-
PDF
- Split View
-
Views
-
Cite
Cite
Guohui Zhang, Dongyan Li, Chaofeng Tu, Lanlan Meng, Yueqiu Tan, Zhiliang Ji, Jiao Cheng, Guangxiu Lu, Ge Lin, Huan Zhang, Jinpeng Sun, Mingwei Wang, Juan Du, Wenming Xu, Loss-of-function missense variant of AKAP4 induced male infertility through reduced interaction with QRICH2 during sperm flagella development, Human Molecular Genetics, Volume 31, Issue 2, 15 January 2022, Pages 219–231, https://doi.org/10.1093/hmg/ddab234
- Share Icon Share
Abstract
Sperm fibrous sheath (FS) is closely related to sperm maturation, capacitation and motility, and A-kinase anchor protein 4 (AKAP4) is the most abundant protein in sperm FS. Previous studies found incomplete sperm FSs and abnormal flagella in Akap4 knockout mice. Meanwhile, it was reported that the partial deletion in AKAP4 is highly relevant to the dysplasia of the FS in an infertile man, and so far, there is no report about male infertility caused by hemizygous AKAP4 variant. Furthermore, the specific mechanisms of how the variant is relevant to the phenotype remain elusive. In this study, we investigated three multiple morphological abnormalities of the sperm flagella-affected men from three independent families (including one consanguine family) carried hemizygous c.C1285T variant in AKAP4. The patients carried this variant, which showed dysplastic sperm FS, and the protein expression of AKAP4 was decreased in flagella, which was further confirmed in HEK-293T cells in vitro. In addition, the co-localization and interaction between AKAP4 and glutamine-rich protein 2 (QRICH2) on the molecular level were identified by immunofluorescence and co-immunoprecipitation (CO-IP). The hemizygous c.1285C > T variant in AKAP4 induced decreased protein expression of QRICH2 in spermatozoa. These results suggested that the normal expression of AKAP4 is required for maintaining the expression of QRICH2 and the decreased protein expression of AKAP4 and QRICH2,as well as the interaction between them induced by the hemizygous variant of AKAP4 caused dysplastic fibrous sheath, which eventually led to reduced sperm motility and male infertility.
Introduction
Infertility is a serious worldwide medical and social problem. It is estimated that ~15% of couples are affected by infertility, with male-factor infertility thought to play a role in ~30–50% of infertile couples (1,2). Male infertility mainly ascribes to sperm abnormalities, including oligozoospermia, asthenozoospermia and azoospermia (3). The flagellum is responsible for sperm motility, which plays a key role in normal fertilization. Dysplastic flagella will lead to decreased sperm motility or even immobility, eventually leading to male infertility. Taking the axoneme as the core, which is composed of a basic ‘9 + 2’ microtubule structure, the flagella is divided into three parts: the middle piece, the principal piece and the end piece. In the middle piece, the axoneme are surrounded by outer dense fibers (ODFs) and mitochondrial sheaths, while in the principal piece, they are encircled by ODF and fibrous sheaths (FSs) (4). Using knockout (KO) mice models, numerous genes related to flagella development have been identified. In Tssk deficient mice, morphological defects existed at the junction between the middle piece and the end piece of the flagella (5). In Ropn1 deletion mice, flagella showed structural defects in the principal piece (6). While in Odf2 KO mice, dysplastic axonemes of flagella were discovered (7). Besides, our previous study proofed that loss-of-function variants in glutamine-rich 2 (QRICH2) caused multiple morphological abnormalities of the sperm flagella (MMAF) and male infertility (8).
MMAF is a special type of asthenozoospermia characterized by MMAF such as shortened, bent, curled and irregular flagella, which may cause severe impairment of sperm motility (9–11). Almost, all cases of MMAF are accompanied by disorganized flagella ultrastructure, such as disordered arrangement of axonemes or dysplastic ODF and FSs (10,12,13). Accumulating studies have been devoted to exploring the molecular mechanisms that induce MMAF and found that the etiology of MMAF is heterogeneous, usually caused by a single gene variant (14). Whole-exome sequencing (WES) analysis has uncovered high frequencies of variants in DNAH1, CFAP43 and CFAP44 which are responsible for approximately one-third of all MMAF cases. Other studies involving AK7, CFAP65, CFAP69, CEP135, CFAP58, DNAH8, FSIP2, STK33, DNAH2, SPEF2 and DNAH17 have also provided strong genetic evidence for the occurrence of MMAF (15–25).
A-kinase anchor protein 4 (AKAP4) is the first protein described to be related to sperm flagella in the AKAP family (26) and is the most abundant protein expressed in sperm FSs (27,28). Moreover, AKAP4 plays a key role in completing the assembly of the FS and is responsible for compartmentalizing protein phosphorylation by tethering cyclic adenosine monophosphate (cAMP)-dependent protein kinase A (PKA) regulatory subunits to various cellular structures (29). FS is a unique cytoskeletal structure in sperm flagella, which provides the sperm tail with mechanical supports that modulates flagella bending and defines the pattern of the flagella beat (30). Furthermore, the FSs are assumed to serve as scaffolds for proteins in signaling pathways involved in sperm maturation, motility, capacitation and glycolysis (27). After knocked out of Akap4, the spermatozoa were found with shortened, bent, coiled, pronged flagella and reduced diameter in the principal piece in mice. As a result, the sperm motility was decreased and the male was infertile (28,31). In addition, AKAP4 and AKAP3 gene deletions were detected in an infertile patient with FSs dysplasia (32). These studies suggested that AKAP4 plays a crucial role in maintaining the integrity of FS and normal sperm motility. However, the mechanism is still unclear and no study regarding the hemizygous AKAP4 variant in human male infertility has been reported so far.
In this study, we identified three MMAF-affected men carried hemizygous variant in AKAP4 from three independent families. The possibly pathogenic hemizygous c.1285C > T variant in AKAP4 was investigated through in vivo and in vitro functional study to explore the pathogenicity and molecular mechanism of the variant.
Results
Identification of the c.1285C > T hemizygous variant of AKAP4 in men affected by MMAF
In this study, WES analysis was implemented to identify potential candidate genes associated with MMAF. Remarkably, through the bioinformatics analysis and tissue expression specific evaluation (showed in Supplementary Material, Fig. S1), the c.1285C > T [p.Arg429Cys] hemizygous missense variant of AKAP4 was identified. The detailed information of the variant was shown in Table 1.
