence of a reliable anti-FSIP2 antibody prevented the formal demonstration that the FSIP2 protein was absent in sperm from subjects with a FSIP2 mutation.
Our findings indicate that FSIP2 is one of the main genes involved in MMAF syndrome. In humans, genes previously associated with a MMAF phenotype encoded axonemal-associated proteins (DNAH1, CFAP43 and CFAP44). We show here that FSIP2, a protein of the sperm FS, is also logically associated with MMAF syndrome as we showed that it is necessary for FS assembly and for the overall axonemal and flagellar biogenesis. As was suggested before in mouse and man, our results also suggest that defects in AKAP4, one of the main proteins interacting with FSIP2, would induce a MMAF phenotype. Finally, this work reinforces the demonstration that WES sequencing is a good strategy to reach a genetic diagnosis for patients with severe male infertility phenotypes.
This work was supported by the following grants: the ‘MAS-Flagella’ project financed by the French ANR and the DGOS for the program PRTS 2014 (14-CE15) and the ‘Whole genome sequencing of patients with Flagellar Growth Defects (FGD)’ project financed by the Fondation Maladies Rares for the program Séquençage à haut débit 2012. The authors have no conflict of interest.
Introduction
Teratozoospermia, a condition defined by the presence of sperm with abnormal morphology, comprises a large variety of phenotypes, many of which are among the most severe infertility phenotypes in human (Coutton et al., 2015). Patients with severe flagellar defects, and most strikingly presenting a much reduced flagellar length, have been described in numerous studies, and as ‘dysplasia of the fibrous sheath (DFS)’, ‘short tails’ or ‘stump tails’ (Chemes et al., 1987, 2000; Rawe et al., 2001; Chemes and Rawe, 2003). In our experience these patients do not present a homogeneous phenotype of short flagella but exhibit various flagellar abnormalities including absent, short, coiled, bent and irregular flagella (for illustration see Fig. 1). We therefore proposed to reclassify this heterogeneous phenotype comprising multiple morphological abnormalities of the sperm flagella (MMAF) for patients with a severe asthenozoospermia and with more than 5% of sperm showing at least four of the five aforementioned flagellar anomalies (Ben Khelifa et al., 2014). As the fibrous sheath (FS) is an internal structure of the flagella, detection of its anomalies (dysplasia) can only be achieved by ultrastructural observation of the flagella by electron microscopy (EM). As such studies are not performed in the routine setting, we did not feel that this designation was practical. The DFS appellation is however interesting as such anomalies are not always marked in sperm from MMAF patients, and DFS therefore likely represents a MMAF ultrastructural phenotype highlighting anomalies pertaining to this specific structure (see discussion in Coutton et al., 2015; Ray et al., 2017). In this article the generic term MMAF is normally used but we also use the term DFS when referring to subjects who have clearly been classified as DSF upon EM examination.
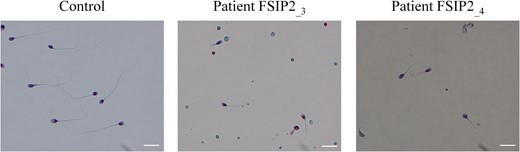
Morphology of spermatozoa from two patients with a mutation in the FSIP2 gene. All spermatozoa from patients FSIP2_3 and FSIP2_4 have shorter flagella than controls with no mutation in the fibrous sheath interacting protein 2 (FSIP2) gene. Additional features of spermatozoa from men with the multiple morphological abnormalities of the sperm flagella (MMAF) phenotype, such as thick and coiled flagella, are also observed. Scale bars 10 μm.
Mutations in DNAH1, CFAP43, CFAP44 and in cilia and flagella associated protein 69 (CFAP69) have recently been reported to be recurrent genetic causes of MMAF in human, highlighting the genetic heterogeneity of this phenotype (Ben Khelifa et al., 2014; Amiri-Yekta et al., 2016; Sha et al., 2017; Tang et al., 2017; Coutton et al., 2018; Dong et al., 2018). These genes encode different components of the axoneme, a highly conserved central cytoskeletal structure shared by both the sperm flagellum and other motile cilia (Inaba, 2007; Satir and Christensen, 2008). In mouse models, in addition to axonemal genes, mutations in several genes coding for accessory structures of the flagellum have also been associated with sperm tail defects (Lehti and Sironen, 2017). Interestingly, a first genetic diagnosis was proposed 12 years ago for a patient described with DFS. He presented with a deletion removing part of A-kinase anchoring protein 3 (AKAP3) and AKAP4, two genes encoding proteins of the sperm FS (Baccetti et al., 2005), one of the main peri-axonemal structures surrounding the flagellum axoneme (Inaba, 2003, 2011). However, no other mutations in AKAP genes or in any other FS-related genes have been reported since this initial description of an AKAP3/4 deletion (Turner et al., 2001; Coutton et al., 2015; Ray et al., 2017). More than 20 proteins are estimated to be located in, or closely associated with, the FS of mammalian spermatozoa and FSIP2 is believed to be a major structural protein of the FS (Eddy, 2007). Altogether, these proteins are involved in the control of sperm flagellum elasticity, glycolytic activities and phosphorylation signaling pathways that regulate sperm motility (Miki et al., 2002; Eddy, 2007; Inaba, 2011).
Previous genetic analysis of a large cohort of 78 infertile patients with MMAF permitted the identification of a total of 22 patients with mutations in DNAH1, CFAP43 and CFAP44 (Coutton et al., 2018). Here we re-analyzed the genetic data obtained by whole-exome sequencing (WES) from the 56 (out of 78) non-diagnosed patients and identified four unrelated subjects carrying bi-allelic loss of function mutations in FSIP2, which encodes a FS protein interacting with AKAP4 (Brown et al., 2003). Importantly, we observed in FSIP2-mutated individuals that the AKAP4 protein was absent from all spermatozoa; a feature which was not observed in spermatozoa from other MMAF individuals of different genetic etiology. Overall, our work confirms what has been suggested long ago: that not only axonemal components, but also peri-axonemal proteins from the FS play an important role in the assembly and/or the stability of the axoneme (Escalier and David, 1984; Chemes et al., 1987, 1998) and that genetic defects interfering with the competent synthesis of these proteins induce a MMAF phenotype.
