-
PDF
- Split View
-
Views
-
Cite
Cite
H.A. Guidobaldi, M. Cubilla, A. Moreno, M.V. Molino, L. Bahamondes, L.C. Giojalas, Sperm chemorepulsion, a supplementary mechanism to regulate fertilization, Human Reproduction, Volume 32, Issue 8, August 2017, Pages 1560–1573, https://doi.org/10.1093/humrep/dex232
- Share Icon Share
Abstract
Are human spermatozoa able of chemorepulsive behaviour?
Capacitated human spermatozoa are able to be chemorepelled by synthetic Progesterone Receptor Ligands (sPRL, known as contraceptives) and zinc (a cation released by the oocyte upon fertilization).
Moving cells can be oriented towards or against a molecular gradient, processes called chemoattraction and chemorepulsion, respectively, which have been described in unicellular organisms such as amoebas and bacteria, to organismic cells such macrophages and developmental cells. In the case of spermatozoa, chemoattraction may help the finding of an oocyte and has been widely studied in various invertebrate and mammalian species; however, chemorepulsion has not yet been verified in spermatozoa.
This is an in vitro study involving human, rabbit and mouse spermatozoa which were used to perform 3–30 experiments per treatment.
Human sperm samples were obtained by masturbation from healthy donors who gave written consent. Only those samples exhibiting normal semen parameters according to current WHO criteria were included in the study. Rabbit spermatozoa were obtained by artificial vagina whereas mice spermatozoa were obtained from epididymis. The sperm selection assay (SSA), originally designed to evaluate sperm chemoattraction towards progesterone (P), and a video-microscopy and computer motion analysis system were used to test sperm chemorepulsion. Additional kinetic parameters were also determined by video-microscopy and computer motion analysis. In some experiments, the level of induced acrosome-reacted spermatozoa was determined. Rabbit mating manipulation was achieved to perform the sperm–oocyte co-incubation assay.
Sperm accumulation in the well containing 100 pg/ml of sPRL was lower than the culture medium negative control (P < 0.05). The percentage of sperm persistence against the well containing 100 pg/ml ulipristal acetate (UPA) (P = 0.001), and the percentage of sperm showing a repulsive pattern of movement (a linear trajectory followed by a transitional one after turning against the UPA), were higher than the culture medium negative control (P = 0.049). Sperm accumulation was diminished when spermatozoa where exposed to a homogeneous distribution of 100 pg/ml sPRL combined with a chemotactic gradient of progesterone (P), with respect to the culture medium negative control (P < 0.05). These results were reverted when non-capacitated spermatozoa were used to perform the same experimental settings. The accumulation of spermatozoa against 100 pg/ml sPRL was lower than the culture medium negative control also in rabbits and mice (P < 0.05). The relative number of rabbit spermatozoa arriving to the vicinity of the oocyte was diminished under the presence of 100 pg/ml UPA (P = 0.004). Sperm accumulation in the well containing zinc was decreased compared to the culture medium negative control (P < 0.05). A homogeneous distribution of zinc combined with a gradient of 10 pM P, was lower than the culture medium negative control (P = 0.016). The results were quite reproducible with two different methodologies (accumulation assay and video-microscopy combined with computer motion analysis), in three mammalian species.
The experiments were performed in vitro. Even though a quite complete characterization of sperm chemorepulsion was provided, the molecular mechanism that governs sperm repulsion is currently under investigation.
Since the chemorepelled spermatozoa are those physiologically ready to fertilize the oocyte, these findings may have both biological and clinical implications, preventing either polyspermy under natural conditions or fertilization under pharmacological treatment with sPRL.
The study was financed by the Universidad Nacional de Cordoba (Argentina). The authors declare that they do not have competing financial interests.
N/A.
Introduction
Moving cells can travel at random or orient themselves towards a specific place. The latter capability is mediated by means of ‘taxis’ in response to external stimulus such as chemicals, temperature, fluid flow and light, among others. The orientation mechanism is named according to the stimulant. Most taxis mechanisms are reported as positive or negative, depending on the effect of the stimulus. The cell orientation mediated by a molecular gradient is generally called chemotaxis, which becomes positive when the cells move towards the source of an attractant, namely chemoattraction, or negative when they move against a repellent source, namely chemorepulsion (Eisenbach, 2004; Huttenlocher and Poznansky, 2008; Reig et al., 2014).
Chemoattraction and chemorepulsion have been described in several cell types, from unicellular organisms such as amoebas and bacteria, to organismic cells such macrophages and developmental cells (Masuda and Shiga, 2005; Huttenlocher and Poznansky, 2008). These taxes explain how cells reach a food source or a wound, or avoid a toxic or improper developmental area. A common feature of directional movement is that the stimulus has to be gradually distributed from its source to indicate the pathway, and that the cells have to be in a singular physiological state (Huttenlocher and Poznansky, 2008). In general, an oriented moving cell needs a receptor (usually located in the cell surface) that senses the stimulus gradient, plus an intracellular signal transduction mechanism that regulates the molecular motor that propels the cell. In some cases, the same ligand and/or receptor modulates these two opposing cell orientation movements, in which their signalling cascades may also be similar (Huttenlocher and Poznansky, 2008). Therefore, chemoattraction and repulsion seem to be complementary processes that operate alternatively to drive the cell according to environmental sensing.
In the case of spermatozoa, chemoattraction may help the finding of an oocyte. This phenomenon has been widely studied in various invertebrate and mammalian species (see references in Eisenbach and Giojalas, 2006 and Giojalas et al., 2015) whose main features are summarized below. In mammals, sperm chemoattraction was reported for humans, mice, rabbits and bovines. Not all spermatozoa are capable of a chemotactic response. Only the few that have acquired a specific physiological state (‘capacitated’) enabling them to fertilize the oocyte, are capable of chemotactic orientation. Spermatozoa can sense several chemical types of gradients of attractants such as hormones, peptides and cyclic nucleotides. Among these, progesterone (P) is of interest because, by the time of ovulation it is secreted by the cumulus cells that surround the oocyte (Vanderhyden and Tonary, 1995; Yamashita et al., 2003), generating a gradual hormone distribution along the cumulus oophorus and beyond (Teves et al., 2006; Guidobaldi et al., 2008). It has been described that human, rabbit and mouse spermatozoa are chemotactically attracted in vitro by a gradient of very low doses of P (Teves et al., 2006, 2009, 2010; Guidobaldi et al., 2008, 2017; Blengini et al., 2011; Gatica et al., 2013; Ernesto et al., 2015). However, although several studies have been dedicated to sperm chemotactic behaviour (Eisenbach and Giojalas, 2006; Giojalas et al., 2015), as far as we know, sperm chemorepulsion was once reported (Tso et al., 1979) but without being fully verified. If sperm chemorepulsive behaviour exists, it could participate in regulating successful fertilization beyond the gamete fusion event, for instance avoiding polyspermy as suggested previously by Eisenbach (2004). In preliminary experiments to test the effect of emergency contraceptives on sperm chemoattraction mediated by P, a response similar to chemorepulsion was observed.
