-
PDF
- Split View
-
Views
-
Cite
Cite
Maria Giulia Minasi, Francesco Fiorentino, Alessandra Ruberti, Anil Biricik, Elisabetta Cursio, Ettore Cotroneo, Maria Teresa Varricchio, Matteo Surdo, Francesca Spinella, Ermanno Greco, Genetic diseases and aneuploidies can be detected with a single blastocyst biopsy: a successful clinical approach, Human Reproduction, Volume 32, Issue 8, August 2017, Pages 1770–1777, https://doi.org/10.1093/humrep/dex215
- Share Icon Share
Abstract
Can simultaneous detection of aneuploidies and genetic diseases or chromosomal aberrations in blastocysts reduce the chance of transferring embryos with low implantation potential, guaranteeing good clinical outcomes?
The screening for chromosomal aneuploidies revealed that 50.6% of blastocysts diagnosed free of genetic disease or balanced, were aneuploid, therefore avoiding the transfer of blastocysts potentially resulting in implantation failures, miscarriages, or in some cases, in health affected live births.
PGD is applied in patients at risk of transmitting genetically inheritable diseases to their offspring. It has been demonstrated that aneuploidies can involve chromosomes other than those investigated with PGD, affecting embryo implantation competence. Performing the biopsy at blastocyst level produces higher clinical outcomes allowing a more accurate diagnosis, compared to blastomere biopsy.
This consecutive case series study was performed from October 2011 to May 2016. Clinical and biological outcomes from 1122 blastocysts obtained in 304 PGD cycles for monogenic diseases (N = 163) or chromosomal rearrangements (N = 141) were analyzed. When the blastocyst resulted transferable after the PGD analysis or chromosomal rearrangement analysis, its ploidy status by mean of preimplantation genetic screening (PGS) was also detected using the same biopsy sample. Mean female age was 35.4 ± 4.2 years old. All biopsies were performed at blastocyst stage and analyzed by Whole Genome Amplification (WGA) followed by PCR for monogenic diseases, and by array-comparative genotype hybridization (array-CGH) for all cycles.
All mature oocytes retrieved were injected and cultured individually until the blastocyst stage at 37°C, 6% CO2, 5% O2. When the blastocyst was formed, it was biopsied and vitrified, awaiting the genetic results. The frozen–thawed embryo transfer was performed in a subsequent cycle. In some cases, when the blastocyst was obtained within the morning of Day 5 of culture, it had been maintained in culture and transferred on Day 6, after receiving the genetic report.
A total of 2809 (2718 fresh and 91 frozen–thawed) mature oocytes were injected with a fertilization rate of 75.5% (N = 2120), leading to the development of 2102 embryos. A further 24 frozen embryos, previously vitrified without any genetic testing, were successfully warmed for genetic screening. A total of 2126 embryos were cultured with a blastocyst formation rate of 52.8% (N = 1122); all of them were biopsied from Day 4 to Day 7 of culture. After the genetic analysis, 309 (27.5%) blastocysts resulted transferable, both for monogenic disease or translocation and for their ploidy status, 42 were diploid/aneuploid mosaic, 55 were no result and 716 were not transferable, due to genetic disease or chromosomal rearrangement and/or for their ploidy status. Of note, 316 (50.6% of transferable blastocysts after PGD and 28.2% of total number of biopsied blastocysts) of the blastocysts resulted healthy for the genetic disease or chromosomal rearrangement were aneuploid. Out of 304 PGD/PGS cycles performed, 28.6% (N = 87) resulted in no-transferable blastocysts after only PGD analysis; this percentage increased to 39.8% (N = 121) when also PGS was carried out (Mc Nemar test P < 0.001). A total of 202 embryo-transfers were performed, 53 fresh and 149 cryopreserved, in which 218 healthy or carrier euploid blastocysts were transferred. Clinical pregnancy, implantation and miscarriage rates were 49.0, 47.7 and 9.9%, respectively. To date, 66 deliveries occurred with 70 healthy babies born and 13 pregnancies are still ongoing. Finally, 91 euploid healthy blastocysts are still cryopreserved waiting to be transferred.
A higher than expected cycle cancellation rate could be found due to the double genetic analysis performed. For this reason, particular care should be taken in drafting and explaining informed consent, in order to avoid patient drop out.
When the biopsy has to be performed in order to prevent the transmission of an inheritable disease, it should be mandatory to analyze also the genetic status of the blastocyst, avoiding useless embryo-transfers in this particular category of patients. In our study, 316 aneuploid healthy blastocysts could have been transferred without performing PGS, leading to implantation failures, miscarriages, or in some cases, to live births affected by different syndromes.
No specific funding was obtained for this study. None of the authors have any competing interests to declare.
Not applicable.