Materials and Methods
Subjects and controls
All patients presented with a typical MMAF phenotype defined by asthenozoospermia (total motility <40%) with >5% of at least three flagellar morphological abnormalities (absent, short, coiled, bent and irregular flagella). The four FSIP2 patients (FSIP2_1 to FSIP2_4) originated from North Africa (Tunisians) and were treated in Tunis at the Clinique des Jasmins for primary infertility from 2008 to 2015. The four patients had normal somatic karyotypes 46,XY and were negative for Y chromosome microdeletions. These four patients were born from related parents, generally first cousins. Sperm analysis was carried out in the source laboratory during the course of the routine biological examination of the patient, according to World Health Organization guidelines (Wang et al., 2014). Written informed consent for genetic testing was obtained from all the subjects participating in the study according to the local protocols and the principles of the Declaration of Helsinki. Sperm samples permitting additional analyses (IF and EM) were only available from patients FSIP2_3 and FSIP2_4. In addition, the study was approved by local ethic committees. The samples were stored in the center for biological resources (CRB) Germetheque (certification under ISO-9001 and NF-S 96-900) following a standardized procedure. Control samples from fertile individuals with normal spermograms were obtained from CRB Germetheque. Consent for CRB storage was approved by the CPCP Sud-Ouest of Toulouse (co-ordination of the multisites CRB Germetheque).
WES and bioinformatics analysis
WES was performed according to our protocol described in Coutton et al. (2018). The main difference with the initial analysis was that we used an updated version of GATK and analyzed the variants which had been initially marked as variants of poor confidence. Briefly, genomic DNA was isolated from saliva using an Oragen DNA extraction kit (DNAgenotech®, Ottawa, Canada). Coding regions and intron/exon boundaries were enriched using the ‘all Exon V5 kit’ (Agilent Technologies, Wokingham, UK). DNA sequencing was undertaken at the Genoscope, Evry, France, on the HiSeq 2000 from Illumina® (San Diego, CA, USA). Sequence reads were aligned to the reference genome (hg19) using MAGIC (SEQC/MAQC-III Consortium, 2014). MAGIC produces quality-adjusted variant and reference read counts on each strand at every covered position in the genome. Duplicate reads and reads that mapped to multiple locations in the genome were excluded from further analysis. Positions where sequence coverage was below 10 on either the forward or reverse strand were marked as low confidence, and positions with coverage below 10 on both strands were excluded. Single nucleotide variations (SNV) and small insertions/deletions (indels) were identified and quality-filtered using in-house scripts, which included part of the Genome Analysis Toolkit (GATK 3.7). Briefly, for each variant, independent calls are made on each strand, and only those positions where both calls agreed were retained. The candidate variants were identified using an in-house bioinformatics pipeline, as follows. Variants with a minor allele frequency >5% in the NHLBI ESP6500 [Exome Variant Server, NHLBI GO Exome Sequencing Project (ESP), Seattle, WA, USA] or in 1000 Genomes Project phase 1 datasets (1000 Genomes Project Consortium et al., 2012), or >1% in ExAC (Lek et al., 2016) or gnomAD (http://gnomad.broadinstitute.org/) were discarded. We also compared these variants to an in-house database of 94 control exomes obtained from subjects mainly originating from North Africa and the Middle East, corresponding to the geographical origin of most patients from this study and which are under-represented in single nucleotide polymorphism public databases. All variants present in a homozygous state in this database were excluded. We used Variant Effect Predictor (VEP version 81 (McLaren et al., 2016)) to predict the impact of the selected variants. We only retained variants impacting splice donor/acceptor or causing frameshift, inframe insertions/deletions, stop gain, stop loss or missense variants, except those scored as ‘tolerated’ by SIFT (Kumar et al., 2009) (sift.jcvi.org) and as ‘benign’ by Polyphen-2 (Adzhubei et al., 2010) (genetics.bwh.harvard.edu/pph2).
Sanger sequencing
FSIP2 mutations identified by WES were validated by Sanger sequencing in each patient. The PCR primers and protocols used for each patient are listed in Supplementary Table SI. Sequencing reactions were carried out with BigDye Terminator v3.1 (Applied Biosystems) and were run on an ABI 3130XL system (Applied Biosystems™, ThemoFisher Scientific, Waltham, MA, USA). Sequences were analyzed using SeqScape software (Applied Biosystems).
Quantitative real-time RT-PCR analysis
Quantitative real-time RT-PCR (RT-qPCR) was performed with cDNAs from various tissues of human and mouse, including testes (Amsbio, Abingdon, UK). A panel of seven organs was used for the experiments: testis, brain, lung, kidney, liver, colon and heart. Each sample was assayed in triplicate for each gene on a StepOnePlus (Life Technologies® ThemoFisher Scientific), with Power SYBR®Green PCR Master Mix (Life Technologies®). The PCR cycle was as follows: 10 min at 95°C, 1 cycle for enzyme activation; 15 s at 95°C, 60 s at 60°C with fluorescence acquisition, and 40 cycles for the PCR. RT-qPCR data were normalized using the reference housekeeping actin beta gene (ACTB) for human with the −ΔΔCt method (Livak and Schmittgen, 2001). The 2 − ΔΔCt value was set at 0 in brain cells, resulting in an arbitrary expression of 1. Primer sequences and RT-qPCR conditions are indicated in Supplementary Table SII. The efficacy of primers was checked using a standard curve. Melting curve analysis was used to confirm the presence of a single PCR product.
Statistical analysis was performed using a two-tailed Student’s t-test on Prism 4.0 software (GraphPad, San Diego, CA, USA) to compare the relative expression of FSIP2 transcripts in several organs. Statistical tests with a two-tailed P values ≤ 0.05 were considered significant.
Immunostaining in human sperm cells
Immunofluorescence (IF) staining were performed using sperm cells from control individuals, patients FSIP2_3 and FSIP2_4 and MMAF patients mutated in CFAP43 and CFAP44 and DNAH1. Sperm cells were fixed in PBS/4% paraformaldehyde for 1 min at room temperature. After washing in 1 ml PBS, the sperm suspension was spotted onto 0.1% poly l-lysine pre-coated slides (Thermo Scientific, ThemoFisher Scientific). After attachment, sperm were washed and permeabilized with 0.1% (v/v) Triton X-100—Dulbecco’s phosphate-buffered saline (Triton X-100; Sigma-Aldrich St. Louis MO, USA.) for 30 min at room temperature. Slides were then blocked in 2% corresponding normal serum–DPBS (normal goat or donkey serum; GIBCO, Invitrogen, Carlsbad, CA, USA) and incubated overnight at 4°C with primary antibodies. Washes were performed with 0.1% (v/v) Triton X-100–DPBS, followed by 90 min incubation at room temperature with secondary antibodies. Appropriate controls were performed, omitting the primary antibodies. Samples were counterstained with 2 μg/ml DAPI (Sigma-Aldrich) and mounted with DAKO mounting media (Life Technology, ThemoFisher Scientific). Fluorescence images were captured with a Zeiss LSM 710 confocal microscope (Carl Zeiss, Oberkochen, Germany). Primary antibodies: polyclonal rabbit anti-AKAP4 (HPA020046, Sigma-Aldrich, 1:100), rabbit polyclonal anti-sperm associated antigen 6 (SPAG6) (HPA038440, Sigma-Aldrich, 1:500), rabbit polyclonal anti-dynein axonemal heavy chain 5 (DNAH5) (HPA037470, Sigma-Aldrich, 1:100), rabbit polyclonal anti-dynein axonemal light intermediate chain 1 (DNALI1) (HPA028305, Sigma-Aldrich, 1:100), mouse monoclonal anti-heat shock protein 60 (HSP60) (ab13532, 1:500, Abcam, Cambridge UK), monoclonal mouse anti-acetylated-α-tubulin (T7451, Sigma-Aldrich, 1:2000). Secondary antibodies: Highly cross-adsorbed secondary antibodies Dylight 488 and Dylight 549 (1:1000, Jackson Immunoresearch®; Ely, UK).