In order to further elucidate this intriguing result, several synthetic Progesterone Receptor Ligands (sPRL) used as contraceptives: mifepristone (MIFE), levonorgestrel (LNG) and ulipristal acetate (UPA) (Chabbert-Buffet et al., 2005) were tested for sperm chemorepulsion. Taken orally, these drugs enter the blood circulation and reach the female reproductive tract and fluids (e.g. cervix, uterus, oviduct) (Saboya Brito et al., 2005). In the presence of P, sPRL behave as P receptor antagonists (Smith and O'Malley, 2004). Several reports suggest that spermatozoa may have one or more cell surface P receptors (Guidobaldi et al., 2008; Thomas, 2008; Miller et al., 2016), thus raising the possibility that spermatozoa may interact with sPRL when they reach the female reproductive tract. In the present study, we showed by means of a simple assay that capacitated spermatozoa can be repelled by sPRL even in the presence of a P gradient, preventing them reaching the oocyte surface.
Material and Methods
Ethics statement
The experiments were performed with human, rabbit and mouse spermatozoa. Human semen samples were treated in accordance with the Declaration of Helsinki. The study was conducted with the approval of the Ethics Committee of the Hospital Nacional de Clínicas (Universidad Nacional de Córdoba, Argentina; #06/10/E). Animals were treated in accordance with the Guide for care and use for laboratory animals (NIH), and the experimental protocols were approved by the Ethics Committee for animal use and care (Universidad Nacional de Cordoba; #7/2015).
Sperm preparation
Human semen samples were obtained by masturbation from donors who gave written consent to participate in the study. Only samples exhibiting normal semen parameters according to the World Health Organization criteria (WHO, 2010) were included in the study. Rabbit semen samples were obtained by artificial vagina (Fabro et al., 2002). Spermatozoa were separated from the seminal plasma by migration–sedimentation in Biggers, Whitter and Whittingham (BWW) medium supplemented with 1% bovine serum albumin (BSA), as previously described (Fabro et al., 2002). The sperm suspension at 8 × 10 cells/ml was further incubated at 37°C in an atmosphere containing 5% CO2 in air, during 4 or 16 h, for humans and rabbits, respectively, or as otherwise specified. Mouse spermatozoa were obtained from cauda epididymis and the sperm suspension at 3 × 106 cells/ml was capacitated in BWW medium supplemented with 1% BSA for 1.5 h.
Sperm selection assay
This method was performed according to the procedure previously described (Gatica et al., 2013), adjusting sperm concentration to 6 × 106 cells/ml for humans and rabbits, and to 3 × 106 cells/ml for mice. To perform the assay (see the device's sketch in Table I), one well (W1) was first filled with the sperm suspension and the other (W2) with the test substance, which gradually diffuses from W2 to W1 along the connecting tube. After 20 min of incubation, the sperm suspension was recovered from W2, and the cell concentration was determined in a Neubauer chamber. The percentage of sperm accumulation in W2 after the SSA was calculated as the difference in the percentage of spermatozoa recovered from W2 with or without the test substance, which was determined by dividing the number of spermatozoa recovered from W2 after the SSA by the number of spermatozoa placed in W1 before the SSA, multiplying the result by 100. In all the experiments, a culture medium control was performed, which was used as a reference to determine sperm accumulation. In addition, in each experiment a chemoattraction control was performed by generating an ascending gradient of P from a concentration of 10 pM for humans, and 100 pM for rabbits and mice, running the respective culture medium control in parallel.
Experimental design to differentiate sperm chemorepulsion from chemoattraction and other kinetic processes. Left column shows a sketch of the SSA device indicating the place and distribution of the test substance, whereas spermatozoa are placed in W1 and the test substance gradient (ascending or descending) is formed along the connection between wells and from the well containing the test substance. When the test substance is homogenously distributed in the SSA device, no molecular gradient is formed. Sperm accumulation in W2 containing the test substance is compared to sperm accumulation in W2 containing culture medium as negative control (not shown). The combination of the results allows to discriminate chemorepulsion from chemoattraction, chemokinesis and hyperactivation. (Adapted from Giojalas et al., 2015.)
Test substance place and distribution in the SSA device . | Expected sperm accumulation in W2 compared to culture medium negative control . | |||
---|---|---|---|---|
Chemorepulsion . | Chemoattraction . | Chemokinesis . | Trapping . | |
i) Ascending gradient | Reduced | Increased | Increased | Increased |
(spermatozoa are repelled from W2 and return to W1) | (spermatozoa are oriented to W2) | (velocity is increased, hence spermatozoa reach W2 faster) | (sperm reaching W2 are retained there due to non-progressive movement) | |
ii) Homogeneous distribution | None | None | Increased | Reduced |
(sperm swims at random due to the absence of the test substance gradient) | (sperm swims at random due to the absence of the test substance gradient) | (velocity is increased, hence spermatozoa reach W2 faster) | (due to a non-progressive movement, spermatozoa are trapped by the test substance in W1 and cannot swim to W2) | |
iii) Descending gradient | Increased | Reduced | Increased | Reduced |
(spermatozoa are repelled from W1 and accumulates in W2) | (spermatozoa swim out from W1, sense the attractant gradient and returns to W1) | (velocity is increased, hence spermatozoa reach W2 faster) | (due to a non-progressive movement, spermatozoa are trapped by the test substance in W1 and cannot swim to W2) |
Test substance place and distribution in the SSA device . | Expected sperm accumulation in W2 compared to culture medium negative control . | |||
---|---|---|---|---|
Chemorepulsion . | Chemoattraction . | Chemokinesis . | Trapping . | |
i) Ascending gradient | Reduced | Increased | Increased | Increased |
(spermatozoa are repelled from W2 and return to W1) | (spermatozoa are oriented to W2) | (velocity is increased, hence spermatozoa reach W2 faster) | (sperm reaching W2 are retained there due to non-progressive movement) | |
ii) Homogeneous distribution | None | None | Increased | Reduced |
(sperm swims at random due to the absence of the test substance gradient) | (sperm swims at random due to the absence of the test substance gradient) | (velocity is increased, hence spermatozoa reach W2 faster) | (due to a non-progressive movement, spermatozoa are trapped by the test substance in W1 and cannot swim to W2) | |
iii) Descending gradient | Increased | Reduced | Increased | Reduced |
(spermatozoa are repelled from W1 and accumulates in W2) | (spermatozoa swim out from W1, sense the attractant gradient and returns to W1) | (velocity is increased, hence spermatozoa reach W2 faster) | (due to a non-progressive movement, spermatozoa are trapped by the test substance in W1 and cannot swim to W2) |
Experimental design to differentiate sperm chemorepulsion from chemoattraction and other kinetic processes. Left column shows a sketch of the SSA device indicating the place and distribution of the test substance, whereas spermatozoa are placed in W1 and the test substance gradient (ascending or descending) is formed along the connection between wells and from the well containing the test substance. When the test substance is homogenously distributed in the SSA device, no molecular gradient is formed. Sperm accumulation in W2 containing the test substance is compared to sperm accumulation in W2 containing culture medium as negative control (not shown). The combination of the results allows to discriminate chemorepulsion from chemoattraction, chemokinesis and hyperactivation. (Adapted from Giojalas et al., 2015.)