Patients . | Gene . | Position . | RefSeq ID . | Variant . | Variant type . | dbSNP ID . | 1000 G . | GO-ESP . | ExAC . | MutationTaster . | SIFT . | Poly-Phen2 . | CADD . |
---|---|---|---|---|---|---|---|---|---|---|---|---|---|
F1a:II-1b F2a:II-1b F3a:II-1b | AKAP4 | ChrX: 49958079 | NM_003886 | c.C1285T p.R429C | Missense | rs372025415 | NAc | 0.000095 | 0.00004566 | Dd | De | Df | 24.3 |
Patients . | Gene . | Position . | RefSeq ID . | Variant . | Variant type . | dbSNP ID . | 1000 G . | GO-ESP . | ExAC . | MutationTaster . | SIFT . | Poly-Phen2 . | CADD . |
---|---|---|---|---|---|---|---|---|---|---|---|---|---|
F1a:II-1b F2a:II-1b F3a:II-1b | AKAP4 | ChrX: 49958079 | NM_003886 | c.C1285T p.R429C | Missense | rs372025415 | NAc | 0.000095 | 0.00004566 | Dd | De | Df | 24.3 |
CADD: Hazard score, 10 points refer to top 10%, 20 refer to top 1%, 30 refer to top 0.1%.
aF1–F3 represent family 1–3.
bII-1 represent the patient number.
cNA, not available.
d‘D’ (‘Disease causing’).
e‘D’ (‘Damaging’).
f‘D’ (‘Probably damaging’).
Patients . | Gene . | Position . | RefSeq ID . | Variant . | Variant type . | dbSNP ID . | 1000 G . | GO-ESP . | ExAC . | MutationTaster . | SIFT . | Poly-Phen2 . | CADD . |
---|---|---|---|---|---|---|---|---|---|---|---|---|---|
F1a:II-1b F2a:II-1b F3a:II-1b | AKAP4 | ChrX: 49958079 | NM_003886 | c.C1285T p.R429C | Missense | rs372025415 | NAc | 0.000095 | 0.00004566 | Dd | De | Df | 24.3 |
Patients . | Gene . | Position . | RefSeq ID . | Variant . | Variant type . | dbSNP ID . | 1000 G . | GO-ESP . | ExAC . | MutationTaster . | SIFT . | Poly-Phen2 . | CADD . |
---|---|---|---|---|---|---|---|---|---|---|---|---|---|
F1a:II-1b F2a:II-1b F3a:II-1b | AKAP4 | ChrX: 49958079 | NM_003886 | c.C1285T p.R429C | Missense | rs372025415 | NAc | 0.000095 | 0.00004566 | Dd | De | Df | 24.3 |
CADD: Hazard score, 10 points refer to top 10%, 20 refer to top 1%, 30 refer to top 0.1%.
aF1–F3 represent family 1–3.
bII-1 represent the patient number.
cNA, not available.
d‘D’ (‘Disease causing’).
e‘D’ (‘Damaging’).
f‘D’ (‘Probably damaging’).
The c.1285C > T variant carried by patients in family 1, 2 and 3 was predicted possibly damaging in polyphen-2, SIFT and mutation taster, with the hazard score of 24.3 points in CADD. These results suggested the possible pathogenicity of the c.1285C > T variant identified in our study.
The detailed information of the pedigrees in which the three patients were identified was shown in Figure 1A. The variant carried by these patients was further verified by Sanger sequencing. All three patients were identified as hemizygote (Fig. 1B). Through further performing sequence alignment analysis, the amino acid affected by the variant were found to be quite conservative across species (Fig. 1B). Besides, the c.1285C > T variant was located in the AKAP4–110 domain on exon 5 of AKAP4 (Fig. 1C). Based on the results of bioinformatics analysis and the clinical phenotype of patients (Table 2), we believed that the hemizygous variant in AKAP4 may be pathogenic and lead to the infertility of the patients.

Genetic and genealogical information of the MMAF-affected men carried hemizygous AKAP4 variant from three families. F1–F3 represent family1–3. I, II and III refer to three successive generations. The numbers 1, 2 and 3 represent patient ID. NC, normal control. (A) Pedigree structure and segregation analysis of three families. Squares represent male pedigree members, circles represent female pedigree members, solid symbols represent members with variant and hollow symbols represent unaffected members. A double horizontal line means consanguineous marriage. The probands are indicated by black arrows. (B) Sanger sequencing verification of AKAP4 variant in three patients and the affected amino acid residues are conserved across species. Arrows indicate the positions of missense variant and the affected conserved amino acids. (C) The precise locations of the variant and changed amino acids in AKAP4. AKAP4 contains two domains: RIIα-binding (amino acid positions 217–233) domain and AKAP 110 (amino acid positions 253–854) domain.
Patients . | Male age . | Male karyotype . | Y-Chromosome . | Semen volume (ml) . | Con. Sperm (106/ml) . | Total Motility: PR + NP(%) . | Progressive motility (%) . | Normal flagella (%) . | Short flagella (%) . | Bent flagella (%) . | Coiled flagella (%) . | Irregular (%) . | Affected spermatozoa (%) . |
---|---|---|---|---|---|---|---|---|---|---|---|---|---|
F1a:II-1b | 32 | 46, XY | No deletion | 2.8 | 2.1 | 26.3 | 8.4 | 3.03 | 15.15 | 21.21 | 33.33 | 27.27 | 96.97 |
F2a:II-1b | 26 | 46, XY | No deletion | 7.2 | 1.5 | 17.9 | 4.2 | 2.55 | 9.82 | 40.36 | 16.36 | 30.91 | 97.45 |
F3a:II-1b | 33 | 46, XY | No deletion | 3.7 | 2.6 | 21.5 | 11.3 | -c | -c | -c | -c | -c | -c |
Patients . | Male age . | Male karyotype . | Y-Chromosome . | Semen volume (ml) . | Con. Sperm (106/ml) . | Total Motility: PR + NP(%) . | Progressive motility (%) . | Normal flagella (%) . | Short flagella (%) . | Bent flagella (%) . | Coiled flagella (%) . | Irregular (%) . | Affected spermatozoa (%) . |
---|---|---|---|---|---|---|---|---|---|---|---|---|---|
F1a:II-1b | 32 | 46, XY | No deletion | 2.8 | 2.1 | 26.3 | 8.4 | 3.03 | 15.15 | 21.21 | 33.33 | 27.27 | 96.97 |
F2a:II-1b | 26 | 46, XY | No deletion | 7.2 | 1.5 | 17.9 | 4.2 | 2.55 | 9.82 | 40.36 | 16.36 | 30.91 | 97.45 |
F3a:II-1b | 33 | 46, XY | No deletion | 3.7 | 2.6 | 21.5 | 11.3 | -c | -c | -c | -c | -c | -c |
aF1–F3 represent family 1–3.
bII-1 represent the patient number.
c‘-’ represents unclear.