Transmission EM of human sperm cells
We performed transmission EM (TEM) experiments using sperm cells from fertile control individuals and from patient FSIP2_4 carrying the homozygous variant c.16389_16392delAATA in FSIP2. Sperm cells were fixed with 2.5% glutaraldehyde and 2% formaldehyde in 0.1 M sodium phosphate (pH 7.4) for 2 h at room temperature. Cells were washed with phosphate buffer and post fixed with 1% osmium tetroxide in the same buffer for 1 h at 4°C. After washing with distilled water, cells were stained overnight at 4°C with 0.5% uranyl acetate pH 4.0. Cells were dehydrated through graded alcohols (30, 60, 90, 100, 100 and 100%; 10 min for each bath) and infiltrated with a mix of 1:1 Epon/alcohol 100% for 2 h before two washes in fresh Epon for 2 h. Finally, cells were immersed in fresh Epon and polymerized for 2 days at 60°C. Ultrathin sections of the cell pellet were prepared with an ultramicrotome (Leica Wetzlar, Germany). Sections were post-stained for 10 min with 5% uranyl acetate pH 4.0, washed four times with distilled water (1 h), and then stained with 0.4% lead citrate before being observed in an JEOL 1200EX electron microscope at 80 kV (JEOL Ltd, Tokyo, Japan).
Results
WES identifies homozygous truncating mutations in FSIP2 in four MMAF patients
Here we re-analyzed the genetic data obtained by WES from the 56 (out of 78) non-diagnosed patients (Coutton et al., 2018). We searched for novel genes associated with MMAF by performing an extensive reanalysis of the remaining exomes and applying stringent technical and biological filters. We identified four patients (4/78, 5.1%) with homozygous truncating mutations in the FSIP2 gene. The FSIP2 gene (NM_173651) encodes a 6907-amino acid protein (Q5CZC0) and is described as one of the main genes of the flagella FS (Eddy, 2007). All identified variants: c.910delC, c.16389_16392delAATA, c.2282dupA and a complex variant c.1606_1607insTGT; 1607_1616delAAAGATTGCA are frameshift variants inserting a premature stop codon and likely abrogating the production of a functional protein. Details of the variants are provided in Fig. 2 and in Supplementary Table SIII. All the identified variants were absent from controls in the sequence databases (dbSNP, 1000 Genomes Project, NHLBI Exome Variant Server, gnomAD and in-house database), which is consistent with the expected key role of FSIP2 in male reproduction and the negative selection of the mutations.
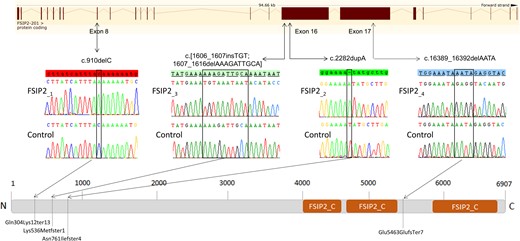
Location of FSIP2 mutations in the intron–exon structure and in the protein representation. Electropherograms of Sanger sequencing for all patients with FSIP2 mutations compared to the reference sequence are reported. Orange squares show the FSIP2_C domains (fibrous sheath-interacting protein 2, C-terminal, IPR031554) as predicted by InterPro server (https://www.ebi.ac.uk/interpro/). Mutations are annotated in accordance to the Human Genome Variation Society’s recommendations. According to the InterPro server, three homologous domains called FSIP2_C (C-terminus of the FSIP2 protein) are present in the C terminal part of the protein. FSIP2_C domains are present in other proteins and have been found to be repeated up to 10 times but their function is not yet known (Finn et al., 2017).
The FSIP2 gene was reported in public expression databases to be strongly expressed in the testis and to be connected with the sperm flagellum (Brown et al., 2003). We performed RT-qPCR experiments, which confirmed at this level of sensitivity that, in mouse and human, FSIP2 is exclusively expressed in the testis (Supplementary Fig. S1).
In conclusion, taking into account the identification of several unrelated patients carrying homozygous FSIP2 truncating mutations absent from all control sequences databases; the absence in these patients of other pathogenic variants in genes significantly expressed in testis and/or related to cilia/flagellum; the specific FSIP2 expression in testes; and data from the literature reporting the involvement of AKAP4, a protein interacting with FSIP2, in human and mouse MMAF-like phenotype, we conclude that the identified FSIP2 mutations are responsible for the MMAF phenotype of patients FSIP2_1–4.
Genotype–phenotype correlation
The morphology of patients’ sperm was assessed with Papanicolaou and Harris-Schorr staining (Fig. 1). The detailed semen parameters of the four FSIP2 patients are presented in Table I and the average semen parameters of the studied MMAF cohort according to the genotype are described in Table II.
Detailed semen parameters in the four patients with the multiple morphological abnormalities of the sperm flagella phenotype and a mutation in the fibrous sheath interacting protein 2 gene.
FSIP2-mutated patients . | Semen parameters . | |||||||||||
---|---|---|---|---|---|---|---|---|---|---|---|---|
Patient ID . | Age (years) . | FSIP2 mutations . | Sperm volume (ml) . | Sperm concentration (106/ml) . | Total motility (1 h) . | Vitality . | Normal spermatozoa . | Absent flagella . | Short flagella . | Coiled flagella . | Bent flagella . | Flagella of irregular caliber . |
FSIP2_1 | 44 | c.[910delC] | 2.5 | 6.7 | 1 | 47 | 0 | 2 | 32 | 26 | – | 57 |
FSIP2_2 | 52 | c.[2282dupA] | 4.7 | 1.25 | 0 | 7 | 0 | 16 | 50 | 18 | – | 36 |
FSIP2_3 | 45 | c.[1606_1607insTGT; 1607_1616delAAAGATTGCA] | 3.4 | 0.66 | 0 | 30 | 0 | 6 | 78 | 6 | – | 6 |
FSIP2_4 | 43 | c.[16389_16392delAATA] | 1.7 | 49.5 | 5 | 52 | 0 | 6 | 70 | 14 | – | 80 |
FSIP2-mutated patients . | Semen parameters . | |||||||||||
---|---|---|---|---|---|---|---|---|---|---|---|---|
Patient ID . | Age (years) . | FSIP2 mutations . | Sperm volume (ml) . | Sperm concentration (106/ml) . | Total motility (1 h) . | Vitality . | Normal spermatozoa . | Absent flagella . | Short flagella . | Coiled flagella . | Bent flagella . | Flagella of irregular caliber . |
FSIP2_1 | 44 | c.[910delC] | 2.5 | 6.7 | 1 | 47 | 0 | 2 | 32 | 26 | – | 57 |
FSIP2_2 | 52 | c.[2282dupA] | 4.7 | 1.25 | 0 | 7 | 0 | 16 | 50 | 18 | – | 36 |
FSIP2_3 | 45 | c.[1606_1607insTGT; 1607_1616delAAAGATTGCA] | 3.4 | 0.66 | 0 | 30 | 0 | 6 | 78 | 6 | – | 6 |
FSIP2_4 | 43 | c.[16389_16392delAATA] | 1.7 | 49.5 | 5 | 52 | 0 | 6 | 70 | 14 | – | 80 |
FSIP2, fibrous sheath interacting protein 2. Values are expressed as a percentage, unless specified otherwise.