Test substance place and distribution in the SSA device . | Expected sperm accumulation in W2 compared to culture medium negative control . | |||
---|---|---|---|---|
Chemorepulsion . | Chemoattraction . | Chemokinesis . | Trapping . | |
i) Ascending gradient | Reduced | Increased | Increased | Increased |
(spermatozoa are repelled from W2 and return to W1) | (spermatozoa are oriented to W2) | (velocity is increased, hence spermatozoa reach W2 faster) | (sperm reaching W2 are retained there due to non-progressive movement) | |
ii) Homogeneous distribution | None | None | Increased | Reduced |
(sperm swims at random due to the absence of the test substance gradient) | (sperm swims at random due to the absence of the test substance gradient) | (velocity is increased, hence spermatozoa reach W2 faster) | (due to a non-progressive movement, spermatozoa are trapped by the test substance in W1 and cannot swim to W2) | |
iii) Descending gradient | Increased | Reduced | Increased | Reduced |
(spermatozoa are repelled from W1 and accumulates in W2) | (spermatozoa swim out from W1, sense the attractant gradient and returns to W1) | (velocity is increased, hence spermatozoa reach W2 faster) | (due to a non-progressive movement, spermatozoa are trapped by the test substance in W1 and cannot swim to W2) |
Test substance place and distribution in the SSA device . | Expected sperm accumulation in W2 compared to culture medium negative control . | |||
---|---|---|---|---|
Chemorepulsion . | Chemoattraction . | Chemokinesis . | Trapping . | |
i) Ascending gradient | Reduced | Increased | Increased | Increased |
(spermatozoa are repelled from W2 and return to W1) | (spermatozoa are oriented to W2) | (velocity is increased, hence spermatozoa reach W2 faster) | (sperm reaching W2 are retained there due to non-progressive movement) | |
ii) Homogeneous distribution | None | None | Increased | Reduced |
(sperm swims at random due to the absence of the test substance gradient) | (sperm swims at random due to the absence of the test substance gradient) | (velocity is increased, hence spermatozoa reach W2 faster) | (due to a non-progressive movement, spermatozoa are trapped by the test substance in W1 and cannot swim to W2) | |
iii) Descending gradient | Increased | Reduced | Increased | Reduced |
(spermatozoa are repelled from W1 and accumulates in W2) | (spermatozoa swim out from W1, sense the attractant gradient and returns to W1) | (velocity is increased, hence spermatozoa reach W2 faster) | (due to a non-progressive movement, spermatozoa are trapped by the test substance in W1 and cannot swim to W2) |
Experimental design to test sperm chemorepulsion
The sperm selection assay (SSA) that we used was originally designed to recruit spermatozoa by chemoattraction towards P (Gatica et al., 2013), and can also be used to evaluate chemorepulsion. The device consists of two wells with a connecting tube between them. Spermatozoa are loaded in one well (W1) and then they swim along the connecting tube when they sense how the test substance is distributed. Depending on where the substance is placed, three different experimental designs can be set up: (i) the test substance in W2, generating an ascending concentration gradient from W1 to W2; (ii) the test substance homogeneously distributed all over the SSA device, hence forming no gradient; and (iii) the test substance in W1 together with spermatozoa, generating a descending concentration gradient from W1 to W2. At the end of the assay, the percentage of spermatozoa accumulated in W2 (compared to the SSA run with culture medium) is determined. Chemorepulsive behaviour would therefore be characterized by reduced sperm accumulation in W2 when cells are repelled from W2 in an ascending gradient, increased sperm accumulation in W2 when cells are repelled from W1 in a descending gradient, and no accumulation (cells move at random when the repellent is homogeneously distributed). The combination of experiments that allows to discriminate sperm accumulation due to chemorepulsion or chemoattraction as well as other kinetic processes is shown in Table I. In each set of experiments, an ascending gradient of 10 pM of P was run in parallel, as a chemoattraction control.
Sperm repulsion determined by video-microscopy and computer motion analysis
The sperm chemorepulsion assay was performed on a modified chemoattraction chamber according to Guidobaldi et al. (2008). Briefly, 6 × 106 cells/ml of human spermatozoa were placed in one compartment (W1) of the chamber while progesterone or UPA were loaded in the other compartment (W2). Thus, a concentration gradient is formed in the capillary space between wells, where sperm movement was recorded. Culture medium was placed in W2 in a parallel chamber, as negative control. Five minutes after sealing the chamber, sperm tracks were recorded in the middle of the capillary space, at 30 Hz for 15 min under a phase contrast microscope (Nikon Instruments Inc., NY, USA). Then, the trajectories of 1500–3000 spermatozoa per treatment were determined automatically with the Fiji software (Schindelin et al., 2012) and the Particle Tracker plugin (Sbalzarini and Koumoutsakos, 2005; Chenouard et al., 2014). Sperm orientation was determined as the sperm persistence in one defined direction. This parameter was calculated with a home-made Fiji macro (paNoel 0.7.4; Universidad Nacional de Córdoba) using the coordinates determined with the Particle Tracker plug-in. This home-made macro evaluates the orientation of 100 consecutive points along each sperm trajectory. This procedure together with the ability to automatically track a high number of spermatozoa per treatment improves the accuracy of sperm orientation determination without the need to perform a lot of experiment replications. The percentage of sperm persistence was determined as the proportion of points on each direction divided by the total number of points multiplied by 100 for each spermatozoon. This calculation was defined for three alternatives directions: toward W2, against W2 and perpendicular to the W1–W2 axis. For each treatment, the mean percentage of sperm persistence was calculated on the bases of each single cell percentage of persistence. The net percentage of sperm persistence was calculated as the difference between each treatment and its corresponding negative control. This home-made macro also determines the fractal dimension (Mortimer et al., 1996), which allows to analyse the pattern of movement of each spermatozoon during swimming along a defined direction.
Sperm kinematic parameters
Straight line velocity (VSL), curvilinear velocity (VCL) (Mortimer et al., 1988) and pattern of movement (Fabro et al., 2002) were determined by video-microscopy and computer motion analysis. Capacitated human spermatozoa were incubated under different treatments for 20 min at 37°C. Then, a droplet of 10 μl of sperm suspension was put on a slide pre-warmed at 37°C, covered with an 18 × 18 mm2 coverslip and sealed with mineral oil. Immediately after, the slide was placed over a thermal plate (37°C) in a phase contrast microscope (Nikon Instruments Inc., NY, USA). Then, sperm movement was digitally recorded at 30 Hz for 20 s using a ×10 objective. Then, at least 200 sperm trajectories per treatment were determined automatically with the Fiji software (Schindelin et al., 2012) and the Particle Tracker plugin (Sbalzarini and Koumoutsakos, 2005; Chenouard et al., 2014). Sperm kinematic parameters were calculated with a home-made Fiji macro (paNoel 0.7.4; Universidad Nacional de Córdoba) using the coordinates determined with the Particle Tracker plug-in.