Introduction
To date, in Europe the average clinical pregnancy rates per transfer reported with fresh and frozen–thawed embryos for a general population are ~32 and 22%, respectively. These outcomes are still quite low and should be indeed greatly improved (Evans et al., 2014). The reasons for such inadequate outcomes could be ascribed to embryo aneuploidy rates that in human can reach 60% (Fragouli et al., 2011) and that are highly related to implantation failure and miscarriage (Grifo et al., 2013; Harton et al., 2013). PGD is an invasive method for embryo selection. Initially, it was used in fertile patients with high risk of transmitting genetically inheritable diseases to their offspring (Handyside et al., 1990). Protocols for genotyping single cells for monogenic disorders typically use PCR (Munnè, 2002). Later, the introduction of other techniques capable of analyzing 24 chromosomes, extended embryo screening to infertile patients willing to investigate the genetic status of their embryos before transfer (preimplantation genetic screening (PGS)) (Harper and Harton, 2010; Harper et al., 2010; Gutierrez-Mateo et al., 2011). However, considerable controversy still remains about the clinical and economic effectiveness of this approach and, in particular, on the efficiency of performing PGS on a routine basis for all categories of patients (Sermon et al., 2016). The first successful attempts to perform a double factor analysis were reported by Obradors and collaborators, with the aim to improve implantation rates by selecting potentially euploid embryos free of mutations responsible for cystic fibrosis (Obradors et al., 2008) or Von Hippel–Lindau syndrome (Obradors et al., 2009). However in both case reports, a very laborious double sequence of biopsies was performed: first PGS was carried out by means of array-comparative genotype hybridization (CGH) on oocyte polar bodies, then PGD was performed by means of PCR on Day 3 blastomeres. A similar procedure was applied by Rechitsky et al. (2013) in 96 cycles resulting in the transfer of 153 unaffected aneuploidy-free embryos and reporting 32 healthy live births. It has been reported that trophoectoderm (TE) biopsy does not impair human embryonic implantation potential and is much less invasive compared to blastomere biopsy (Scott et al., 2013a; Greco et al., 2013). The analysis of morphokinetic parameters showed that blastomere biopsy interferes with the dynamics of embryo development, delaying compaction and blastulation and reducing the implantation rate (Bar-El et al., 2016). A reasonable explanation for a lower disturbance of TE biopsy compared to blastomere removal could be found in the smaller proportion of cells and in the type of cells removed, that are only extra-embryonic. In addition, it has been observed that performing the TE biopsy at the blastocyst stage produces higher ongoing and clinical pregnancy rates, compared to cleavage stage biopsy (Hodes-Wertz et al., 2012; Harton et al., 2013; Scott et al., 2013b; Alder et al., 2014; Minasi and Greco, 2014). Importantly, concordance between the genetic results obtained from TE and inner cell mass (ICM) samples from the same blastocyst has been reported, demonstrating that TE karyotype is an excellent predictor of ICM karyotype (Johnson et al., 2010). This indicates that it is possible to accurately assess the chromosome constitution of a blastocyst by examining TE cells (Fragouli et al., 2008; Johnson et al., 2010). Preimplantation genetic diagnosis is now a well-established worldwide procedure that theoretically could be applied for the detection of any genetic disease (Fiorentino et al., 2011; Capalbo et al., 2016). Several studies highlighted that the presence of aneuploidies involving chromosomes other than those investigated with PGD can affect embryo implantation potential (Fiorentino et al., 2013; Daina et al., 2015; Zimmerman et al., 2016). Therefore, the focus in the PGD field has now shifted toward TE biopsy and the use of PGS, in order to assess all chromosomes and provide a more accurate assessment of the embryo's reproductive potential. For these reasons, the realization of a safe method combining PGD and PGS in order to obtain information regarding both ploidy and the genetic status of an embryo with a single TE biopsy, has become one of the most attractive challenge in the field of ART. The aim of the present study was to investigate whether a single TE biopsy is efficient in detecting either genetic diseases or chromosomal rearrangements (such as translocations, inversions or deletions) and the ploidy status of embryos, limiting the negative effect on implantation potential and guaranteeing good clinical outcomes in patients who cannot avoid PGD for genetical reasons.
Materials and Methods
This consecutive case series study was performed from October 2011 to May 2016. Clinical and biological outcomes from 1122 blastocysts obtained in 304 PGD cycles for monogenic diseases or chromosomal rearrangements in 227 couples were analyzed. A list of the specific single gene disorders and chromosomal abnormalities is reported in Supplementary Table S1. Mean female and male ages were 35.4 ± 4.2 and 38.1 ± 5.1 years old, respectively. In particular, 163 and 141 cycles were performed analyzing 638 and 484 blastocysts in couples carrying single gene disease or chromosomal rearrangement, respectively (Table I). Patients were counseled by a clinical geneticist of Genoma Group Center, who first assessed the feasibility of carrying out the diagnosis of the genetic disease at single cell level. Written informed consent was obtained from all couples. A more detailed ‘Material and Methods’ section is available online as Supplementary Material.
Biological outcomes of PGD + PGS cycles performed from October 2011 to October 2016.