Detailed semen parameters in the four patients with the multiple morphological abnormalities of the sperm flagella phenotype and a mutation in the fibrous sheath interacting protein 2 gene.
FSIP2-mutated patients . | Semen parameters . | |||||||||||
---|---|---|---|---|---|---|---|---|---|---|---|---|
Patient ID . | Age (years) . | FSIP2 mutations . | Sperm volume (ml) . | Sperm concentration (106/ml) . | Total motility (1 h) . | Vitality . | Normal spermatozoa . | Absent flagella . | Short flagella . | Coiled flagella . | Bent flagella . | Flagella of irregular caliber . |
FSIP2_1 | 44 | c.[910delC] | 2.5 | 6.7 | 1 | 47 | 0 | 2 | 32 | 26 | – | 57 |
FSIP2_2 | 52 | c.[2282dupA] | 4.7 | 1.25 | 0 | 7 | 0 | 16 | 50 | 18 | – | 36 |
FSIP2_3 | 45 | c.[1606_1607insTGT; 1607_1616delAAAGATTGCA] | 3.4 | 0.66 | 0 | 30 | 0 | 6 | 78 | 6 | – | 6 |
FSIP2_4 | 43 | c.[16389_16392delAATA] | 1.7 | 49.5 | 5 | 52 | 0 | 6 | 70 | 14 | – | 80 |
FSIP2-mutated patients . | Semen parameters . | |||||||||||
---|---|---|---|---|---|---|---|---|---|---|---|---|
Patient ID . | Age (years) . | FSIP2 mutations . | Sperm volume (ml) . | Sperm concentration (106/ml) . | Total motility (1 h) . | Vitality . | Normal spermatozoa . | Absent flagella . | Short flagella . | Coiled flagella . | Bent flagella . | Flagella of irregular caliber . |
FSIP2_1 | 44 | c.[910delC] | 2.5 | 6.7 | 1 | 47 | 0 | 2 | 32 | 26 | – | 57 |
FSIP2_2 | 52 | c.[2282dupA] | 4.7 | 1.25 | 0 | 7 | 0 | 16 | 50 | 18 | – | 36 |
FSIP2_3 | 45 | c.[1606_1607insTGT; 1607_1616delAAAGATTGCA] | 3.4 | 0.66 | 0 | 30 | 0 | 6 | 78 | 6 | – | 6 |
FSIP2_4 | 43 | c.[16389_16392delAATA] | 1.7 | 49.5 | 5 | 52 | 0 | 6 | 70 | 14 | – | 80 |
FSIP2, fibrous sheath interacting protein 2. Values are expressed as a percentage, unless specified otherwise.
Average semen parameters in patients mutated in FSIP2, in other xonemal-associated genes, and for the 78 subjects with multiple morphological abnormalities of the sperm flagella in the study.
Semen parameters . | MMAF with FSIP2 mutations . | MMAF other mutations$ . | Overall MMAF . | Reference limits(%) . |
---|---|---|---|---|
n = 4 . | n = 22 . | n = 78 . | ||
Mean age (years) | 46 ± 4 | 39.8 ± 7 | 41.6 ± 7.7 | NA |
(n′ = 4) | (n′ = 21) | (n′ = 77) | ||
Sperm volume (ml) | 3.1 ± 1.3 | 3.4 ± 1.2 | 3.5 ± 1.4 | 1.5 (1.4–1.7) |
(n′ = 4) | (n′ = 20) | (n′ = 75) | ||
Sperm concentration (106/ml) | 14.5 ± 23.5 | 20.1 ± 18.8 | 25.6 ± 32.1 | 15 (12–16) |
(n′ = 4) | (n′ = 20) | (n′ = 75) | ||
Total motility (1 h) | 1.5 ± 2.4 | 0.7 ± 2.4 | 3.9 ± 5.6 | 40 (38–42) |
(n′ = 4) | (n′ = 21) | (n′ = 76) | ||
Vitality | 34 ± 20.3 | 50.5 ± 22.7 | 52.7 ± 20 | 58 (55–63) |
(n′ = 4) | (n′ = 19) | (n′ = 72) | ||
Normal spermatozoa | 0 ± 0 | 0.5 ± 2.3 | 1.6 ± 2.7 | 23 (20–26) |
(n′ = 4) | (n′ = 20) | (n′ = 61) | ||
Absent flagella | 7.5 ± 5.9* | 28.1 ± 14.4 | 20.7 ± 15.7 | 5 (4–6) |
(n′ = 4) | (n′ = 15) | (n′ = 66) | ||
Short flagella | 57.5 ± 20.6 | 57.1 ± 27.9 | 43.7 ± 27.3 | 1 (0–2) |
(n′ = 4) | (n′ = 19) | (n′ = 72) | ||
Coiled flagella | 16 ± 8.3 | 10.4 ± 6.6 | 12.8 ± 9.4 | 17 (15–19) |
(n′ = 4) | (n′ = 16) | (n′ = 69) | ||
Bent flagella | NA | 8.7 ± 5.8 | 4.2 ± 8.4 | 13 (11–15) |
(n′ = 6) | (n′ = 26) | |||
Flagella of irregular caliber | 44.8 ± 31.5 | 27.9 ± 19.4 | 31.7 ± 25.1 | 2 (1–3) |
(n′ = 4) | (n′ = 15) | (n′ = 67) |
Semen parameters . | MMAF with FSIP2 mutations . | MMAF other mutations$ . | Overall MMAF . | Reference limits(%) . |
---|---|---|---|---|
n = 4 . | n = 22 . | n = 78 . | ||
Mean age (years) | 46 ± 4 | 39.8 ± 7 | 41.6 ± 7.7 | NA |
(n′ = 4) | (n′ = 21) | (n′ = 77) | ||
Sperm volume (ml) | 3.1 ± 1.3 | 3.4 ± 1.2 | 3.5 ± 1.4 | 1.5 (1.4–1.7) |
(n′ = 4) | (n′ = 20) | (n′ = 75) | ||
Sperm concentration (106/ml) | 14.5 ± 23.5 | 20.1 ± 18.8 | 25.6 ± 32.1 | 15 (12–16) |
(n′ = 4) | (n′ = 20) | (n′ = 75) | ||
Total motility (1 h) | 1.5 ± 2.4 | 0.7 ± 2.4 | 3.9 ± 5.6 | 40 (38–42) |
(n′ = 4) | (n′ = 21) | (n′ = 76) | ||
Vitality | 34 ± 20.3 | 50.5 ± 22.7 | 52.7 ± 20 | 58 (55–63) |
(n′ = 4) | (n′ = 19) | (n′ = 72) | ||
Normal spermatozoa | 0 ± 0 | 0.5 ± 2.3 | 1.6 ± 2.7 | 23 (20–26) |
(n′ = 4) | (n′ = 20) | (n′ = 61) | ||
Absent flagella | 7.5 ± 5.9* | 28.1 ± 14.4 | 20.7 ± 15.7 | 5 (4–6) |
(n′ = 4) | (n′ = 15) | (n′ = 66) | ||
Short flagella | 57.5 ± 20.6 | 57.1 ± 27.9 | 43.7 ± 27.3 | 1 (0–2) |
(n′ = 4) | (n′ = 19) | (n′ = 72) | ||
Coiled flagella | 16 ± 8.3 | 10.4 ± 6.6 | 12.8 ± 9.4 | 17 (15–19) |
(n′ = 4) | (n′ = 16) | (n′ = 69) | ||
Bent flagella | NA | 8.7 ± 5.8 | 4.2 ± 8.4 | 13 (11–15) |
(n′ = 6) | (n′ = 26) | |||
Flagella of irregular caliber | 44.8 ± 31.5 | 27.9 ± 19.4 | 31.7 ± 25.1 | 2 (1–3) |
(n′ = 4) | (n′ = 15) | (n′ = 67) |
MMAF, multiple morphological abnormalities of the sperm flagella.