Induced acrosome reaction
Acrosome reaction is considered as the end point of the capacitation process (Jaiswal et al., 1998). Thus, the pharmacologically induced acrosome reaction was determined as an indication of the sperm capacitation level as previously described (Fabro et al., 2002). The acrosome status was verified by means of the Coomassie Blue labelling (Larson and Miller, 1999). The sperm suspension was divided into two aliquots, one was stimulated with 8 μM A23187 (Sigma-Aldrich, St. Louis, USA) for 30 min at 37°C and the other was kept as control. After fixing the cells with 1% formaldehyde for 20 min, spermatozoa were washed three times with 100 mM ammonium acetate solution for 7 min each at 300g. The cells were stained for 10 min with 0.22% Coomassie Blue in a solution of 10% acetic acid: 50% methanol in water. After washing with distilled water, spermatozoa were put on a slide, left to dry at room temperature, and mounted with 90% glycerol in PBS. The state of the acrosome vesicle was observed in at least 200 cells per sample at ×1000 under a light microscope (Olympus Corp., Tokyo, Japan). The percentage of acrosome-intact (dark blue acrosome) and acrosome-reacted (light blue acrosome) spermatozoa was determined.
Sperm–oocyte co-incubation assay
This assay was performed with oocyte cumulus complexes (OCC) obtained by surgery from rabbit females induced for ovulation by mating. The OCC were incubated in M199 culture medium for ~14–16 h at 37°C until cumulus expansion. Then, the OCC were washed twice with 250 μl of M199 culture medium and then were placed in a 150 μl drop of fresh M199 culture medium, with or without 100 pg/ml of MIFE or UPA. Rabbit spermatozoa were collected and capacitated as mentioned above for 15 h. Then they were loaded with 2 μM Fluo 4-AM (Uñates et al., 2014) and incubated for 15 min with sPRL, at the same concentration used with the OCC or without it. Then, 10 000 motile spermatozoa were put together with the OCC and, after 15 min of co-incubation, the cells were fixed with 4% formaldehyde for 20 min. They were directly visualized, maintaining the three-dimensional structure of the OCC, under a fluorescence microscope (Nikon Instruments Inc., NY, USA). The total number of spermatozoa around the oocyte was determined through a vertical scanning of the OCC, verifying the presence of sperm in all focal planes. Data was expressed as the relative sperm number determined by the sperm count in each experiment divided by the mean number of sperm in the control.
Statistical analysis
Each experiment (including the corresponding control) was performed with the same semen sample, whereas at least three experiments were performed for each treatment (the number of experiments per treatment is included in each figure). Significant differences between mean values were determined by the ‘t’ test or one-way ANOVA by means of the Infostat software (Di Rienzo et al., 2011), where differences between treatments were determined with the DGC (David Garcia Callejas) post-hoc test (Di Rienzo et al., 2002), considering statistically significant differences at P ≤ 0.05.
Results
Spermatozoa show chemorepulsive behaviour
To test sperm chemorepulsion, several sPRL concentrations were placed in W2 (ascending gradient). Spermatozoa showed a bell-shaped chemorepulsive response, where the maximum chemorepellent effect was mainly observed at 100 pg/ml, equivalent to 210 pM for UPA, 233 pM for MIFE and 320 pM for LNG (Fig. 1A). Sperm chemorepulsion was confirmed by exposing spermatozoa to a descending gradient of sPRL, at which a significant increase in sperm accumulation in W2 was observed (Fig. 1B), meaning that they were repelled from W1 containing the sPRL. No sperm accumulation was observed in W2 when sPRL were homogeneously distributed along the SSA device (Fig. 1C). This experiment was performed as a negative control of chemorepulsion because its occurrence depends on the presence of a gradient. The sperm velocity and pattern of movement were not affected by sPRL, as confirmed by video-microscopy and computer motion analysis (Supplementary Table S1), showing that these parameters did not interfere with repulsion determination. A chemotactic (ascending) gradient of P and culture medium controls were performed in parallel (Fig. 1D). A similar assay was performed with fulvestrant (a steroid that antagonizes the estrogen receptor; Wakeling et al., 1991) and no repellent effect was observed (Fig. 2), suggesting that the repellent effect mediated by sPRL may be specific.
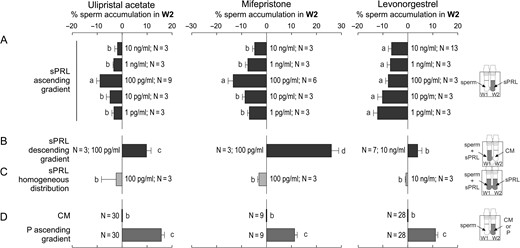
Spermatozoa elicit chemorepulsive behaviour. Spermatozoa previously incubated under capacitating conditions were used to run the Sperm Selection Assay (SSA) under different experimental settings, and then the percentage of spermatozoa recovered from W2 was determined. (A) Spermatozoa were placed in W1 of the SSA chamber while different concentrations of synthetic Progesterone Receptor Ligands (sPRL) were placed in W2, generating an ascending gradient. The following experiments were performed with the sPRL concentration that showed the highest negative response. (B) Spermatozoa were placed in W1 together with the sPRL generating a descending gradient. (C) Spermatozoa were placed in W1 and the sPRL were homogeneously distributed along the SSA device. (D) Control treatments: spermatozoa were placed in W1 and culture medium (CM) or 10 pM of progesterone (P) were placed in W2. Data are expressed as mean ± SEM and the number of independent experiments (N) is shown for each treatment. Different letters (a, b, c) indicate significant differences between treatments, P < 0.05.
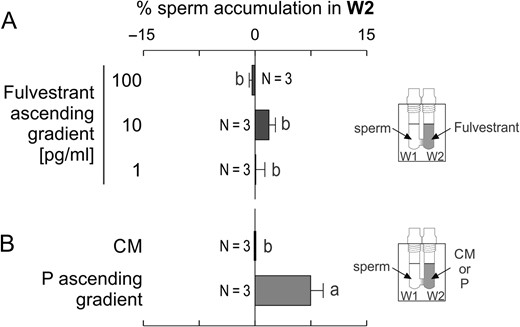
Fulvestrant, a steroid that antagonizes the estrogen receptor, did not repel spermatozoa. Spermatozoa previously incubated under capacitating conditions were placed in W1 under different experimental settings, and then the percentage of spermatozoa recovered from W2 was determined. (A) an ascending gradient generated by different concentrations of Fulvestrant. (B) Control treatments: culture medium (CM) or an ascending gradient of 10 pM of progesterone (P). Data are expressed as mean ± SEM and the number of independent experiments (N) is shown for each treatment. Different letters (a, b) indicate significant differences between treatments, P < 0.05.
Moreover, we further investigated this sperm behaviour in a chemoattraction chamber (Fabro et al., 2002), according to sperm orientation evaluated by video-microscopy combined with a new automatic motion analysis software. The rational and dimensions of this chamber are similar to those of the SSA device. Hence, capacitated spermatozoa were placed in W1 and 10 pM P, 100 pg/ml UPA or culture medium (as negative control) in W2. The percentage of sperm persistence against W2, as compared to the negative control, shows a significant increment under a UPA gradient and a significant decrease under the P gradient (Fig. 3A). In contrast, the percentage of sperm persistence towards W2, as compared to the negative control, shows a significant decrease under a UPA gradient and a significant increase under the P gradient (Fig. 3B). Meanwhile, the percentage of sperm persistence moving perpendicular to the W1–W2 axis under the UPA or P gradient was similar to the negative control (Fig. 3C). We further analysed the sperm pattern of movement while changing direction against W2 containing UPA or culture medium as negative control. Six different patterns were observed as described in Fig. 4. Then, we determined the percentage of spermatozoa showing each pattern in comparison with the culture medium negative control. The percentage of cells showing a linear movement followed by a transitional one after turning against W2 (pattern C) was significantly higher than that of the negative control (Fig. 4), as similarly observed for sperm chemoattraction towards P (Blengini et al., 2011). As a whole, the results obtained by two different methodologies (accumulation assay and video-microscopy combined with computer motion analysis), suggested that spermatozoa show a behaviour consistent with chemorepulsion mediated by sPRL.