. | Monogenic disease . | Chromosomal rearrangement . | Total . |
---|---|---|---|
Number of cycles | 163 | 141 | 304 |
Patients | 131 | 96 | 227 |
Female mean age | 35.8 ± 3.8 | 34.8 ± 4.5 | 35.4 ± 4.2 |
Male mean age | 38.6 ± 5.3 | 37.4 ± 4.8 | 38.1 ± 5.1 |
CCOCs | 1847 | 1734 | 3581 |
MII | 1404 | 1314 | 2718 |
Thawed oocytes survived | 63 | 28 | 91 |
Total injected oocytes (fresh and cryopreserved) | 1467 | 1342 | 2809 |
Zygotes | 1126 (76.8%) | 994 (74.1%) | 2120 (75.5%) |
Embryos obtained | 1121 (99.6%) | 981 (98.7%) | 2102 (99.2%) |
Thawed embryos | 13 | 11 | 24 |
Blastocysts obtained | 638 (56.3%) | 484 (48.8%) | 1122 (52.8%) |
Transferable blastocysts (PGD + PGS) | 181 (28.4%) | 128 (26.4%) | 309 (27.5%) |
Mosaic blastocysts | 24 (3.8%) | 18 (3.7%) | 42 (3.7%) |
Not transferable blastocysts | 394 (61.8%) | 322 (66.5%) | 716 (63.8%) |
due only to aneuploidies | 179 | 137 | 316 |
No result blastocysts | 39 (6.1%) | 16 (3.3%) | 55 (4.9%) |
Cycles without transferable blastocysts | 64 (39.3%) | 57 (40.4%) | 121 (39.8%) |
. | Monogenic disease . | Chromosomal rearrangement . | Total . |
---|---|---|---|
Number of cycles | 163 | 141 | 304 |
Patients | 131 | 96 | 227 |
Female mean age | 35.8 ± 3.8 | 34.8 ± 4.5 | 35.4 ± 4.2 |
Male mean age | 38.6 ± 5.3 | 37.4 ± 4.8 | 38.1 ± 5.1 |
CCOCs | 1847 | 1734 | 3581 |
MII | 1404 | 1314 | 2718 |
Thawed oocytes survived | 63 | 28 | 91 |
Total injected oocytes (fresh and cryopreserved) | 1467 | 1342 | 2809 |
Zygotes | 1126 (76.8%) | 994 (74.1%) | 2120 (75.5%) |
Embryos obtained | 1121 (99.6%) | 981 (98.7%) | 2102 (99.2%) |
Thawed embryos | 13 | 11 | 24 |
Blastocysts obtained | 638 (56.3%) | 484 (48.8%) | 1122 (52.8%) |
Transferable blastocysts (PGD + PGS) | 181 (28.4%) | 128 (26.4%) | 309 (27.5%) |
Mosaic blastocysts | 24 (3.8%) | 18 (3.7%) | 42 (3.7%) |
Not transferable blastocysts | 394 (61.8%) | 322 (66.5%) | 716 (63.8%) |
due only to aneuploidies | 179 | 137 | 316 |
No result blastocysts | 39 (6.1%) | 16 (3.3%) | 55 (4.9%) |
Cycles without transferable blastocysts | 64 (39.3%) | 57 (40.4%) | 121 (39.8%) |
CCOC: cumulus-corona-oocyte complex; MII: metaphase II; PGD: preimplantation genetic diagnosis; PGS: preimplantation genetic screening.
Biological outcomes of PGD + PGS cycles performed from October 2011 to October 2016.
. | Monogenic disease . | Chromosomal rearrangement . | Total . |
---|---|---|---|
Number of cycles | 163 | 141 | 304 |
Patients | 131 | 96 | 227 |
Female mean age | 35.8 ± 3.8 | 34.8 ± 4.5 | 35.4 ± 4.2 |
Male mean age | 38.6 ± 5.3 | 37.4 ± 4.8 | 38.1 ± 5.1 |
CCOCs | 1847 | 1734 | 3581 |
MII | 1404 | 1314 | 2718 |
Thawed oocytes survived | 63 | 28 | 91 |
Total injected oocytes (fresh and cryopreserved) | 1467 | 1342 | 2809 |
Zygotes | 1126 (76.8%) | 994 (74.1%) | 2120 (75.5%) |
Embryos obtained | 1121 (99.6%) | 981 (98.7%) | 2102 (99.2%) |
Thawed embryos | 13 | 11 | 24 |
Blastocysts obtained | 638 (56.3%) | 484 (48.8%) | 1122 (52.8%) |
Transferable blastocysts (PGD + PGS) | 181 (28.4%) | 128 (26.4%) | 309 (27.5%) |
Mosaic blastocysts | 24 (3.8%) | 18 (3.7%) | 42 (3.7%) |
Not transferable blastocysts | 394 (61.8%) | 322 (66.5%) | 716 (63.8%) |
due only to aneuploidies | 179 | 137 | 316 |
No result blastocysts | 39 (6.1%) | 16 (3.3%) | 55 (4.9%) |
Cycles without transferable blastocysts | 64 (39.3%) | 57 (40.4%) | 121 (39.8%) |
. | Monogenic disease . | Chromosomal rearrangement . | Total . |
---|---|---|---|
Number of cycles | 163 | 141 | 304 |
Patients | 131 | 96 | 227 |
Female mean age | 35.8 ± 3.8 | 34.8 ± 4.5 | 35.4 ± 4.2 |
Male mean age | 38.6 ± 5.3 | 37.4 ± 4.8 | 38.1 ± 5.1 |
CCOCs | 1847 | 1734 | 3581 |
MII | 1404 | 1314 | 2718 |
Thawed oocytes survived | 63 | 28 | 91 |
Total injected oocytes (fresh and cryopreserved) | 1467 | 1342 | 2809 |
Zygotes | 1126 (76.8%) | 994 (74.1%) | 2120 (75.5%) |
Embryos obtained | 1121 (99.6%) | 981 (98.7%) | 2102 (99.2%) |
Thawed embryos | 13 | 11 | 24 |
Blastocysts obtained | 638 (56.3%) | 484 (48.8%) | 1122 (52.8%) |
Transferable blastocysts (PGD + PGS) | 181 (28.4%) | 128 (26.4%) | 309 (27.5%) |
Mosaic blastocysts | 24 (3.8%) | 18 (3.7%) | 42 (3.7%) |
Not transferable blastocysts | 394 (61.8%) | 322 (66.5%) | 716 (63.8%) |
due only to aneuploidies | 179 | 137 | 316 |
No result blastocysts | 39 (6.1%) | 16 (3.3%) | 55 (4.9%) |
Cycles without transferable blastocysts | 64 (39.3%) | 57 (40.4%) | 121 (39.8%) |
CCOC: cumulus-corona-oocyte complex; MII: metaphase II; PGD: preimplantation genetic diagnosis; PGS: preimplantation genetic screening.