Patients . | Male age . | Male karyotype . | Y-Chromosome . | Semen volume (ml) . | Con. Sperm (106/ml) . | Total Motility: PR + NP(%) . | Progressive motility (%) . | Normal flagella (%) . | Short flagella (%) . | Bent flagella (%) . | Coiled flagella (%) . | Irregular (%) . | Affected spermatozoa (%) . |
---|---|---|---|---|---|---|---|---|---|---|---|---|---|
F1a:II-1b | 32 | 46, XY | No deletion | 2.8 | 2.1 | 26.3 | 8.4 | 3.03 | 15.15 | 21.21 | 33.33 | 27.27 | 96.97 |
F2a:II-1b | 26 | 46, XY | No deletion | 7.2 | 1.5 | 17.9 | 4.2 | 2.55 | 9.82 | 40.36 | 16.36 | 30.91 | 97.45 |
F3a:II-1b | 33 | 46, XY | No deletion | 3.7 | 2.6 | 21.5 | 11.3 | -c | -c | -c | -c | -c | -c |
Patients . | Male age . | Male karyotype . | Y-Chromosome . | Semen volume (ml) . | Con. Sperm (106/ml) . | Total Motility: PR + NP(%) . | Progressive motility (%) . | Normal flagella (%) . | Short flagella (%) . | Bent flagella (%) . | Coiled flagella (%) . | Irregular (%) . | Affected spermatozoa (%) . |
---|---|---|---|---|---|---|---|---|---|---|---|---|---|
F1a:II-1b | 32 | 46, XY | No deletion | 2.8 | 2.1 | 26.3 | 8.4 | 3.03 | 15.15 | 21.21 | 33.33 | 27.27 | 96.97 |
F2a:II-1b | 26 | 46, XY | No deletion | 7.2 | 1.5 | 17.9 | 4.2 | 2.55 | 9.82 | 40.36 | 16.36 | 30.91 | 97.45 |
F3a:II-1b | 33 | 46, XY | No deletion | 3.7 | 2.6 | 21.5 | 11.3 | -c | -c | -c | -c | -c | -c |
aF1–F3 represent family 1–3.
bII-1 represent the patient number.
c‘-’ represents unclear.
To further figure out the effects of the hemizygous AKAP4 variant on fertilization and embryo development, we tracked the clinical outcomes of these patients. The detailed information of intracytoplasmic sperm injection (ICSI) of the three patients was shown in Table 3. For patients F1: II-1 and F2: II-1, two embryos were transferred and one embryo was successfully implanted. Subsequently, the two patients successfully had their children. However, the wife of patient F3: II-1 failed to get pregnant despite two well-developed embryos were transferred.
Clinical outcomes of ICSI cycles using the spermatozoa from the patients carried AKAP4 variant
Subject . | F1a: II-1b . | F2a: II-1b . | F3a: II-1b . |
---|---|---|---|
Male age (year) | 32 | 29 | 37 |
Female age (year) | 28 | 31 | 35 |
Number of ICSI cycles | 1 | 1 | 1 |
Number of oocytes injected | 9 | 6 | 4 |
Number (and rate) of fertilized oocytes | 9(100%) | 6(100%) | 3(75%) |
Number (and rate) of cleavage embryos | 9(100%) | 6(100%) | 3(100%) |
Number (and rate) of 8-cells | 9(100%) | 6(100%) | 2(67%) |
Number (and rate) of blastocysts | 9(100%) | 5(83%) | 2(67%) |
Number of transfer cycles | 1 | 1 | 1 |
Number of embryos transferred per cycle | 2 | 2 | 2 |
Implantation rate | 50% | 50% | 0 |
Clinical pregnancy rate | 100% | 100% | 0% |
Miscarriage rate | NAc | NAc | NAc |
Subject . | F1a: II-1b . | F2a: II-1b . | F3a: II-1b . |
---|---|---|---|
Male age (year) | 32 | 29 | 37 |
Female age (year) | 28 | 31 | 35 |
Number of ICSI cycles | 1 | 1 | 1 |
Number of oocytes injected | 9 | 6 | 4 |
Number (and rate) of fertilized oocytes | 9(100%) | 6(100%) | 3(75%) |
Number (and rate) of cleavage embryos | 9(100%) | 6(100%) | 3(100%) |
Number (and rate) of 8-cells | 9(100%) | 6(100%) | 2(67%) |
Number (and rate) of blastocysts | 9(100%) | 5(83%) | 2(67%) |
Number of transfer cycles | 1 | 1 | 1 |
Number of embryos transferred per cycle | 2 | 2 | 2 |
Implantation rate | 50% | 50% | 0 |
Clinical pregnancy rate | 100% | 100% | 0% |
Miscarriage rate | NAc | NAc | NAc |
aF1–F3 represent family 1–3.
bII-1 represent the patient number.
c‘NA’ represent unclear.