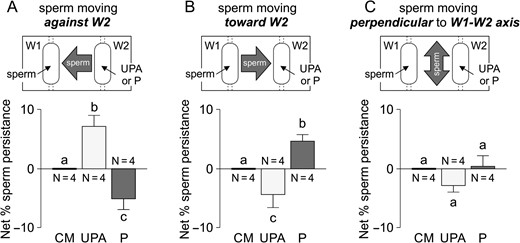
Sperm chemorepulsion verified by video-microscopy and computer motion analysis. Experiments were performed in a chemoattraction chamber (Fabro et al., 2002) where spermatozoa previously incubated under capacitating conditions were loaded in W1 and 100 pg/ml of ulipristal acetate (UPA) or 10 pM progesterone (P) were placed in W2, which formed an ascending gradient between both wells. Net percentage of sperm persistence was determined in three directions: cells moving against W2 (A), cells moving towards W2 (B), and cells moving perpendicular to the W1–W2 axis (C). Data are expressed as mean ± SEM and the number of independent experiments (N) is shown for each treatment. Different letters (a, b, c) indicate significant differences between treatments, P < 0.05.
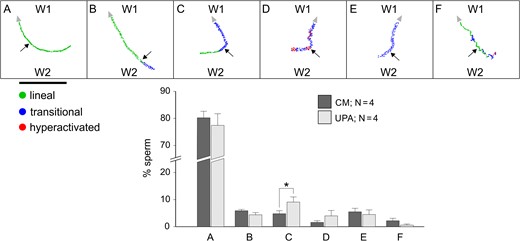
Sperm chemorepulsion verified by means of the sperm pattern of movement while changing direction against W2 containing UPA. Experiments were performed in a chemoattraction chamber (Fabro et al., 2002) where spermatozoa previously incubated under capacitating conditions were loaded in W1 and 100 pg/ml of ulipristal acetate (UPA) (ascending gradient) or culture medium as negative control were placed in W2. The pattern of movement (lineal, transitional or hyperactivated) was determined while turning against the UPA gradient. Six sperm returning patterns were identified (A–F). The percentage of spermatozoa showing each returning pattern (for UPA and culture medium negative control) was represented in the bar chart. Horizontal black bar represents a distance of 200 μm. Arrow head indicates the direction of each sperm track. Arrows point out the moment where the sperm turned against W2. Data are expressed as mean ± SEM and the number of independent experiments (N) is shown for each treatment. * indicates significant differences between treatments, P < 0.05.
sPRL repel spermatozoa from the progesterone attracting source
If the sPRL pill is administered after ovulation, then a homogeneous distribution of sPRL could co-exist with the P gradient generated by the cumulus cells surrounding the oocyte. To test whether this situation may affect sperm attraction, the following experimental design was performed. Capacitated spermatozoa were pre-incubated with sPRL and then loaded in W1 with 10 pM of P in W2 (ascending gradient of P), and sPRL were distributed homogeneously throughout the SSA device. If sPRL inhibit sperm chemoattraction mediated by P, sperm accumulation should not increase in W2. A significant decrease in sperm accumulation was observed in W2 in the presence of sPRL (Fig. 5A), compared to the P gradient and culture medium controls (Fig. 5C). This decreased sperm accumulation was reverted by removing the sPRL by centrifugation and then exposing spermatozoa to the P gradient (Fig. 5B). These results suggest that, although a P gradient is expected to attract spermatozoa, it can be converted into a repulsive gradient in the presence of sPRL.
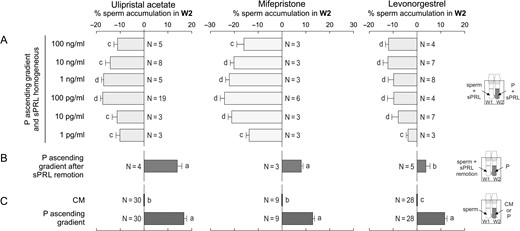
sPRL repel spermatozoa from the progesterone attracting source. Spermatozoa previously incubated under capacitating conditions were used to run the SSA under different experimental settings, and then the percentage of spermatozoa recovered from W2 was determined. (A) Before running the SSA, spermatozoa were previously incubated with different concentrations of each synthetic Progesterone Receptor Ligands (sPRL) for 20 min; then they were placed in W1 of the SSA chamber while the corresponding sPRL concentration was homogeneously distributed in the presence of an ascending gradient of 10 pM of progesterone (P). (B) Spermatozoa were previously incubated for 20 min with the highest active concentrations of each sPRL, which were then removed by centrifugation; then, these washed cells were loaded in W1 under the presence of an ascending gradient of P. (C) Control treatments: spermatozoa were placed in W1 and culture medium (CM) or 10 pM of P were placed in W2. Data are expressed as mean ± SEM and the number of independent experiments (N) is shown for each treatment. Different letters (a, b, c, d) indicate significant differences between treatments, P < 0.05.
Sperm chemorepulsion is apparently conserved in mammals
Since chemoattraction has been observed in several mammalian species, sperm chemorepulsion was also tested in mouse epididymis and rabbit ejaculated spermatozoa. A significant decrease in sperm accumulation in W2 was observed when spermatozoa of these two species were exposed to either an ascending gradient or a homogeneous distribution of sPRL, combined with an ascending gradient of P (Fig. 6). These results suggest that sperm's chemorepulsive behaviour is apparently conserved in mammals.
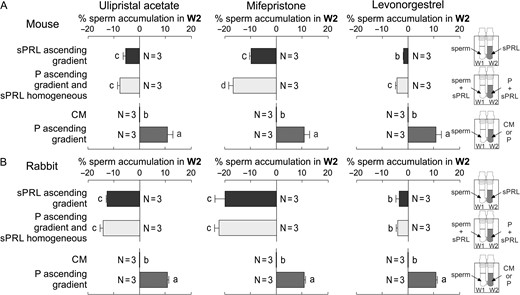
Sperm chemorepulsion is apparently conserved in mammals. Mouse (A) and rabbit (B) spermatozoa previously incubated under capacitating conditions were placed in W1 and treated as follows: an ascending gradient of the synthetic Progesterone Receptor Ligands (sPRL; 100 pg/ml); a homogeneous distribution of sPRL (100 pg/ml) combined with an ascending gradient of 10 pM of progesterone (P); culture medium (CM) or 10 pM P were placed in W2 as controls. Data are expressed as mean ± SEM and the number of independent experiments (N) is shown for each treatment. Different letters (a, b,c) indicate significant differences between treatments for each sPRL and species, P < 0.05.