Ovarian stimulation, oocyte denudation, insemination and culture
Controlled ovarian stimulation was performed using recombinant follicle stimulating hormone (FSH. Gonal F, Merck Serono, Geneva, Switzerland) and a gonadotrophin-releasing hormone (GnRH) agonist suppression protocol (short or long) or GnRH antagonist flexible protocol according to ovarian reserve and anti-mullerian hormone (AMH) values, as described elsewhere (Greco et al., 2014). When at least three follicles reached 19 mm in diameter, hCG (Gonasi, 10.000 IU, IBSA, Lodi, Italy) was administered by intramuscular injection. For all oocytes, denudation was performed by brief exposure to 20 IU/ml hyaluronidase (Hyaluronidase 80 U/mL in HEPES-HTF, Sage, USA) in HEPES-buffered medium (Quinn's Advantage Medium with HEPES, SAGE, USA) under oil (Oil for tissue culture, SAGE, USA). Subsequently, oocytes were gently aspirated in and out of a plastic pipette (Flexipet, 170 and 140 μm i.d., COOK, Australia) to allow the complete removal of cumulus and corona cells. All embryos were cultured individually until blastocyst stage under oil, in sequential media (Quinn's Advantage Medium, SAGE, USA), at 37°C, 5% O2, 6% CO2 (Minasi et al., 2016).
Blastocyst biopsy, vitrification and warming
On Day 3, a non-contact diode laser was used to create a circular 6–9 μm diameter opening in the zona pellucida of cleavage stage embryos, in order to allow the blastocyst cells to herniate at the time of expansion. All biopsies were performed at blastocyst stage. On the day of biopsy, 5–10 TE cells were gently aspirated with a biopsy pipette (COOK, Ireland Ltd, Limerick, Ireland) followed, if necessary, by a laser assisted cut. The obtained TE cells were washed in sterile phosphate-buffered saline (PBS) solution and then sent to Genoma laboratory for the genetic analysis (Greco et al., 2014). Vitrification was carried out with the use of the Kuwayama protocol with Cryotop (Kuwayama, 2007). Blastocysts were individually loaded onto Cryotops in a volume of <0.1 μL and quickly plunged into liquid nitrogen. On the day of transfer, blastocysts were transferred 1–2 h post-warming (Minasi et al., 2016).
Pre-clinical work-up and whole genome amplification
Determination or confirmation of the genetic status of the couples was performed as previously described (Fiorentino et al., 2003). PCR amplification of each region of interest was performed using the outer oligonucleotide primers reported elsewhere (Fiorentino et al., 2003, 2006). For whole genome amplification (WGA), cells were first lysed and genomic DNA was randomly fragmented and amplified using the SurePlex DNA Amplification System (Illumina Inc., San Diego, USA), according to the manufacturer's protocol. This proprietary single tube technology is based on random fragmentation of genomic DNA and subsequent amplification by PCR utilizing flanking universal priming sites, as previously described (Alfarawati et al., 2011; Fiorentino et al., 2011). To assess the success of the amplification, 5 μL of each amplified sample plus 5 μL gel loading buffer were examined by electrophoresis on a 1.5% agarose 1x Tris/borate/EDTA (TBE) gel. The samples having very low DNA concentrations were excluded from the following experiments and a new biopsy from corresponding embryos was requested to repeat WGA.
Multiplex PCR
DNA analysis for monogenic diseases was performed through multiplex PCR starting from WGA products. The technical details of the PCR protocols used for monogenic disease have been described elsewhere (Fiorentino et al., 2003). PCR conditions used were reported earlier (Fiorentino et al., 2003, 2006). When a blastocyst resulted transferable after the PGD analysis, its ploidy status was also detected by means of array-CGH based PGS using the same WGA products.
Array-CGH analysis
WGA products were processed with 24sure V3 microarrays (Illumina, Inc.), according to the manufacturer's protocol. Briefly, amplified samples, controls and some reference DNAs (Illumina, Inc.) were labeled with Cy3 and Cy5 fluorophores using random primers of the 24sure V3 Pack (Illumina, Inc.). Labeled DNA was resuspended in dextran sulfate hybridization buffer and hybridized under cover slips to 24sure V3 slides. A laser scanner was used to excite the hybridized fluorophores read and store the resulting images of the hybridization, as described elsewhere (Fiorentino et al., 2014). The analysis of 24sure single channel experiments was fully automated and proceeded in a similar way to all BlueGnome microarrays. The software automatically combines the data from the single channel sample experiments with both male and female references from the hybridized reference subarrays, to produce a single fused result compared with a sex matched and a mismatched reference. To pass hybridization quality control, female samples hybridized with a male reference DNA (sex mismatch) had to show a consistent gain on the X chromosome and a consistent loss of the Y chromosome (Gutierrez-Mateo et al., 2011; Fiorentino et al., 2011).