Values are expressed as a percentage, unless specified otherwise. Values are mean ± SD; n = total number of patients in each group; n′ = number of patients used to calculate the average based on available data; NA, not available.
$Other mutations correspond to patients with mutations in cilia and flagella associated protein 43 (CFAP43) and 44 (CFAP44) and dynein axonemal heavy chain 1 (DNAH1) genes, according to Coutton et al. (2018).
(%)Reference limits (fifth centiles and their 95% CI) according to the World Health Organization standards (Cooper et al., 2010) and the distribution range of morphologically normal spermatozoa observed in 926 fertile individuals (Auger et al., 2016).
*Significant P < 0.05 (Student’s t-test).
Average semen parameters in patients mutated in FSIP2, in other xonemal-associated genes, and for the 78 subjects with multiple morphological abnormalities of the sperm flagella in the study.
Semen parameters . | MMAF with FSIP2 mutations . | MMAF other mutations$ . | Overall MMAF . | Reference limits(%) . |
---|---|---|---|---|
n = 4 . | n = 22 . | n = 78 . | ||
Mean age (years) | 46 ± 4 | 39.8 ± 7 | 41.6 ± 7.7 | NA |
(n′ = 4) | (n′ = 21) | (n′ = 77) | ||
Sperm volume (ml) | 3.1 ± 1.3 | 3.4 ± 1.2 | 3.5 ± 1.4 | 1.5 (1.4–1.7) |
(n′ = 4) | (n′ = 20) | (n′ = 75) | ||
Sperm concentration (106/ml) | 14.5 ± 23.5 | 20.1 ± 18.8 | 25.6 ± 32.1 | 15 (12–16) |
(n′ = 4) | (n′ = 20) | (n′ = 75) | ||
Total motility (1 h) | 1.5 ± 2.4 | 0.7 ± 2.4 | 3.9 ± 5.6 | 40 (38–42) |
(n′ = 4) | (n′ = 21) | (n′ = 76) | ||
Vitality | 34 ± 20.3 | 50.5 ± 22.7 | 52.7 ± 20 | 58 (55–63) |
(n′ = 4) | (n′ = 19) | (n′ = 72) | ||
Normal spermatozoa | 0 ± 0 | 0.5 ± 2.3 | 1.6 ± 2.7 | 23 (20–26) |
(n′ = 4) | (n′ = 20) | (n′ = 61) | ||
Absent flagella | 7.5 ± 5.9* | 28.1 ± 14.4 | 20.7 ± 15.7 | 5 (4–6) |
(n′ = 4) | (n′ = 15) | (n′ = 66) | ||
Short flagella | 57.5 ± 20.6 | 57.1 ± 27.9 | 43.7 ± 27.3 | 1 (0–2) |
(n′ = 4) | (n′ = 19) | (n′ = 72) | ||
Coiled flagella | 16 ± 8.3 | 10.4 ± 6.6 | 12.8 ± 9.4 | 17 (15–19) |
(n′ = 4) | (n′ = 16) | (n′ = 69) | ||
Bent flagella | NA | 8.7 ± 5.8 | 4.2 ± 8.4 | 13 (11–15) |
(n′ = 6) | (n′ = 26) | |||
Flagella of irregular caliber | 44.8 ± 31.5 | 27.9 ± 19.4 | 31.7 ± 25.1 | 2 (1–3) |
(n′ = 4) | (n′ = 15) | (n′ = 67) |
Semen parameters . | MMAF with FSIP2 mutations . | MMAF other mutations$ . | Overall MMAF . | Reference limits(%) . |
---|---|---|---|---|
n = 4 . | n = 22 . | n = 78 . | ||
Mean age (years) | 46 ± 4 | 39.8 ± 7 | 41.6 ± 7.7 | NA |
(n′ = 4) | (n′ = 21) | (n′ = 77) | ||
Sperm volume (ml) | 3.1 ± 1.3 | 3.4 ± 1.2 | 3.5 ± 1.4 | 1.5 (1.4–1.7) |
(n′ = 4) | (n′ = 20) | (n′ = 75) | ||
Sperm concentration (106/ml) | 14.5 ± 23.5 | 20.1 ± 18.8 | 25.6 ± 32.1 | 15 (12–16) |
(n′ = 4) | (n′ = 20) | (n′ = 75) | ||
Total motility (1 h) | 1.5 ± 2.4 | 0.7 ± 2.4 | 3.9 ± 5.6 | 40 (38–42) |
(n′ = 4) | (n′ = 21) | (n′ = 76) | ||
Vitality | 34 ± 20.3 | 50.5 ± 22.7 | 52.7 ± 20 | 58 (55–63) |
(n′ = 4) | (n′ = 19) | (n′ = 72) | ||
Normal spermatozoa | 0 ± 0 | 0.5 ± 2.3 | 1.6 ± 2.7 | 23 (20–26) |
(n′ = 4) | (n′ = 20) | (n′ = 61) | ||
Absent flagella | 7.5 ± 5.9* | 28.1 ± 14.4 | 20.7 ± 15.7 | 5 (4–6) |
(n′ = 4) | (n′ = 15) | (n′ = 66) | ||
Short flagella | 57.5 ± 20.6 | 57.1 ± 27.9 | 43.7 ± 27.3 | 1 (0–2) |
(n′ = 4) | (n′ = 19) | (n′ = 72) | ||
Coiled flagella | 16 ± 8.3 | 10.4 ± 6.6 | 12.8 ± 9.4 | 17 (15–19) |
(n′ = 4) | (n′ = 16) | (n′ = 69) | ||
Bent flagella | NA | 8.7 ± 5.8 | 4.2 ± 8.4 | 13 (11–15) |
(n′ = 6) | (n′ = 26) | |||
Flagella of irregular caliber | 44.8 ± 31.5 | 27.9 ± 19.4 | 31.7 ± 25.1 | 2 (1–3) |
(n′ = 4) | (n′ = 15) | (n′ = 67) |
MMAF, multiple morphological abnormalities of the sperm flagella.