Clinical outcomes of ICSI cycles using the spermatozoa from the patients carried AKAP4 variant
Subject . | F1a: II-1b . | F2a: II-1b . | F3a: II-1b . |
---|---|---|---|
Male age (year) | 32 | 29 | 37 |
Female age (year) | 28 | 31 | 35 |
Number of ICSI cycles | 1 | 1 | 1 |
Number of oocytes injected | 9 | 6 | 4 |
Number (and rate) of fertilized oocytes | 9(100%) | 6(100%) | 3(75%) |
Number (and rate) of cleavage embryos | 9(100%) | 6(100%) | 3(100%) |
Number (and rate) of 8-cells | 9(100%) | 6(100%) | 2(67%) |
Number (and rate) of blastocysts | 9(100%) | 5(83%) | 2(67%) |
Number of transfer cycles | 1 | 1 | 1 |
Number of embryos transferred per cycle | 2 | 2 | 2 |
Implantation rate | 50% | 50% | 0 |
Clinical pregnancy rate | 100% | 100% | 0% |
Miscarriage rate | NAc | NAc | NAc |
Subject . | F1a: II-1b . | F2a: II-1b . | F3a: II-1b . |
---|---|---|---|
Male age (year) | 32 | 29 | 37 |
Female age (year) | 28 | 31 | 35 |
Number of ICSI cycles | 1 | 1 | 1 |
Number of oocytes injected | 9 | 6 | 4 |
Number (and rate) of fertilized oocytes | 9(100%) | 6(100%) | 3(75%) |
Number (and rate) of cleavage embryos | 9(100%) | 6(100%) | 3(100%) |
Number (and rate) of 8-cells | 9(100%) | 6(100%) | 2(67%) |
Number (and rate) of blastocysts | 9(100%) | 5(83%) | 2(67%) |
Number of transfer cycles | 1 | 1 | 1 |
Number of embryos transferred per cycle | 2 | 2 | 2 |
Implantation rate | 50% | 50% | 0 |
Clinical pregnancy rate | 100% | 100% | 0% |
Miscarriage rate | NAc | NAc | NAc |
aF1–F3 represent family 1–3.
bII-1 represent the patient number.
c‘NA’ represent unclear.
The hemizygous c.1285C > T variant in AKAP4 induced flagella dysplasia
In this study, all the patients showed significantly reduced sperm motility, in particular progressive motility (average percentage 8% of three patients), far below the criterion of the World Health Organization laboratory manual for the examination and processing of human semen (≤32%). Besides, the semen samples of these patients were obtained and further analyzed. MMAF were observed in the patients by hematoxylin–eosin (H&E) staining and scanning electron microscope (SEM) (Fig. 2A and B). The proportions of each common flagella abnormality were shown in Table 2. Coiled and bent flagella served as the primary types of flagella abnormalities and averagely account for 24.8 and 30.8%. Subsequently, the results of transmission electron microscopy (TEM) showed disordered ultrastructure of the sperm FSs in the AKAP4-variant patient (Fig. 2C). More concretely, some areas of the sperm FS were missing or inhomogeneous (Fig. 2C, shown in black arrows), and the morphology was incomplete compared with the normal person. Moreover, the detailed ‘9 + 2’ structures were shown with disordered or partially missing (microtubule doublet(s) 4–7) (33) in some spermatozoa of AKAP4-variant patient (Fig. 2C, shown in ellipse). Abnormalities of FS and absence of double microtubule were primary ultrastructural abnormalities of flagella and approximately account for 40 and 20%, respectively (Fig. 2D). These results suggested that the hemizygous missense variant in AKAP4 of the patients induced anomalous morphology and ultrastructure of spermatozoa and primarily manifested as abnormal flagella sheaths.
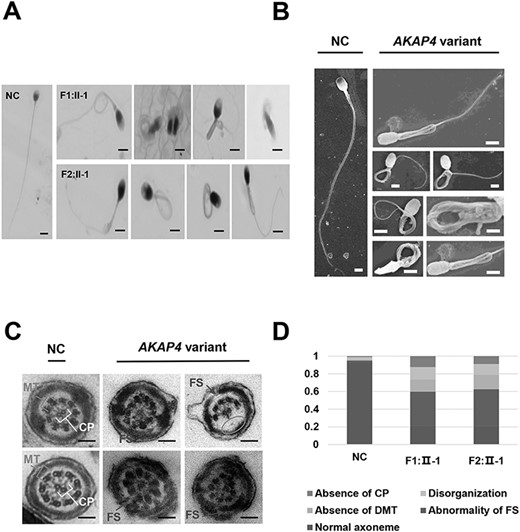
Anomalous sperm flagellar development of MMAF-affected patients carried AKAP4 variant. NC: normal control. AKAP4 variant: men harboring hemizygous AKAP4 variant. F1–F2 represent family 1–2. II-1 represent the patient number. (A) H&E staining of spermatozoa from patients and NC (scale bars, 20 μm). (B) SEM analysis of the spermatozoa from NC and men harboring hemizygous AKAP4 variant (scale bars, 20 μm). (C) The ultrastructure analysis of the sperm flagella from NC and men harboring hemizygous AKAP4 variant using transmission electron microscope (TEM) (scale bars, 200 nm). (D) The proportion distribution of ultrastructural abnormalities of the sperm flagella (1 represents 100%).
The hemizygous c.1285C > T variant of AKAP4 induced decreased protein expression of AKAP4 in sperm flagella.
Considering AKAP4 is localized in the sperm FSs and involved in the assembly of the FSs, we further detected the protein expression of AKAP4 in sperm flagella. By immunofluorescence, we found significantly reduced protein expression of AKAP4 in flagella of the patients carried AKAP4 variant, which was consist with the results of western blot (Fig. 3A). In the patient of F1: II-1, the staining of AKAP4 was almost absent in the flagella, while in F2: II-1, it was significantly reduced. Besides, interestingly, the staining of α-tubulin in F1: II-1 was hypertrophy at the midpiece of the spermatozoa, while the staining of α-tubulin in F2: II-1 was abnormal location. Subsequently, the corresponding plasmids were constructed and transfected into HEK-293T cells in vitro. Western blot showed that the c.1285C > T variant in plasmids significantly reduced the protein expression of AKAP4 compared with the wild-type control (Fig. 3B), which was consistent with the findings in patients and provided possible explanations for the pathogenicity of the missense variant of AKAP4.

Decreased protein expression of AKAP4 was detected in the MMAF-affected patients carried AKAP4 variant. NC: normal control. F1-F2 represent family 1–2. II-1 represents the patient number. (A) Immunofluorescence staining and western blot of AKAP4 in human spermatozoa from NC and the patients. (Red, AKAP4; Green, α-tubulin; Blue, DAPI. Scale bars, 20 μm). (B) The wild type and variant plasmids of AKAP4 were constructed and the protein expression was detected in vitro (p.Arg429Cys represent variant plasmids carried c.1285C > T variant). Gray analysis of protein bands was also showed in B. Data are presented as the Mean ± SEM, n = 3 in each group for protein assay. *P < 0.05, **P < 0.01 versus control.