Classification of the results
For the array-CGH approach, abnormalities are detected as copy number imbalances, when the ratio of a test sample to reference deviates from 1:1 for a series of data points. Embryos were diagnosed as ‘normal/balanced’ if the array-CGH plot generated showed no gain or loss greater than 3 × SD of autosome. Embryos were diagnosed as ‘unbalanced’ if the array-CGH results showed a 3 × SD of autosome (Gutierrez-Mateo et al., 2011). A trisomy (partial or full) is detected as a shift of the clones for the specific chromosome towards the green line (gain). On the contrary, a monosomy (partial or full) is identified as a shift towards the red line (loss). An ‘inconclusive’ diagnosis was assigned for those embryos where the signal pattern was not a clear-cut normal result (Fiorentino et al., 2006).
Embryo transfer
Single or double embryo transfers, of either fresh or cryopreserved embryos, were performed. All transfer procedures were carried out with the use of a catheter (Wallace, Smits-Medical, Dublin, Ireland) under direct ultrasound guidance as previously described (Brown et al., 2016). No blastocyst transfer was done with endometrium thickness of <7 mm. Within healthy or carrier blastocysts for the genetic disease, only euploid blastocysts were selected for transfer.
Statistical analysis
Quantitative data are shown by mean ± standard deviation (SD). Categorical data are presented as numbers and percentages. Chi square test, or Fisher's exact test when necessary, was used to compare categorical data. Frequencies to have not transferable blastocysts after PGD and after PGD + PGS were compared by Mc Nemar test. Analysis was performed by STATA 14.2 (Stata; Data Analysis and Statistical Software,TX, USA); P < 0.05 was considered statistically significant.
Ethical approval
All the procedures reported in this study are routinely applied in our infertility center. For this reason, the Institutional Ethics Committee of the European Hospital approved the present study proposal in accordance with the Helsinki Declaration. An informed consent was signed from all the patients enrolled in this study.
Results
General results are shown in Table I. A total of 2809 (2718 fresh and 91 frozen–thawed) mature oocytes were injected with a fertilization rate of 75.5% (N = 2120), leading to the development of 2102 embryos. Other 24 frozen embryos, previously vitrified without any genetic testing, were successfully warmed. A total of 2126 embryos were cultured with a blastocyst formation rate of 52.8% (N = 1122); all of them were biopsied from Day 4 to Day 7 of culture. A higher blastocyst formation rate was obtained in monogenic disease compared to chromosomal rearrangement cycles (56.3 vs 48.8%, P < 0.001, respectively). After the genetic analysis, 309 (27.5%) blastocysts resulted transferable, in total for monogenic disease or chromosomal rearrangement and for their ploidy status, 42 (3.7%) were mosaic diploid/aneuploid, 55 (4.9%) had no conclusive result and 716 (63.8%) were not transferable, being affected by a genetic disease or a chromosomal abnormality and/or for their ploidy status. Of note, 35.7% (N = 400) of the blastocysts were not transferable after the PGD analysis and this percentage increased to 63.8% (N = 716) when they were also analyzed by means of PGS (Mc Nemar test P < 0.001; Figure 1A). Furthermore, 316 blastocysts (50.6% of transferable blastocysts after PGD and 28.2% of total number of biopsied blastocysts) resulted healthy for the examined genetic disease or for the parental chromosomal abnormality were however aneuploid. Out of the 304 PGD/PGS cycles, 39.8% (N = 121) resulted with affected and/or aneuploid blastocysts only and thus without the possibility to perform any embryo replacement (Table I). The percentage of cycles without available blastocysts for transfer was 28.6% (N = 87) after PGD analysis and another 11.2% (N = 34) of the cycles were canceled after PGS analysis, resulting in a significant increase in cycles without transferable blastocysts (Mc Nemar test P < 0.001; Figure 1B). Clinical results are shown in Table II. A total of 202 embryo transfers were performed in 167 patients, 53 fresh and 149 cryopreserved, where 218 healthy or carrier euploid blastocysts were transferred. To date, 66 deliveries occurred with 70 healthy babies born; 35 males and 35 females; 13 pregnancies are still ongoing and 91 euploid healthy blastocysts are still cryopreserved waiting to be transferred. A higher percentage of premature babies (≤37 weeks of gestation and/or ≤2500 g at birth) was found in cycles performed for chromosomal abnormality reasons (28.6%) compared to monogenic disorders (7.1%, P = 0.022). No significant differences were detected in the gender of the newborns. No adverse outcomes, such as perinatal deaths or birth defects, were obtained (Table II). A comparison between fresh and cryopreserved embryo transfer outcomes is reported in Supplementary material(Supplementary Table S2).
Clinical outcomes of PGD + PGS cycles performed from October 2011 to October 2016.