Values are expressed as a percentage, unless specified otherwise. Values are mean ± SD; n = total number of patients in each group; n′ = number of patients used to calculate the average based on available data; NA, not available.
$Other mutations correspond to patients with mutations in cilia and flagella associated protein 43 (CFAP43) and 44 (CFAP44) and dynein axonemal heavy chain 1 (DNAH1) genes, according to Coutton et al. (2018).
(%)Reference limits (fifth centiles and their 95% CI) according to the World Health Organization standards (Cooper et al., 2010) and the distribution range of morphologically normal spermatozoa observed in 926 fertile individuals (Auger et al., 2016).
*Significant P < 0.05 (Student’s t-test).
As observed for the other 68 MMAF individuals of the cohort, analysis of sperm morphology from patients FSIP2_1–4 revealed very few spermatozoa with normal flagella at the expense of spermatozoa with short, absent or irregular flagella (Fig. 1). The semen parameters of the overall MMAF cohort and the detailed semen parameters of FSIP2 patients (patients FSIP2_1–4) are presented in Tables I and II. Interestingly, we observed that FSIP2 patients (FSIP2_1–4) presented a significantly lower percentage of spermatozoa with absent flagellum compared to MMAF patients with mutations in the axonemal genes DNAH1, CFAP43 or CFAP44 (t-test; P < 0.05) but no other differences were observed in the semen parameters between the two groups (Tables I and II).
FSIP2 mutations lead to severe disorganization of the FS and axonemal structure
We next studied the ultrastructure of sperm cells from patient FSIP2_4 by TEM (Fig. 3). Owing to the low sperm concentration, only a few usable images could be obtained. Longitudinal sections showed severe FS defects, which appeared completely disorganized and dysplastic (Fig. 3A and B). Outer dense fibers (ODFs) remained present whereas the mitochondrial sheath was totally absent from the midpiece and, as a consequence, the hypertrophic FS extended up to the sperm neck (Fig. 3B). Cross-sections permitting us to observe the ultrastructure of the axonemal components were also analyzed (n = 15). In the midpiece of spermatozoa from fertile controls, the axoneme is surrounded by nine ODFs and the mitochondrial sheath (Fig. 3C); while in the principal piece, the axoneme is surrounded by seven ODFs and flanked by the FS composed of two longitudinal columns connected by circumferential ribs (ODFs 3 and 8 are replaced by the two longitudinal columns) (Fig. 3D). In FSIP2 patients, 100% of the analyzed cross-sections showed a redundant and randomly arranged FS without the expected organization in longitudinal columns and circumferential ribs (Fig. 3E–H). In some principal-piece cross-sections, we counted nine ODFs indicating that the ODFs 3 and 8 were not replaced by the longitudinal columns (Fig. 3H). ODF organization was frequently altered with the presence of supernumerary or missing ODFs (Fig. 3E–G). Mitochondrial sheaths were not visible in any cross-sections. Due to limited access to patient biological material, these results, although convincing could only be obtained from a small number of sections and should be confirmed. Additional results were obtained by light microscopy which often showed a highly disorganized midpiece and IF experiments. IF experiments with anti-HSP60, which detects a mitochondrial protein located in the midpiece (Magnoni et al., 2014), showed that mitochondria are still present but are strongly disorganized and scattered all along the sperm flagellum (Supplementary Fig. S2). This suggests that the absence of the mitochondrial sheath is not due to the absence of mitochondria but may be related to the impaired caudal migration of the annulus during spermiogenesis. The axoneme also showed a constant abnormal conformation with variable degrees of defects ranging from the absence of the central pair (CP) (9 + 0 conformation) to a complete disorganization (Fig. 3E–H). In addition to these obvious flagellar defects, we also observed some severe nuclear alterations, particularly in chromatin texture (data not shown).
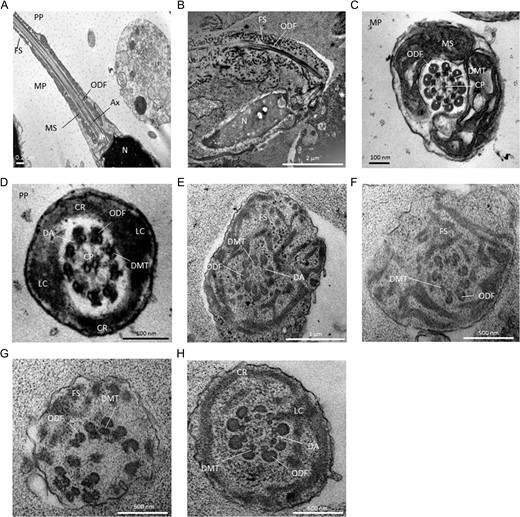
Transmission electron microscopy analyses of sperm cells from a control subject (A, C, D) and patient FSIP2_4 (B, E–H). (A) Longitudinal sections of sperm flagellum from control. In the midpiece (MP), outer dense fibers (ODF) are found longitudinally between the mitochondrial sheath (MS) and the axoneme (Ax). The fibrous sheath (FS) is found in the principal piece (PP). N, nucleus. (B) Longitudinal sections of sperm flagellum from patient FSIP2_4. We notice an abnormal flagellum with the FS which appears severly disorganized and extends up to the sperm neck. ODFs remains present whereas the MS is totally absent from the midpiece likely due to an abnormal migration of the annulus. The central axoneme is not clearly visible. (C) Cross-sections of the MP in a control. The axoneme is surrounded by nine ODFs and the MS. The axoneme is composed of nine peripheral microtubules doublets (DMTs) and a central pair (CP) of singlet microtubules. (D) Cross-sections of the PP from a fertile control. The axoneme is surrounded by seven ODFs and by the FS composed of two longitudinal columns (LC) connected by circumferential ribs (CR). DA, dynein arms. (E–H) Cross-sections of sperm flagellum from patient FSIP2_4. The FS is dysplastic, thickened and dramatically disorganized with randomly oriented fibers (E–G). When the CR seem present, we observed only one nascent and abnormal LC adjacent to doublets 3 or 8 (H). Various axonemal anomalies can be observed including the lack of the CP (E, F, H) or complete axonemal desorganization (G). Alterations of ODFs is also seen with surpernumeray (E), missing (F) or fully disorganized ODFs (G). DA are rarely visible (E, H).