Spermatozoa already prepared to fertilize the oocyte are chemorepelled by sPRL
Since capacitated spermatozoa are those recruited in W2 by a 10 pM gradient of P (Fig. 7A; Gatica et al., 2013), we next tested whether the sperm's chemorepulsive behaviour was also dependent on sperm capacitation. When the SSA was performed with non-capacitated spermatozoa (immediately after seminal plasma removal), they were not repelled by an ascending gradient of sPRL or by the presence of a homogeneous distribution of sPRL combined with a gradient of P, whereas no chemoattraction was observed under an ascending gradient of P (Fig. 7B). In another set of experiments, we determined the level of induced acrosome-reacted spermatozoa (as an indicator of capacitation), in those recovered from W2 after performing the SSA with spermatozoa previously incubated under capacitating conditions. As expected, an increase in the level of induced acrosome-reacted spermatozoa was observed in W2 in the presence of the P ascending gradient (Fig. 7C). Meanwhile, a significant depletion in induced acrosome-reacted sperm in W2 was observed in the presence of sPRL homogeneously distributed under a P gradient (Fig. 7C). Furthermore, by means of video-microscopy and computer motion analysis system, non-capacitated spermatozoa did not show repulsive behaviour under the UPA gradient nor attracted behaviour towards P (Fig. 8A–C). In addition, the percentage of non-capacitated spermatozoa showing pattern C (Fig. 4C) was not different from the culture medium negative control (Fig. 8D). Thus, similar to chemoattraction, the chemorepelled spermatozoa are a subpopulation of capacitated cells, those known to be ready to fertilize the oocyte.
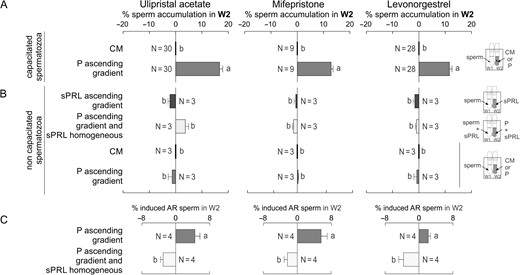
Spermatozoa already prepared to fertilize the oocyte are chemorepelled by sPRL. (A) Spermatozoa previously incubated under capacitating conditions were placed in W1, and culture medium (CM) or 10 pM of progesterone (P) were loaded in W2, determining the percentage of spermatozoa recovered from W2. (B) After seminal plasma removal (non-capacitating conditions), spermatozoa were placed in W1 combined with the following treatments: CM in W2; an ascending gradient of P; an ascending gradient of synthetic Progesterone Receptor Ligands (sPRL); a homogeneous distribution of sPRL combined with an ascending gradient of P. In all conditions, the percentage of spermatozoa in W2 was determined after the SSA. (C) Spermatozoa previously incubated under capacitating conditions were placed in W1 in combination with the following treatments: an ascending gradient of P alone or together with a homogeneous distribution of sPRL. Then, the percentage of induced acrosome-reacted spermatozoa (AR; as an indication of capacitated spermatozoa) was determined in W2. The experiments were performed with the sPRL concentration that showed the highest negative response in (A). Data are expressed as mean ± SEM and the number of independent experiments (N) is shown for each treatment. Different letters (a, b) indicate significant differences between treatments for each sPRL, P < 0.05.
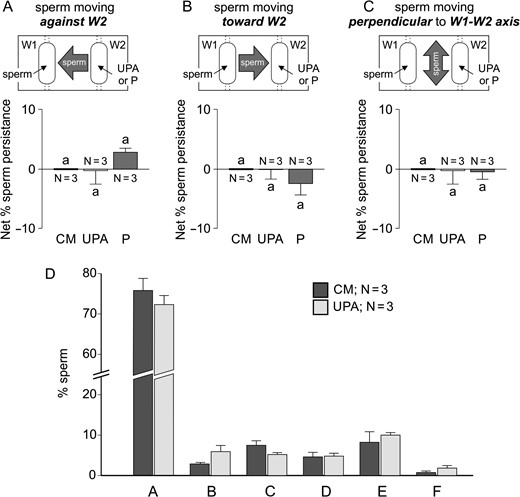
Sperm persistence against UPA and the corresponding repulsive pattern of movement are abolished with non-capacitated spermatozoa. Experiments were performed in a chemoattraction chamber (Fabro et al., 2002) with spermatozoa after removing the seminal plasma which were loaded in W1 and 100 pg/ml of UPA or 10 pM P were placed in W2, which formed an ascending gradient between both wells. Net percentage of sperm persistence was determined in three directions: cells moving against W2 (A), cells moving towards W2 (B), and cells moving perpendicular to the W1–W2 axis (C). The percentage of spermatozoa showing each returning pattern for UPA and culture medium negative control (see the corresponding drawings in Fig. 4A–F) was represented in the bar chart (D). Data are expressed as mean ± SEM and the number of independent experiments (N) is shown for each treatment. No significant differences between treatments were shown, P > 0.05.
sPRL prevent spermatozoa reaching the oocyte
Under in vivo conditions, the ovulated egg secretes progesterone, forming a gradient along the cumulus and beyond which may attract spermatozoa (Teves et al., 2006; Guidobaldi et al., 2008). Taking into account that in the presence of a P gradient, the homogeneous distribution of sPRL stimulates chemorepulsive behaviour, then the incubation of OCC with sPRL could prevent spermatozoa reaching the oocyte. To test this hypothesis, capacitated rabbit spermatozoa were pre-treated with UPA or MIFE (those sPRL showing a higher repellent effect), and then co-incubated with rabbit oocyte–cumulus complex under the continuous presence of sPRL, determining the total number of spermatozoa around the oocyte (Fig. 9A and B). A reduction of ~75% in sperm count was observed when spermatozoa were previously treated with UPA, in comparison to the culture medium control, while no significant reduction was observed with MIFE (Fig. 9C). These results suggest that spermatozoa might be pharmacologically repelled from a non-fertilized egg.
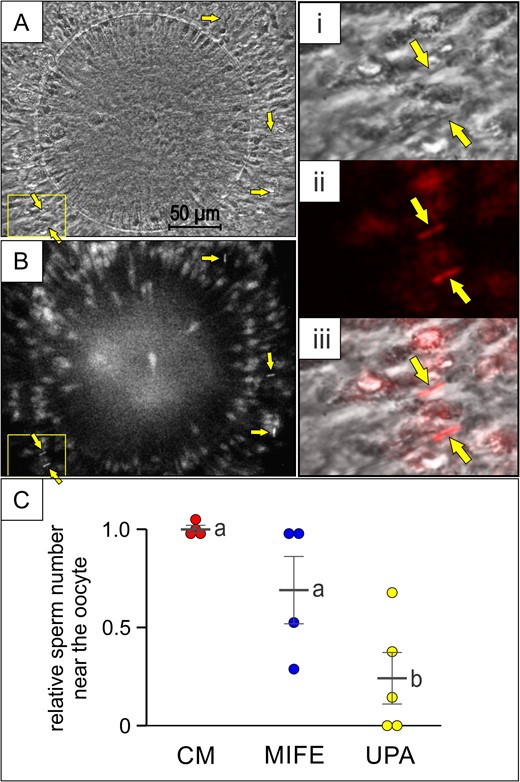
sPRL prevent spermatozoa reaching the oocyte. Rabbit oocyte–cumulus complexes were exposed to rabbit spermatozoa (previously incubated under capacitating conditions and stained with Fluo-4), pre-treated (or not) with 100 pg/ml of mifepristone (MIFE) or ulipristal acetate (UPA); (A and B) phase contrast and fluorescence images of the oocyte–cumulus complex where some spermatozoa are indicated by arrows. The yellow square shows the area amplified on the right of the figure: phase contrast (i), pseudo-coloured fluorescence (ii) and merge (iii). (C) relative sperm number in the area near the oocyte determined in the presence of CM, MIFE or UPA, with a sperm range of 7–14 with a mean value of 11.8 ± 1.6, for CM. Data are expressed as mean ± SEM of independent assays, and individual experiments are represented by circles. Different letters (a, b) indicate significant differences between treatments for each synthetic Progesterone Receptor Ligands (sPRL), P < 0.05.