. | Monogenic disease . | Chromosomal rearrangement . | Total . |
---|---|---|---|
Thawed blastocysts | 92 | 70 | |
Survived blastocysts | 89 (96.7%) | 69 (98.6%) | – |
Total ET (procedures) | 120 | 82 | 202 |
Total ET (patients) | 101 | 66 | 167 |
(SET/DET) | (112/8) | (74/8) | (186/16) |
Transferred blastocysts | 128 | 90 | 218 |
Positive tests | 73 (60.8%) | 44 (53.7%) | 117 (57.9%) |
Biochemical pregnancies | 11 (9.2%) | 7 (8.5%) | 18 (8.9%) |
Clinical pregnancies | 62 (51.7%) | 37 (45.1%) | 99 (49.0%) |
Miscarriages | 15 (12.5%) | 5 (6.1%) | 20 (9.9%) |
Ectopic pregnancies | 1 | 0 | 1 |
Ongoing pregnancies | 6 | 7 | 13 |
Fetal heartbeats | 64 (50.0%) | 40 (44.4%) | 104 (47.7%) |
Deliveries | 41 (34.2%) | 25 (30.5%) | 66 (32.7%) |
Singleton/twin | 40/1 | 22/3 | 62/4 |
Spontaneus deliveries | 17 (41.5%) | 8 (32.0%) | 25 (37.9%) |
Cesarean deliveries | 24 (58.5%) | 17 (68.0%) | 41 (62.1%) |
Babies born (M/F) | 42 (18/24) | 28 (17/11) | 70 (35/35) |
Premature babies | 3 (7.1%) | 8 (28.6%) | 11 (15.7%) |
. | Monogenic disease . | Chromosomal rearrangement . | Total . |
---|---|---|---|
Thawed blastocysts | 92 | 70 | |
Survived blastocysts | 89 (96.7%) | 69 (98.6%) | – |
Total ET (procedures) | 120 | 82 | 202 |
Total ET (patients) | 101 | 66 | 167 |
(SET/DET) | (112/8) | (74/8) | (186/16) |
Transferred blastocysts | 128 | 90 | 218 |
Positive tests | 73 (60.8%) | 44 (53.7%) | 117 (57.9%) |
Biochemical pregnancies | 11 (9.2%) | 7 (8.5%) | 18 (8.9%) |
Clinical pregnancies | 62 (51.7%) | 37 (45.1%) | 99 (49.0%) |
Miscarriages | 15 (12.5%) | 5 (6.1%) | 20 (9.9%) |
Ectopic pregnancies | 1 | 0 | 1 |
Ongoing pregnancies | 6 | 7 | 13 |
Fetal heartbeats | 64 (50.0%) | 40 (44.4%) | 104 (47.7%) |
Deliveries | 41 (34.2%) | 25 (30.5%) | 66 (32.7%) |
Singleton/twin | 40/1 | 22/3 | 62/4 |
Spontaneus deliveries | 17 (41.5%) | 8 (32.0%) | 25 (37.9%) |
Cesarean deliveries | 24 (58.5%) | 17 (68.0%) | 41 (62.1%) |
Babies born (M/F) | 42 (18/24) | 28 (17/11) | 70 (35/35) |
Premature babies | 3 (7.1%) | 8 (28.6%) | 11 (15.7%) |
ET: embryo transfer; SET: single embryo transfer; DET: double embryo transfer; M: male; F: female.
Clinical outcomes of PGD + PGS cycles performed from October 2011 to October 2016.
. | Monogenic disease . | Chromosomal rearrangement . | Total . |
---|---|---|---|
Thawed blastocysts | 92 | 70 | |
Survived blastocysts | 89 (96.7%) | 69 (98.6%) | – |
Total ET (procedures) | 120 | 82 | 202 |
Total ET (patients) | 101 | 66 | 167 |
(SET/DET) | (112/8) | (74/8) | (186/16) |
Transferred blastocysts | 128 | 90 | 218 |
Positive tests | 73 (60.8%) | 44 (53.7%) | 117 (57.9%) |
Biochemical pregnancies | 11 (9.2%) | 7 (8.5%) | 18 (8.9%) |
Clinical pregnancies | 62 (51.7%) | 37 (45.1%) | 99 (49.0%) |
Miscarriages | 15 (12.5%) | 5 (6.1%) | 20 (9.9%) |
Ectopic pregnancies | 1 | 0 | 1 |
Ongoing pregnancies | 6 | 7 | 13 |
Fetal heartbeats | 64 (50.0%) | 40 (44.4%) | 104 (47.7%) |
Deliveries | 41 (34.2%) | 25 (30.5%) | 66 (32.7%) |
Singleton/twin | 40/1 | 22/3 | 62/4 |
Spontaneus deliveries | 17 (41.5%) | 8 (32.0%) | 25 (37.9%) |
Cesarean deliveries | 24 (58.5%) | 17 (68.0%) | 41 (62.1%) |
Babies born (M/F) | 42 (18/24) | 28 (17/11) | 70 (35/35) |
Premature babies | 3 (7.1%) | 8 (28.6%) | 11 (15.7%) |
. | Monogenic disease . | Chromosomal rearrangement . | Total . |
---|---|---|---|
Thawed blastocysts | 92 | 70 | |
Survived blastocysts | 89 (96.7%) | 69 (98.6%) | – |
Total ET (procedures) | 120 | 82 | 202 |
Total ET (patients) | 101 | 66 | 167 |
(SET/DET) | (112/8) | (74/8) | (186/16) |
Transferred blastocysts | 128 | 90 | 218 |
Positive tests | 73 (60.8%) | 44 (53.7%) | 117 (57.9%) |
Biochemical pregnancies | 11 (9.2%) | 7 (8.5%) | 18 (8.9%) |
Clinical pregnancies | 62 (51.7%) | 37 (45.1%) | 99 (49.0%) |
Miscarriages | 15 (12.5%) | 5 (6.1%) | 20 (9.9%) |
Ectopic pregnancies | 1 | 0 | 1 |
Ongoing pregnancies | 6 | 7 | 13 |
Fetal heartbeats | 64 (50.0%) | 40 (44.4%) | 104 (47.7%) |
Deliveries | 41 (34.2%) | 25 (30.5%) | 66 (32.7%) |
Singleton/twin | 40/1 | 22/3 | 62/4 |
Spontaneus deliveries | 17 (41.5%) | 8 (32.0%) | 25 (37.9%) |
Cesarean deliveries | 24 (58.5%) | 17 (68.0%) | 41 (62.1%) |
Babies born (M/F) | 42 (18/24) | 28 (17/11) | 70 (35/35) |
Premature babies | 3 (7.1%) | 8 (28.6%) | 11 (15.7%) |
ET: embryo transfer; SET: single embryo transfer; DET: double embryo transfer; M: male; F: female.