FSIP2 mutations lead to the absence of AKAP4 protein and an altered distribution of the axonemal components
Despite repeated attempts, no antibodies specifically recognizing FSIP2 in human sperm cells could be obtained, precluding the immunodetection of FSIP2 protein on sperm samples from control individuals and its potential absence in FSIP2_1–4 patients. We therefore decided to analyze the distribution of various proteins of the sperm flagellum, and in particular of AKAP4, a protein described to localize to the FS and to directly interact with FSIP2 (Brown et al., 2003). We performed some IF assays on sperm cells and observed that the AKAP4 staining was totally absent from patient FSIP2_3 and FSIP2_4 whereas AKAP4 immunostaining was strong in the principal piece of the sperm flagellum of control sperm cells from fertile individual (Fig. 4). In order to determine if the loss of AKAP4 was a constant feature of the MMAF phenotype, we analyzed MMAF patients carrying mutations in CFAP43, CFAP44 and DNAH1. In these patients, AKAP4 immunostaining was comparable with that observed in fertile controls (Fig. 4), indicating that the molecular structure of the FS was not as severely altered in the sperm from MMAF patients with mutations in axoneme-related genes. We decided to further investigate the flagellum structure of FSIP2 patients by performing immunostaining with antibodies targeting different axonemal proteins. We analyzed the sperm associated antigen 6 (SPAG6), a component of the CP complex. In a fertile control, we observed that SPAG6 staining was concentrated in the principal piece of the spermatozoa whereas staining was absent or strongly reduced in FSIP2-mutated patients with an abnormal and diffuse pattern concentrated on the flagellum’s proximal part (Supplementary Fig. S3A). Moreover, staining of dynein axonemal heavy chain 5 (DNAH5) and dynein axonemal light intermediate chain 1 (DNALI1), two proteins located in the outer dynein arms and inner dynein arms, respectively, were absent in FSIP2_3 and FSIP2_4 patients demonstrating that the whole axonemal structure was strongly affected by the presence of the FSIP2 mutations (Supplementary Fig. S3B, C).
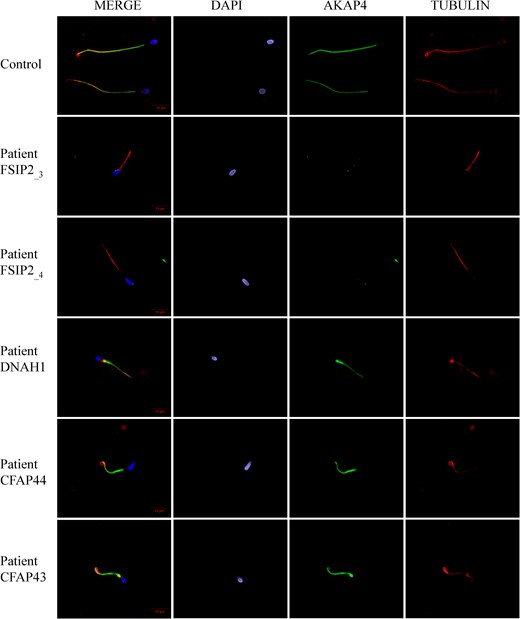
A-kinase anchoring protein 4 is absent in sperm cells from patients with FSIP2 mutations. Sperm cells from a fertile control, the two patients FSIP2_3 and FSIP2_4, and three other patients mutated in dynein axonemal heavy chain 1 (DNAH1) gene, and in the cilia and flagella associated protein 43 (CFAP43) and 44 (CFAP44) genes, respectively, were stained with anti-A-kinase anchoring protein 4 (AKAP4: green) and anti-acetylated tubulin (red) antibodies. DNA was counterstained with DAPI showing sperm nuclear DNA (blue). The AKAP4 immunostaining is concentrated in the PP of the spermatozoa from the fertile control and the three patients mutated in DNAH1, CFAP43 and CFAP44. Conversely, AKAP4 staining is absent in sperm from the two patients FSIP2_3 and FSIP2_4. Scale bar 10 μm.
Discussion
The study presented here allowed us to demonstrate that the absence of functional FSIP2, a protein of the sperm flagella FS, induced a MMAF phenotype. Logically, TEM analyses of sperm from our FSIP2 patients revealed a severe dysplasia of the FS, which appeared extremely disorganized and hypertrophic. Such FS defects were previously reported and studied in patients with a MMAF-like phenotype initially described as DFS (Chemes et al., 1987; Chemes and Rawe, 2003, 2010; Chemes and Alvarez Sedo, 2012). These FS defects do not seem to be as marked in sperm cells from other MMAF patients with mutations in the three axonemal genes DNAH1, CFAP43 and CFAP44 (Ben Khelifa et al., 2014; Coutton et al., 2015, 2018; Tang et al., 2017). The FS is a cytoskeletal structure located in the principle piece of the sperm flagellum, which seems to be unique to amniote sperm flagellum (Fawcett, 1970). This is consistent with our expression data showing a specific expression of FSIP2 in the testis with no detectable expression in the other ciliated tissues (Supplementary Fig. S1). In the principal piece, the FS is formed by two longitudinal columns connected by circumferential ribs (Eddy et al., 2003). The FS is believed to serve as a scaffold for numerous proteins including glycolytic enzymes or signaling protein and to play a role in the regulation of sperm motility but also in sperm maturation, capacitation and hyperactivation (Eddy et al., 2003; Eddy, 2007). An important part of the enzymatic and energy metabolism of the principal piece is likely regulated by the cAMP-dependent protein kinase A (PKA) which is anchored in the FS through different AKAPs (Carr and Newell, 2007). AKAPs are a family composed of around 50 scaffold proteins which confine various enzymes including kinases, phosphatases and phosphodiesterases and their corresponding enzymatic activities to specific intracellular locations (Kumar et al., 2017). In sperm, six AKAPs have been identified, three of which (AKAP3, AKAP4 and AKAP14) are located in the FS (Fiedler et al., 2008). FSIP2 was shown to bind to AKAP4, the most abundant AKAP of the FS, which was described to be important for completing FS assembly (Brown et al., 2003; Nipper et al., 2006). Furthermore, the region where FSIP2 likely docks onto AKAP4 encompasses the RIa/RIIa-dual domain, which is the docking domain of PKA onto the AKAP proteins, suggesting that FSIP2 could have a role in regulating PKA anchoring to AKAP4 (Turner et al., 1998; Brown et al., 2003; Welch et al., 2010). Here we showed that in FSIP2-deficient patients, AKAP4 was absent from the sperm flagellum contrary to what was observed in the other patients with a similar phenotype owing to mutations in axonemal-associated MMAF genes (Fig. 4). Interestingly, it has been previously reported that AKAP4-deficient mice also had shortened flagella with an irregular diameter of the principal piece (Miki et al., 2002) as observed in our FSIP2-mutated patients (Fig. 1). In addition, the only patient reported with a partial deletion of AKAP4 (Baccetti et al., 2005), also presented spermatozoa with extremely short tails and severe FS dysplasia.