Zinc, a cation released at fertilization, can mediate sperm chemorepulsion
Sperm chemorepulsion has been hypothetically suggested as a mechanism to avoid polyspermy in vivo (Eisenbach, 2004). Then, a repellent must be released after the penetration of the oocyte by one spermatozoon. Interestingly, ~0.5 mM of zinc is released during the first 2 min after fertilizing an oocyte, which is rapidly homogenized outside the zygote (Kim et al., 2011; Que et al., 2017). Notably, this cation may regulate the chemotactic signalling of invertebrate's spermatozoa (Beltrán et al., 2014). Considering that, immediately after fertilization, the oocyte–cumulus complex is still immersed in a progesterone gradient, we hypothesized that a homogeneous distribution of this cation associated with a gradient of P might chemorepel spermatozoa. We found that spermatozoa exposed simultaneously to a gradient of P and a homogeneous allocation of different concentrations of zinc, were significantly decreased in W2 (Fig. 10A). In addition, the rapid liberation of zinc by the fertilized oocyte can also form a sudden transient gradient of this cation, stimulating chemorepulsion per se. Indeed, when spermatozoa were exposed to an ascending gradient of zinc, decreased sperm accumulation in W2 was also observed (Fig. 10B). A descending gradient of zinc reverted the sperm accumulation in W2 (Fig. 10C), while a homogeneous distribution of zinc caused no sperm accumulation in W2 (Fig. 10D), treatments that were not significantly different from the culture medium negative control (Fig. 10 F). Sperm velocity and the pattern of movement were not affected by zinc, as confirmed by video-microscopy and computer motion analysis (Supplementary Table 2), hence, these parameters did not interfere with the determination of repulsion. In addition, sperm chemorepulsion mediated by zinc appears to be specific, since neither calcium nor magnesium (other bivalent cations) induced sperm chemorepulsion (Fig. 10E). These results suggest that zinc may repel spermatozoa per se or in the presence of a gradient of P.
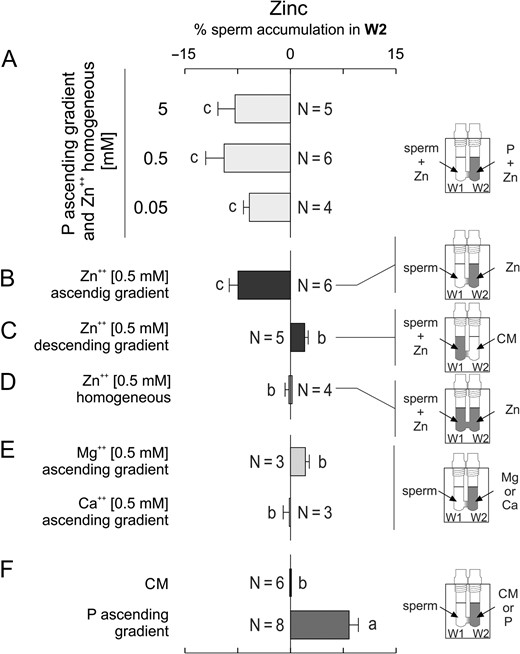
Zinc, a cation released at fertilization, can mediate sperm chemorepulsion. Spermatozoa incubated under capacitating conditions were placed in W1 in combination with the following treatments, determining the percentage of sperm in W2 at the end of the SSA. (A) a homogeneous distribution of several concentrations of zinc (Zn) under an ascending gradient of 10 pM of progesterone (P). (B) an ascending gradient of 0.5 mM Zn; (C) a descending gradient of 0.5 mM Zn. (D) a homogeneous distribution of 0.5 mM Zn. (E) an ascending gradient of 0.5 mM either of magnesium (Mg) or calcium (Ca). (F) culture medium (CM) or an ascending gradient of 10 pM P, as controls. Data are expressed as mean ± SEM and the number of independent experiments (N) is shown for each treatment. Different letters (a, b, c) indicate significant differences between treatments, P < 0.05.
Discussion
To the best of our knowledge, this set of experiments verifies for the first time the occurrence of sperm chemorepulsion. This motility behaviour shares several similarities with sperm chemoattraction. Repulsion seems to be a conserved phenomenon because it was stimulated by very low doses of the repellents in spermatozoa from several mammalian species. The chemorepelled spermatozoa comprise a subpopulation of cells, those physiologically ready to fertilize. Both pharmacological (sPRL) and natural (zinc) repellents counteract the attracting force of progesterone. Apparently, some sPRL prevent spermatozoa from reaching the vicinity of the oocyte.
Somatic cell orientation usually involves a combined action of multiple gradients of the same or different molecules that drive the cell movement once in one direction and then on the opposite one (Huttenlocher and Poznansky, 2008). Mammalian spermatozoa are apparently not an exception. When spermatozoa are transiting through the female genital tract, they are in contact with different molecular gradients which may stimulate different sperm behaviour, such as chemoattraction, (Eisenbach and Giojalas, 2006; Giojalas et al., 2015), or repulsion (this study), depending on the presence of a concentration molecular gradient and the physiological state of the cell. Thus, the similarities found between sperm chemoattraction and chemorepulsion match those observed in somatic migrating cells (Dickson, 2002; Huttenlocher and Poznansky, 2008).
In this study, we observed that spermatozoa can be repelled by an ascending gradient of sPRL and zinc. The variety of the chemical nature of known repellents (proteins, cyclic nucleotides and steroids) is similar to that of chemoattractants. For instance, trichomonas can be repelled by picomolar gradients of steroids (Sugarman and Mummaw, 1988).
In this report, we described and characterized sperm repulsion and its possible role in vivo, but the mechanism that governs this sperm motility leads to many more open questions than facts. For instance, the identity of the sperm-binding protein for sPRL or zinc is not defined yet for sperm chemorepulsion. In the case of zinc, it may interact with a potassium channel located at invertebrate sperm membrane that regulates sperm chemoattraction (Beltrán et al., 2014) or a zinc receptor associated to mammalian sperm capacitation (Michailov et al., 2014). But in the case of sPRL, they are known to bind to the genomic progesterone receptor as described for somatic cells (Chabbert-Buffet et al., 2005). But it was recently reported that the sPRL apparently bind to a progesterone receptor located at the cell surface of human spermatozoa (Zumoffen et al., 2017). However, a more complex situation arises when the repellent effect is caused by homogeneous distribution of the repellent in combination with an ascending attractant gradient of progesterone. In addition, this apparent chemical conflict not only inhibits sperm chemoattraction but also stimulates sperm repulsion. How can this work? Apparently, the repellent converts the chemotactic signalling into a repulsive one. Preliminary observations in our laboratory suggest that the main signalling cascades operating in sperm chemoattraction are also activated during repulsion in humans, rabbits and mice. For instance, the depletion of the intracellular calcium and cAMP, and the inhibition of guanylyl cyclase, abolished sperm chemorepulsion mediated by sPRL (unpublished data). This finding is similar to that observed in somatic cells like neutrophils, where chemoattraction and repulsion are mediated by interleukin 8 which activates the same signalling cascades, but during repulsion the intracellular calcium is significantly increased while the level of cAMP is decreased (Huttenlocher and Poznansky, 2008).