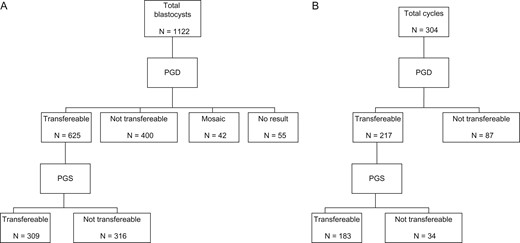
(A) Schematic representation of blastocyst distribution according to the genetic outcome after PGD and PGS analysis. (B) Schematic representation of the distribution of the cycles with and without transferable blastocysts available for transfer after PGD and PGS; PGD: preimplantation genetic diagnosis; PGS: preimplantation genetic screening.
Discussion
Our study demonstrates that the combination of PGD and PGS improves the capability of detecting embryos with high potential to implant simultaneously avoiding the transfer of embryos that, although free of monogenic disease or chromosomal rearrangements, carry chromosomal aneuploidies. Monogenic diseases or chromosomal abnormalities and aneuploidies can be detected with a single TE biopsy without negative impact on blastocyst implantation and developmental potential producing good clinical outcomes, comparable with those recently reported in the literature (Traversa et al., 2010; Tan et al., 2013; De Rycke et al., 2015). The ESHRE PGD Consortium reports that only 28% of the transferred embryos lead to pregnancy (De Rycke et al., 2015). Our study demonstrates that PGD/PGS based IVF is able to increase the embryo implantation rate up to 47.7%. In the present data set we obtained overall 49% clinical pregnancy rate per transfer. This outcome is higher than that reported in our previous study in which only PGD cycles for single gene disorders were performed (Fiorentino et al., 2006). In that study, 427 embryos were transferred in 211 cycles, resulting in 71 (33.6%) clinical pregnancies per embryo transfer and in the birth of 35 healthy babies. Thus our study reporting further improvement in clinical outcomes demonstrates not only that PGS/PGD does not reduce the IVF success rate, but indeed it enhances the efficacy of PGD treatment by increasing the chances of transferring a healthy and euploid embryo. Consistent with our hypothesis, recent additional studies using next-generation sequencing (NGS)-based PGD/PGS also reported a fetal heart pregnancy rate per embryo transfer of 46% for reciprocal carriers and 40% for Robertsonian carriers (Traversa et al., 2010) and ongoing pregnancy rate per transfer of 38% (Tan et al., 2013). It is also important to underline that the patients enrolled in our study had to perform PGD for genetical reasons. We found that out of 304 PGD/PGS cycles performed, 28.6% (N = 87) resulted in no-transferable blastocysts after PGD alone and this percentage significantly increased to 39.8% (N = 121) when also PGS was carried out (Mc Nemar test P < 0.001). Without performing PGS, these cycles could have led to the transfer of aneuploid blastocysts therefore producing negative outcomes.