In addition to the severe abnormalities of the peri-axonemal structures described above, sperm from patients with FSIP2 mutations also displayed some axonemal anomalies including the absence of the CP and of all the inner/outer dynein arms (Figs 3 and 4). Such axonemal defects were also described in sperm cells from patients described as DFS (Chemes and Rawe, 2003, 2010). Moreover, when discussing the phenotype of their AKAP4 deleted patient, Baccetti et al. (2005) also reported that their patient’s sperm had an altered axonemal structure with missing dynein arms and CP. They however did not present any sperm tail cross-sections, making a reliable comparison with our patients difficult. Since the axoneme is assembled soon after the completion of meiosis and appears before the FS (Horowitz et al., 2005), we can presume that these axonemal defects are secondary to FS misassembly or disorganization. This assumption is supported by studies on testicular biopsies from DFS patients showing that the phenotype arises during late spermiogenesis (Ross et al., 1973; Barthelemy et al., 1990; Rawe et al., 2002; Chemes and Rawe, 2010). Altogether these elements clearly indicate that the patients whose MMAF is caused by FSIP2 mutations present a DFS ultrastructural phenotype.
Absence of FSIP2 and consequently of AKAP4 could impede the correct localization of functionally important FS-associated proteins that are critical for the maintenance of sperm function and structure, such as PKA or glycolytic enzymes (Miki et al., 2002; de Boer et al., 2015). Additionally, the important disorganization of the FS and the failure to form the longitudinal columns may also alter the direct interaction of microtubule doublets (DMTs) 3 and 8 with the FS in the principal piece and weaken the stability of the axonemal structures leading to the total destabilization of the axoneme. We have just demonstrated that CFAP43 and CFAP44 likely link the axoneme with the extra-axonemal components (Coutton et al., 2018). This suggests that most of the flagellar structures are tightly connected and defects in any of these structures may alter the whole process of flagellar genesis and produce a MMAF phenotype. Intriguingly, we observed that the sperm phenotype induced by the presence of FSIP2 mutations is more severe than what was described for Akap4 mutant mice (Miki et al., 2002), where only the FS was described as being affected. This could indicate that FSIP2 is not only a structural protein of the FS but that it may be involved in the intraflagellar transport of several components, potentially including AKAP4. Further studies are now required to define the role of the different proteins found to be associated with a MMAF phenotype, and to identify and characterize sub-phenotypes induced by the different molecular defects. Overall, this demonstrates the crucial importance of FSIP2 in maintaining the whole structure and function of the sperm flagellum.
WES analysis permitted the identification of four FSIP2 loss of function variants. All were indels including nucleotide deletions, duplications or insertions. No obvious phenotype differences were observed among these four patients, concordant with the fact that all identified mutations are expected to induce the production of a truncated non-functional protein. Interestingly we noted that patients with FSIP2 mutations presented a significant lower rate of spermatozoa with absent flagella compared to patients with an identified mutation in other MMAF genes suggesting that FSIP2 mutations might not alter the head-flagella junction as severely as mutations in axonemal-related genes. Moreover, although not statistically significant, FSIP2 patients had a higher rate of flagella with an irregular diameter, which is consistent with the overabundance and disorganization of the FS (Chemes and Rawe, 2010). Several questions remain concerning the prognosis of ICSI using sperm cells from FSIP2 patients. Successful application of ICSI in patients with MMAF has been previously described, including patients carrying mutations in DNAH1 (Olmedo et al., 2000; Chemes and Rawe, 2003; Chemes and Alvarez Sedo, 2012; Dávila Garza and Patrizio, 2013; Coutton et al., 2015; Wambergue et al., 2016). However, so far it remains difficult to predict the prognosis for patients carrying FSIP mutations, as ICSI success rates have been described to be influenced by the type of ultrastructural flagellar defects (Mitchell et al., 2006; Fauque et al., 2009; Coutton et al., 2015). Additional correlation studies should now be performed to take into account the individual genotypes in the counseling of MMAF patients.
A previous study of the cohort presented here permitted the identification of 22 patients (28.2%) with mutations in DNAH1, CFAP43 and CFAP44 (Coutton et al., 2018) and two patients with CFAP69 mutations (Dong et al., 2018). In the present study, we identified four additional patients with FSIP2 mutations and established FSIP2 as a new recurrent genetic cause of MMAF present in more than 5% of our cohort. The overall rate of a positive molecular diagnosis for this cohort is therefore 36% (28/78) which is close to the diagnosis rate found in other diseases with heterogeneous genetic etiologies (Farwell et al., 2015; Fogel et al., 2016). Our data thus demonstrate the clinical utility of WES for the identification of genetic causes responsible for male infertility due to a MMAF phenotype. In-depth bioinformatic analyses of WES data from the remaining 50 undiagnosed individuals from this cohort, together with the inclusion of additional MMAF patients, will likely improve the diagnosis yield and bring to light other rare genetic defects inducing a MMAF phenotype.
Acknowledgements
We thank all patients and control individuals for their participation.
Authors’ roles
C.C., P.F.R., C.A., M.B. and A.T. analyzed the data and wrote the article; T.K., Z.K., N.T.M., M.B., A.A.Y., P.F.R. and C.C. performed and analyzed genetic data; G.M., A.S. performed IF experiments; K.P.G., A.B. and G.M. performed EM experiments, sperm analyses; R.Z., V.S. provided clinical samples and data; C.C., P.F.R., C.A. and G.M. designed the study, supervised all molecular laboratory work, had full access to all of the data in the study and takes responsibility for the integrity of the data and its accuracy. All authors contributed to the report.
Funding
The ‘MAS-Flagella’ project financed by the French Agence nationale de la Recherche (ANR) and the Direction générale de l'offre de soins (DGOS) for the program Programme de Recherche Translationnelle en Santé (PRTS) 2014 and the ‘Whole genome sequencing of patients with Flagellar Growth Defects (FGD)’ project financed by the Fondation Maladies Rares for the program Séquençage à haut débit 2012.
Conflict of interest
The authors have declared that no conflict of interest exists.
References
Author notes
Pierre F. Ray and Charles Coutton Equal contribution, the authors should both be regarded as last authors.