The interaction between AKAP4 and QRICH2 plays a key role in sperm flagella development
We detected their locations in mature human spermatozoa by immunofluorescence and found that AKAP4 and QRICH2 were co-localized in sperm flagella (Fig. 4A). To further figure out whether AKAP4 and QRICH2 interact with each other and thus have a role in mutual function, the total proteins of human spermatozoa were extracted and the CO-IP experiment in vivo was conducted, showing positive results (Fig. 4B). When AKAP4 or QRICH2 plasmids were separately transfected into HEK-293T cells, AKAP4 was mainly distributed in the regions around the nucleus, while QRICH2 was mostly distributed in the nucleus (Fig. 4C). However, when HEK-293T cells were transfected simultaneously by these two plasmids, both AKAP4 and QRICH2 were distributed in the nucleus. Interestingly, after wild-type AKAP4 plasmids being replaced by mutant AKAP4 plasmids (p.Arg429Cys) in the co-transfection, the original distributions around the nucleus of AKAP4 were observed. These results revealed that the co-localization and interactions at the molecular level between AKAP4 and QRICH2 are important for AKAP4 traffic during the assembly of flagella.
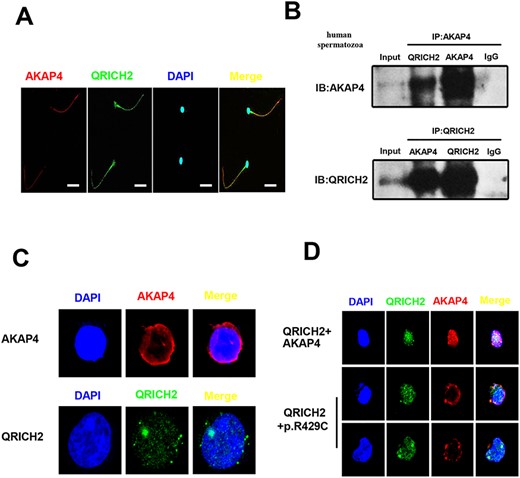
Co-localization and interaction at the molecular level of AKAP4 and QRICH2 in human sperm flagella. (A) AKAP4 and QRICH2 were observed co-localized in human sperm flagella by immunofluorescence staining (Red, AKAP4; Green, QRICH2; Blue, DAPI. Scale bars, 20 μm). (B) The interactions between AKAP4 and QRICH2 in human spermatozoa were detected by Co-IP in vivo. (C and D) The distribution of AKAP4 and QRICH2 by immunofluorescence staining in HEK-293T cells (Red, AKAP4; Green, QRICH2; Blue, DAPI).
After demonstrating the interaction between AKAP4 and QRICH2, we further detected the protein expression of QRICH2 in spermatozoa of the patients carried the c.1285C > T variant. The immunofluorescence and western blot showed consistent results of significantly decreased QRICH2 expression (Fig. 5A and B). Subsequently, QRICH2 and AKAP4 (wild-type AKAP4 and p.Arg429Cys mutant AKAP4) plasmids were co-transfected into HEK-293T cells. The results showed that compared with the group of wild-type AKAP4 plasmids, the protein expression levels of QRICH2 were lower in the group of p.Arg429Cys mutant AKAP4 plasmids when they were co-transfected into HEK-293T cells (Fig. 5C). Moreover, forskolin, an activator of the cAMP-PKA signaling pathway, increased the interactions between AKAP4 and QRICH2 (Fig. 5D). These results suggested that AKAP4 may play a key role in maintaining the normal expression of QRICH2, and their interactions are activated by the cAMP-PKA signaling pathway. However, CFTRinh-172, a proven CFTR inhibitor, showed no effect on the interactions of AKAP4 and QRICH2, indicating that the interactions could be modulated by channels other than CFTR in human spermatozoa (Fig. 5D).

AKAP4 played a role in maintaining the expression of QRICH2. (A and B) The QRICH2 expression of the patient carried c.1285C > T variant in AKAP4 was measured by immunofluorescence staining (A) and western blot (B) (A: Red, β-tubulin; Green, QRICH2; Blue, DAPI; Scale bars, 20 μm. B: NC, normal control; F1: II-1 and F2: II-1represent the patient carried c.1285C > T variant in family 1 and 2). (C) The effects of AKAP4 protein on QRICH2 expression were examined in vitro by western blot (QRICH2, wild type plasmids of QRICH2; AKAP4, wild type plasmids of AKAP4; p.R429C, AKAP4 plasmids with variant of c.1285C > T. (D) The interaction between QRICH2 and AKAP4 was detected by Bret assay in HEK-293T. Data are presented as the Mean ± S.E.M., n = 3 in each group for Bret assay. **P < 0.01 versus control, N means no statistical significance.
The c.1285C > T variant may affect the phosphorylation of S432
As shown in Figure 6A, in the structure of the normal AKAP4, the phosphorylation site S432 was adjacent to R429 and there was a hydrogen bond interaction between them. After the occurrence of the c.1285C > T [p. Arg429Cys] variant, the arginine residue was substituted by cysteine residue, the hydrogen bond interaction between them was disappeared and the cavity volume was expanded by 73.656 Å3. Therefore, we inferred that the c.1285C > T [p. Arg429Cys] variant may affect the phosphorylation of S432 in AKAP4.
![The structure comparison of AKAP4 before and after the C1285T [p. R429C] variant. (A) The structure of normal AKAP4. The R429 residue was shown in green, the phosphorylation site S432 residue was shown in cyan and the hydrogen bond interaction was indicated by yellow dotted line. (B) The C429 residue was shown in rose red and the phosphorylation site S432 residue was shown in cyan. After the occurrence of the c.1285C > T [p. Arg429Cys] variant, the arginine residue was substituted by cysteine residue, the hydrogen bond interaction between them was disappeared and the cavity volume was expanded by 73.656 Å3.](https://oup.silverchair-cdn.com/oup/backfile/Content_public/Journal/hmg/31/2/10.1093_hmg_ddab234/1/m_ddab234f6.jpeg?Expires=1750260200&Signature=v-EXG1HsfIJpnzBq3pmNr2tYTby8LmtfVUFORj-inY3IchAJ7VERUSHWTe9kQzzyFJ~mBSrJmIinmx9YxPnxsKHDp1lhDE83GRdqaZVibUFNAojFN2L8~nQy8oRPS2Lpi98QXIpj~tnwlxOQkMbJzyAl2fi5YyCwQf-4yIeZE~DralBh5J1MwYo1XMdxRnX-tM-fiGA37EXWL34rMZdhCIjUvuNvMu6inUUwcmpwxk40x6OZ2tzem3KhAbFYMAvjaV3YFowQk3P58PXRz-IKJx8BZVucOl8sEnyAyi3IrpppSuwWeGzQGSZ-puM5ipy0QjZ6ieS4k7QSOIasl5016Q__&Key-Pair-Id=APKAIE5G5CRDK6RD3PGA)
The structure comparison of AKAP4 before and after the C1285T [p. R429C] variant. (A) The structure of normal AKAP4. The R429 residue was shown in green, the phosphorylation site S432 residue was shown in cyan and the hydrogen bond interaction was indicated by yellow dotted line. (B) The C429 residue was shown in rose red and the phosphorylation site S432 residue was shown in cyan. After the occurrence of the c.1285C > T [p. Arg429Cys] variant, the arginine residue was substituted by cysteine residue, the hydrogen bond interaction between them was disappeared and the cavity volume was expanded by 73.656 Å3.