Since only those spermatozoa physiologically ready to fertilize the oocyte can be repelled, the target cell population that can be either attracted or repelled may be the same. This phenomenon may be of biological and medical significance. For instance, what could the role of sperm chemorepulsion be in vivo? One possibility would be the prevention of the entry of a capacitated spermatozoon inside a fertilized oocyte (Eisenbach, 2004). Here we presented evidence supporting this option. Several mechanisms have been proposed to regulate polyspermy. The cortical reaction is the most accepted, which involves exocytosis of the oocyte cortical granules (Gardner and Evans, 2006). Recently, two additional mechanisms have been also proposed to control polyspermy: exocytosis of micro-vesicles of oocyte origin (Saavedra et al., 2014) and removal of the sperm-binding protein Juno from the oocyte membrane (Bianchi et al., 2014). However, these three mechanisms are rather slow since the full process of the sperm blockage takes 30 min to 2 h, depending on the mechanism. Therefore, to rapidly avoid polyspermy, the sperm repulsive behaviour might act as an early complementary event. Since sperm can be repelled by 0.5 mM of zinc, concentration that was observed outside the oocyte immediately after fertilization (Kim et al., 2011; Que et al., 2017), this cation might serve as a natural repellent, operating as a first barrier for capacitated spermatozoa arriving to the fertilized oocyte. Additionally, polytocous species require a mechanism to guarantee the fertilization of all ovulated oocytes (Hunter, 1993). In this context, capacitated spermatozoa can be rejected from the fertilized egg environment, leaving them ready to sense a chemotactic gradient attracting them to an unfertilized egg (Eisenbach, 2004).
Another role of sperm chemorepulsion could be the prevention of fertilization, as part of the mechanism of action of some contraceptives. Of the sPRL tested, UPA, which is a novel emergency contraceptive pill, prevents sperm reaching the oocyte. This result is in apparent conflict with the recent finding of Gómez-Elías et al. (2016) who reported that the administration of UPA did not affect fertilization. However, in that study the timing of in vitro fertilization was too long to preserve a P gradient along the cumulus and over time, necessary to support the repellent effect and prevention of sperm reaching the egg, in the presence of a homogeneous distribution of UPA. Since the introduction of the first combined oral contraceptive, several new drugs and contraceptive delivery systems have been introduced (Blumenthal et al., 2011). However, in some cases, their mechanisms of action are not fully understood (Donnez et al., 2012; Nallasamy et al., 2012; Williams et al., 2012), particularly their effect on sperm physiology. For instance, sperm capacitation and acrosome reaction are not affected by some sPRL (Saboya Brito et al., 2005; Gómez-Elías et al., 2016). Here we provide evidence supporting the hypothesis that, when a gradient of P is generated by an ovulated egg, simultaneously with the availability of low levels of sPRL, fertilizing spermatozoa may be repelled from the oocyte's vicinity, thus preventing fertilization.
This experimental evidence leads us to propose a tentative role for the occurrence of sperm chemorepulsion in vivo. Under natural conditions, when an ovulated egg gets inside the fertilization site, the cumulus cells that surround the oocyte secrete progesterone, forming a concentration gradient along the cumulus and beyond. Thus, those spermatozoa physiologically ready to fertilize may be attracted towards the unfertilized oocyte (Fig. 11A). However, when the oocyte is already fertilized, it may secrete a natural repellent (probably zinc) that, even in the presence of a gradient of P, prevents spermatozoa reaching the oocyte avoiding polyspermy (Fig. 11B). If a sPRL has been taken as a contraceptive (e.g. UPA), it would probably be homogeneously distributed inside the oviduct. Then, under the gradient of P generated by the cumulus cells, capacitated spermatozoa would be chemorepelled from the non-fertilized oocyte (Fig. 11C).
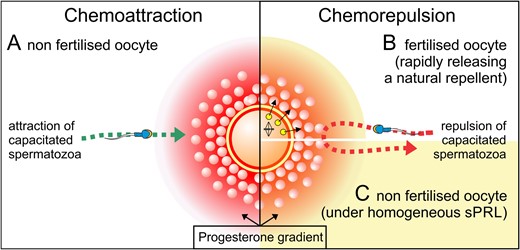
Tentative role for the occurrence of sperm chemorepulsion in vivo. At the cellular level, mammalian reproduction can be regulated by mechanisms that favour or prevent fertilization, in an orchestrated way of two opposed sperm navigation mechanism, chemoattraction and chemorepulsion. Under natural conditions, by sensing a gradient of progesterone capacitated spermatozoa get the location of the egg (A). Once fertilized, a rapid release of zinc prevents the entrance of additional capacitated spermatozoa inside the egg by converting the progesterone gradient into a repulsive one (B). Under pharmacological conditions, sPRL may also convert the attracting progesterone gradient into a repulsive one, preventing capacitated spermatozoa to get inside an unfertilized egg (C).
Thus, sperm chemoattraction and chemorepulsion become cell motility mechanisms that behave as two sides of a coin, as it was similarly observed in somatic cells (Huttenlocher and Poznansky, 2008). In any event, the intriguing molecular mechanism that regulates sperm chemorepulsion and its occurrence in vivo needs to be further investigated. Our results could open new biological and medical frontiers to be scientifically explored.
Supplementary data
Supplementary data are available at Human Reproduction online.
Authors’ roles
H.A.G. designed and performed experiments, analysed data and revised the article. M.C., A.M. and V.M. performed experiments. L.B. designed study and revised article. L.C.G. designed the study and experiments, got financial support, wrote the article.
Funding
Universidad Nacional de Córdoba (Argentina) PCC-UNC-2013.
Conflict of interest
None declared.
Acknowledgements
The authors are indebted to Dr Delphine Levy of HRA Pharma (Paris, France) and Regine Sitruk-Ware (Population Council, USA), for the kind donation of ulipristal acetate. L.C.G. and H.A.G. are researchers from the Consejo Nacional de Investigaciones Científicas y Técnicas (Argentina). M.C. is a Post doctoral fellow of the Agencia Nacional de Promoción Científica y Tecnológica (Argentina). A.M. and M.V.M. are PhD and graduate students of the Universidad Nacional de Córdoba (Argentina), respectively, and fellowship holders of the Consejo Nacional de Investigaciones Científicas y Técnicas (Argentina). The authors thank Julieta Regalado for her contribution with preliminary experiments.
References
Author notes
The authors consider that the first two authors should be regarded as joint first authors.