In 2011, the first clinical pregnancy after concurrent genetic analysis for gangliosidosis and aneuploidy, by single nucleotide polymorphism microarray on a single TE biopsy in 10 blastocysts, was reported (Brezina et al., 2011). Later, Yan et al. (2015) obtained the live births of two babies free of their parent's monogenic diseases, the hereditary multiple exostoses and an X-linked chromosome recessive hereditary disorders. In both case reports, the simultaneous avoidance of monogenic diseases and chromosome abnormalities was obtained by means of NGS and linkage analysis performed on 23 biopsied blastocysts. Konstantinidis et al. (2015) reported the first clinical analysis performed with karyomapping on 55 IVF–PGD cycles. The authors found a high concordance compared to conventional PCR approach. However, in 44 out of 281 blastocysts it was not possible to obtain a conclusive diagnosis after the first biopsy. This rate (15.7%) seems to be still quite high, requiring additional and potentially dangerous invasive procedures on the embryos in order to determine their genetic status. In our data set, we obtained a conclusive diagnosis for double genetic factors in 1067 out of 1122 biopsied blastocysts (95.1%), implying that only 4.9% of them need a second round of warming, biopsy and vitrification. Finally, karyomapping remains a time consuming, costly and extremely complex strategy (Zimmerman et al., 2016). In 2015, Daina et al. performed the double factor PGD/PGS analysis on a single blastomere biopsy from 62 Day-3 embryos obtained in seven cycles in two families at risk for monogenic-inherited diseases. The authors reported that 68.2% of tested embryos were not affected by the monogenic diseases although only 43.3% of them were found to be euploid. In 2016, Zimmerman et al. analyzed 304 blastocysts with concurrent single gene disorders and comprehensive chromosomal aneuploidy screening without whole genome amplification: 116 of them resulted transferable both for single gene defects and aneuploidy, while 47 (15.5%) were found healthy for the monogenic diseases but aneuploid. It is interesting to note that also when the mean female age was young (33 years old in both studies), the percentage of aneuploidy was still high (50% and almost 30% in Daina et al. and Zimmerman et al., respectively), underlying the importance performing comprehensive chromosome screening in PGD cycles. The first four live births obtained in patients with structural chromosomal rearrangements with comprehensive chromosome screening applied to polar bodies, blastomeres or TE cells were reported in 2011 (Alfarawati et al., 2011). Out of 132 analyzed samples, only 22.3% were both normal/balanced and chromosomally normal. Later, Ghevaria et al. (2016) analyzed 298 embryos from 23 couples carriers of Robertsonian (N = 7) and reciprocal translocations (N = 16). Of the analyzed embryos, only 14% (N = 42) resulted both normal and balanced for the translocation, while 63% (N = 170) were diagnosed with additional aneuploidies. Finally Christodoulou et al. (2016) reported nine live births obtained after TE biopsy of 224 blastocysts from 24 couples carrying a balanced chromosomal rearrangement. Authors found that only 35.7% (N = 74) of successfully amplified blastocysts showed a normal array-CGH profile.
Our results are in agreement with previous findings: while 55.7% of the biopsied blastocysts (for which a genetic result was obtained) were healthy after PGD analysis, only 27.5% of them were also chromosomally normal. Without performing aneuploidy screening, 316 blastocysts healthy for the genetic disease could have been transferred leading to implantation failures, miscarriages, or even, to unhealthy live births. To our knowledge, this is the largest reported series of double genetic tests regarding different monogenic diseases or translocations and aneuploidy screening performed with a single biopsy on 1122 blastocysts leading to 70 healthy live births.
Bay et al. (2016) reported that PGD pregnancies are at increased risk of cesarean section, preterm birth and shorter gestation, compared with spontaneous conceptions. These risks were comparable with those achieved after IVF/ICSI. Particularly they found that 53.2% of PGD pregnancies ended with a cesarean delivery and that 17.4 and 19.5% PGD newborns were preterm (<37 weeks of gestation) and with low birth weight (<2500 g), respectively. In our data we found comparable percentage of cesarean sections (62.1%) and preterm/low birth weight babies (15.7%). In addition, Bay et al. in a sub-analysis of their results, underlined that adverse outcomes were present in children born from parents with monogenic disorders and concluded that obstetric and neonatal risks are mainly related to the underlying parental conditions rather than to the PGD procedure. Comparing monogenic diseases with chromosomal rearrangements, we found a lower percentage of premature babies in the former (7.1 vs 28.6%, respectively). Although the number of newborns reported in our study is still too small in order to draw significant conclusions and more data are required to clarify whether there could be an influence of the parental disorder on the final obstetric and perinatal outcomes.
In conclusion, we strongly believe that when the biopsy needs to be performed in order to prevent the transmission of an inheritable disease, it should be mandatory to analyze also the genetic status of the blastocyst, avoiding useless and/or harmful embryo transfers in this particular category of patients. However, a higher than expected rate of cycle cancellation could be found due to the double cytogenetic analysis performed. For this reason, particular care should be taken in drafting and explaining informed consents, especially about the risks related to this approach, in order to avoid patients’ drop out.
Supplementary data
Supplementary data are available at Human Reproduction online.
Authors’ roles
M.G.M. is the director of the embryologic laboratory, coordinated all the embryological laboratory procedures and was involved in the study conception and design, data analysis and drafting article. F.F. was involved in the genetic analysis, critical revision of the article and final approval. A.R. and E.C. (Elisabetta Cursio) played a central role in the embryologic laboratory procedures, data analysis and final approval. A.B. played a central role in the genetic laboratory procedures, critical revision of the article and final approval. E.C. (Ettore Cotroneo) and M.S. played a central role in the genetic laboratory procedures, data analysis and final approval. M.T.V. was involved in stimulation protocols, in surgical procedures and final approval. F.S. was involved in the genetic analysis, in the critical revision of the article and final approval. E.G. was involved in the study conception and design, in the critical revision of the article, in stimulation protocols and final approval.
Funding
This work has not received any external financial support from any commercial company.
Conflict of interest
None declared.
References
Author notes
Presented at the ESHRE 32th annual meeting, Helsinki, Finland, July 3–6, 2016. Pre-selected for the Clinical Science Award for Oral Presentation and for the Fertility Society of Australia Exchange Award.
- polymerase chain reaction
- pregnancy
- informed consent
- chromosome abnormality
- biopsy
- chromosome rearrangements
- genetic disorder
- abortion, spontaneous
- aneuploidy
- embryo stage 3
- blastomere
- chromosomes
- cryopreservation
- embryo
- embryo transfer
- genome
- infant
- oocytes
- ovum implantation
- ploidies
- translocation (genetics)
- diagnosis
- genetics
- mosaicism
- treatment outcome
- genetic analysis
- genetic screening
- live birth
- amplification
- transfer technique