Discussion
AKAP4 is the most abundant protein in the sperm FSs (27), and the previous study showed that mature AKAP4 is processed by N-terminal from its precursor, pro-AKAP4, which is expressed in the principal piece of human sperm flagella, and the levels are positively correlated with motility after density gradient centrifugation of the semen (34). Besides, AKAP4 participated in the assembly of sperm FSs, and the absence of AKAP4 induced MMAF (31). Moreover, there were findings suggested that AKAP4 plays an important role in the 4HNE-induced oxidative stress damage, thus strengthening the importance of AKAP4 as a biomarker of sperm quality (35). However, until now, no research about the pathogenicity of hemizygous AKAP4 variant has been reported yet. In this study, for the first time, three patients carried pathogenic hemizygous missense variant of AKAP4 in three MMAF-affected men were identified by WES and comprehensively investigated. The patients mainly presented as asthenozoospermia owing to significantly reduced sperm motility. The dysplastic FSs of these patients might be attributed to the reduced protein expression of AKAP4 induced by AKAP4 variant and were responsible for the low sperm motility and infertility of the patients.
In this study, MMAF was observed and some spermatozoa were accompanied by disordered or incomplete ‘9 + 2’ structures. The results were similar to the discoveries of Baccetti B that anomalous axonemes with missing dynein arms or microtubules were found in an infertile patient with gene deletions in AKAP4 and AKAP3 (32). Failure of the normal development of the axonemes severely disrupted the formation of the FSs and ODFs (36). However, it is still unclear whether the dysplastic FSs affect the formation of axonemes and ODF. The study of Miki et al. (28) and Fang et al. (31) showed no obvious abnormality in the flagella except for the FSs in Akap4 KO mice. The classical point of view was that the FS plays a passive structural role, affecting the degree and shape of flagella bending (30). Therefore, we speculated that the incomplete development of the skeletal FSs might lead to the disarrangement of axonemes owing to the loss of structural supports. Our previous study has demonstrated the key role of QRICH2 in sperm flagella development since QRICH2 deletion induced MMAF and male infertility (8). Furthermore, the proteomic work identified that the deletion of QRICH2 affected the expression of key proteins involved in the flagella assembly. Therefore, we hypothesize that the absence of AKAP4 may affect the expression and functional key genes responsible for flagella development, such as QRICH2, and eventually caused the dysplasia of the axonemes.
In this study, the co-localization and interactions of AKAP4 and QRICH2 in sperm flagella of healthy subjects were confirmed by immunofluorescence and CO-IP. Whether the decreased expression of AKAP4 will affect the expression of QRICH2? The answer is yes and supported by the results in vivo and in vitro. These findings demonstrated the key role of AKAP4 in maintaining the expression of QRICH2.
Our results were mostly consistent with the previous findings that AKAP4 deficiency induced male infertility (28,31,32). However, discrepancies still existed among several studies. In our study, the sperm concentrations of these patients were lower than normal standard, which was consistent with the study of Fang et al. (31), but contrary to the discoveries of Miki that Akap4 KO mice had a higher quantity of spermatozoa in epididymis compared with the wild-type mice (28), while Baccetti et al. (32) found no abnormalities of sperm quantity in AKAP4 deficient patients. The discrepancy of these results probably ascribed to species diversity (i.e. human or mouse), different sperm retrieval methods (i.e. ejaculation or dissociation from epididymis) and gene defect pattern (i.e. point mutation or gene deletions). According to the spermatogenic wave in mice spermatogenesis, the seminiferous tubules can be divided into 12 stages. Testicular sections showed no obvious defects at any stage of spermiogenesis, and all of the components in the wild-type testis were able to be found in the Akap4 KO testis (31). However, the possibility that fewer spermatozoa were generated in AKAP4 deficient patients could not be excluded.
Furthermore, it is generally accepted that AKAPs serve as scaffolds for binding protein complexes involved in regulating several signal transduction processes, including shaping, moving and segmenting (37). AKAP4 is present only in spermatogenic cells and is the most abundantly expressed protein in the FSs of the sperm flagella. A more recent model broadens its roles to anchor PKAs within particular cytoplasmic regions, placing them in proximity to proteins immediately downstream in signal transduction pathways involved in regulating sperm maturation, motility and capacitation (27,28,37). In our study, forskolin, an activator of adenylate cyclase (AC), was found to enhance the binding between AKAP4 and QRICH2, suggesting that the AC-cAMP-PKA pathway was involved in the interactions between AKAP4 and QRICH2. The down-stream pathway warrants further investigation. Furthermore, according to the structural model analysis and prediction, the c.1285C > T [p. Arg429Cys] variant may affect the binding with protein kinase and thus affect the phosphorylation level of S432 in AKAP4, and the phosphorylation change may be a momentous step leading to diminished interaction with QRICH2 and disrupting the signaling pathway critical for flagella assembly.
At present, the outcomes of ICSI in patients with MMAF are not completely clear. In the review of Charles Coutton, partial typical MMAF induced by gene variants, such as CFAP43 and CFAP44, seemed did not affect the ICSI prognosis. However, in the patients carried variants of DNAH17 and CFAP65 (38), the ICSI was performed but failed to get pregnant. Previous studies (28,31) showed consistent results of no obvious morphological abnormalities of acrosomes and nucleus in spermatozoa of Akap4 KO mice with our study, suggesting that it may have no effect on the prognosis of ICSI. In our current study of three patients, F1: II-1 and F2: II-1 successfully had their children assisted by ICSI. However, for F3: II-1, the implantation was failed despite two embryos were successfully transferred. This heterogeneity suggested that different gene variants or variant sites may lead to different ICSI prognosis owing to complex and diverse molecular mechanisms. Even carrying the same variant site, the outcomes of ICSI may also be inconsistent owing to individual discrepancy, including gestational age, psychological and nutritional status during pregnancy, etc. In addition, the future development and health conditions of the fetus assisted by ICSI remained unclear and needed long-term observation and evaluation.
There are still some limitations in our study. First, we merely got the cooperation of two patients and obtaining their spermatozoa for further analysis, the flagella morphology and the expression of AKAP4 in the spermatozoa of other patients remained unclear. Second, we proved that the interactions at the molecular level between AKAP4 and QRCIH2 may be related to the AC-cAMP-PKA pathway, while the regulatory mechanism needed further investigation. Last, there were many differences between previous studies focused on AKAP4 mutations and our present study, such as the effects on sperm quantities, suggesting that different variant loci may lead to different phenotypes since different protein interactors binding with different regions of AKAP4, as well as the modifier genes involved in the different patients; therefore, the possibility of the decreased spermatogenesis induced by specific AKAP4 variant cannot be excluded. Further study investigating this possibility is warranted to better understand the detailed mechanism of how the different variants affect the different functions of AKAP4.
In conclusion, we reported the c.1285C > T [p. Arg429Cys] variant of AKAP4 in three MMAF-affected patients from three independent families. We concluded that the hemizygous missense variant of AKAP4 resulted in the decreased protein expression of AKAP4 and abnormal flagella morphology in spermatozoa, which eventually caused decreased sperm motility and infertility. We further found the key role of AKAP4 in interacting with QRICH2, the crucial protein responsible for flagella development, at the molecular level and maintaining its expression. The decreased protein expression of AKAP4 induced by c.1285C > T [p.Arg429Cys] variant affected the normal expression of QRICH2, aggravating the abnormalities of sperm flagella. Our research is of great value for understanding the pathogenicity of the c.1285C > T variant of AKAP4 and further reveals the key role of AKAP4 in sperm flagella development, providing genetic explanations for the etiology of AKAP4 mutation in MMAF-affected patients.
Materials and Methods
Participants involved in the study
A total of 243 MMAF-affected Chinese men from the Reproductive and Genetic Hospital of CITIC-Xiangya were recruited in this study. All of these individuals displayed typical MMAF phenotypes (i.e. a combination of sperm flagellar defects, including shortened, bent, curled and/or irregular flagella) with no obvious primary ciliary dyskinesia-related symptoms such as sinusitis, pneumonia and bronchitis. By physical examination and hormone analysis, patients with obstruction of vas deferens, abnormal androgen levels and testicular injuries were excluded. No abnormal karyotypes of somatic cells or microdeletions of azoospermia factor on the Y chromosome were found in selected patients. Healthy Han males with sperm concentration ≥15 million/ml and progressively motile spermatozoa ≥32% were recruited as members of the control group in this study.
Ethical approval
The present study was approved by the Institutional Ethics Committees of Central South University and the Reproductive Genetic Hospital of CITIC-Xiangya, China. All participants in this study signed the informed consents.
WES and bioinformatic analysis
Blood samples were obtained from 243 MMAF-affected men. The genomic DNA was extracted using DNA extraction kits (Qiagen, Germany) and then analyzed by WES. Library construction, WES and data analysis were implemented by Beijing Genome Institute in Shenzhen. The variants were identified with the Genomic Analysis Toolkit and annotated by ANNOVAR software, including single nucleotide variants, small insertions and deletions.
The workflow for screening possible pathogenic genes was shown in Supplementary Material, Figure S1. Previous studies reported several homozygous loss-of-function gene variants in MMAF cases, indicating that MMAF follows a chromosome recessive genetic pattern. Therefore, heterozygous gene variants were excluded. By searching in gnomAD (http://gnomad.broadinstitute.org), 1000 Genomes Project (ftp://ftp.1000genomes.ebi.ac.uk/vol1/ftp/release/) and GO-Exome Sequencing Project (ESP) database (http://evs.gs.washington.edu/EVS/), the gene variants with allele frequency ≥0.01 were removed because pathological variants inducing MMAF and male infertility are rare in the human population. Moreover, variants in the noncoding region (except for the typical splice site), synonymous variants in the coding region and missense variants predicted to be harmless by tools, such as PolyPhen-2, SIFT and Mutation Taster, were also abandoned. Subsequently, the selected candidate genes were further assessed in the website of human protein atlas (http://www.protonatlas.org), which contains tissue-specific information of gene expression. The genes specifically expressed in testis were included in our research.
Sanger sequencing
The specific PCR primers of AKAP4 for sanger sequencing verification were designed and showed in Supplementary Material, Table S1. The amplified PCR products were separated and extracted by 2.0% agarose gels and then bilaterally sequenced using the ABI 3730 automated sequencer (Applied Biosystems, USA).
Scanning electron microscopy (SEM) and TEM
Fresh semen samples from healthy men and the patients (F1: II-1, F2: II-1) were washed three times with 1× phosphate buffer and then fixed on poly-l-lysine coated coverslips with 2.5% phosphate buffered glutaraldehyde for 2 h (4°C). The coverslips carrying spermatozoa were washed with distilled water and then dehydrated by a gradient ethanol of 50, 70, 95 and 100%. Subsequently, the specimens were dried with Quorum K850 Critical Point Dryer (East Sussex, UK) and coated with gold particles using an ion sputter coater (Q150RS RotaryPumped, Quorum Technologies, East Sussex, UK). The spermatozoa were finally observed under S-3400N SEM (Hitachi, Japan).
Besides, semen samples from healthy men and the patients (F1: II-1 and F2: II-1) were pretreated and fixed with glutaraldehyde (Sigma-Aldrich, St. Louis, USA) and osmic acid, and then dehydrated with gradient ethanol. Subsequently, samples were embedded with Epon 812, dodecenyl succinic anhydride, methylnaphthalic anhydride and dimethylaminomethylphenol, and then, ultrathin sections (70–90 nm) were double-stained with lead citrate and uranyl acetate. Finally, the images were taken with MegaView III digital camera (Munster, Germany) under HT7700 Hitachi electron microscope for further analysis.
Histology H&E staining
Semen samples from healthy men and the patients (F1: II-1, F2: II-1) were washed and resuspended with 0.5–1 ml saline depending on the amount of sediment. Subsequently, the sperm suspension was smeared and fixed with 4% paraformaldehyde and then dehydrated in ethanol, stained routinely with hematoxylin and eosin for histological examination.
Plasmid construction and cell transfection
The QRICH2 (RefSeq ID: NM_032134) plasmids are obtained from Bio-Atom Biotechnology Co., Ltd (Chengdu, China). In our study, the AKAP4 plasmids (RefSeq ID: NM_003886.3) were synthesized and cloned into pcDNA3.1 (+) vector (including C-terminal flag) by Gene Company (Shanghai, China). All mutant plasmids of AKAP4 were generated by the Fast Mutagenesis System of TransGen Biotech Co., Ltd (Beijing, China) following the instructions. The primer sequences for obtaining mutant AKAP4 plasmids were showed in Supplementary Material, Table S1.
The HEK-293T cell line was obtained from the American type culture collection (ATCC, USA). The 293T cells were cultured in 6-wells cell culture plates and 100 mm cell culture dishes (WHB, China) with basic DMEM medium containing 10% foetal bovine serum (Gibco, USA) and 0.1% penicillin/streptomycin in a humidified incubator at 37°C with 5% CO2. According to the experimental scheme, the AKAP4 and QRICH2 plasmids were transfected into 293T cells for 24–48 h.
Western blot assay
The details for western blot assay were previously described (8). Briefly speaking, collected cells and spermatozoa were lysed for 30–60 min on ice in RIPA lysis buffer (Bioteke, China), which contained the Protease Inhibitor Cocktail (Roche, Switzerland). The total proteins were separated by electrophoresis via 7.5–12.5% SDS-PAGE and then transferred to polyvinylidene difluoride membranes (Millipore, USA) for further immunoblot analysis. The GAPDH (1:5000, Proteintech, China), AKAP4 (1:1000, Thermo, USA) and QRICH2 (1:500, Santa Cruz Biotechnology, USA) primary antibodies were used in our research.
Immunofluorescence
The spermatozoa or the 293T cells were fixed onto slides with 4% paraformaldehyde and then perforated with 0.3% Triton X-100 for 15 min. Subsequently, they were blocked in 5% BSA for 30 min at room temperature. After blocking, the slides were incubated with primary antibodies overnight at 4°C in a wet box, including AKAP4 (1:100, Thermo, USA), QRICH2 (1:50, Santa Cruz Biotechnology, USA) and α-tubulin (1:50, Proteintech, China). Next, the slides were incubated with Alexa Fluor 488 (1:1000, Thermo Fisher, USA) or Alexa Fluor 594 (1:1000, Thermo Fisher, USA)-labeled secondary antibodies for 1 h at room temperature in dark. Then, the slides were washed in 1× PBS twice and counterstained with 4, 6-diamidino-2-phenylindole (DAPI, Sigma-Aldrich, China) to label the nuclei. Images were obtained using a laser scanning confocal microscope (Olympus, Japan).
Co-immunoprecipitation
The extracted total proteins were incubated with 6 μl of target antibodies overnight at 4°C. Next, 50 μl of Protein A/G magnetic beads (Invitrogen, USA) were added into each sample for incubating 2 h at room temperature. After washed three times and resuspended with 1× PBS, the co-immunoprecipitated proteins were eluted by standard 1× SDS sample buffer and heated for 15 min at 95°C. Finally, the proteins were separated in 10% SDS-polyacrylamide gels and then transferred onto PVDF membranes for further immunoblot analysis.
Bioluminescence resonance energy transfer(Bret)
The QRICH2-Rluc and AKAP4-EYFP plasmids were constructed by homologous recombination using Trelief™ SoSoo Cloning Kit (TSINGKE, China). The primers for homologous recombination were showed in Supplementary Material, Table S1. The details for Bret assay were described before (39).
The prediction of the 3D structure of AKAP4 and the effects of the c.C1285T [p.R429C] variant on AKAP4 structure
The 3D structure of AKAP4 was predicted by trRosetta (40), an accurate and fast protein structure de novo prediction software. Then, the predicted structure models were molecular dynamics simulated 50 ns through the software gromacs (41). Next, the predicted structure models were optimized on the software Molecular Operating Environment, which contains repairing the incorrect number of hydrogen atoms, reducing clash and balancing the charge. Subsequently, by inputting the structure in the website of ERRAT (42) (https://servicesn.mbi.ucla.edu/ERRAT/), the quality of the predicted structure models were evaluated. Model 1 with the highest score was selected as the final AKAP4 structure model by comparing model structure quality scores.
Furthermore, the harmfulness analyses of the c.C1285T [p.R429C] variant were performed using PolyPhen-2 tool (43) (http://genetics.bwh.harvard.edu/pph2/). The structural changes that may be caused by the variant were predicted through Missense3D (44) online tool (http://www.sbg.bio.ic.ac.uk/~missense3d/).
Statistical analysis
All statistical analyses were performed using the SPSS 17.0 software (IBM Company, USA). The Student’s t-test was used to compare the parameters between the experimental groups. The P-values less than 0.05 were considered significant.
Acknowledgements
Thanks to all the researchers for their contribution to the current research. Guohui Zhang, Dongyan li and Chaofeng Tu were responsible for the experiments involving human sperm and cells in vitro. Lanlan Meng, Guangxiu Lu, Ge lin and Huan Zhang were responsible for the collection and analysis of clinical data of the patients. Zhiliang Ji and Jiao Cheng were responsible for predicting the effects of the variants on the structure of AKAP4 using bioinformatics methods and tools. Jinpeng Sun and Mingwei Wang were responsible for the Bret assays. Yueqiu Tan, Juan Du and Wenming Xu were responsible for experimental design and article revision.
Conflict of Interest statement. The authors declare no conflict of interest related to this study.
Funding
National Key R&D Program of China (2018YFC1003603 (to W.X.), 2018YFC1004900 (to Y.T.); the National Natural Science Foundation of China (81971445 (to W.X.), 81771645, 81971447 (to Y.T.).
References
Author notes
The authors wish it to be known that, in their opinion, the first two authors should be regarded as joint First Authors.