-
PDF
- Split View
-
Views
-
Cite
Cite
S. A. Pisarevsky, D. P. Gladkochub, T. A. Donskaya, B. de Waele, A. M. Mazukabzov, Palaeomagnetism and geochronology of mafic dykes in south Siberia, Russia: the first precisely dated Early Permian palaeomagnetic pole from the Siberian craton, Geophysical Journal International, Volume 167, Issue 2, November 2006, Pages 649–658, https://doi.org/10.1111/j.1365-246X.2006.03160.x
- Share Icon Share
Summary
New palaeomagnetic and geochronological data from mafic dykes emplaced into the basement of the Siberian Craton on the southwestern coast of the Baikal Lake are present. The concordant zircon U–Pb SHRIMP age of these dykes is 275 ± 4 Ma. Palaeomagnetic study of the dykes isolated a stable primary remanence with steep upward direction supported by a positive baked contact test. The palaeomagnetic pole (50.5°N, 121.4°E, A95= 16.7°) is the first precisely dated Siberian pole between ~360 and 260 Ma. It falls near the interpolated Siberian APWP and suggests that Siberia had not joined Eurasia by 275 Ma. The studied dykes are the first reported evidence of Permian magmatic activity in the cratonic part of southern Siberia. They are probably related to the prominent 300–260 Ma magmatic event recognized in Trans-Baikalia. The comparison of our new result with recently published palaeomagnetic data from Trans-Baikalia suggests that differential movements between the Siberian Craton and blocks of Trans-Baikalia around 275 Ma are unlikely. This confirms tectonic models considering Trans-Baikalia as an Andean-type active margin of the Siberian continent in Permian times.
1 Introduction
The Pangaea supercontinent was assembled by the Late Palaeozoic through the closure of the Rheic Ocean between Gondwana and Laurussia (McElhinny & McFadden 2000). At around the same time, the Siberian Craton and Kazakhstan microcontinent joined Laurussia through the closure of the Uralian Ocean (e.g. Puchkov 1997). However, the precise history of ocean closure is not known because of a lack of precisely dated reliable Early Permian palaeomagnetic poles from the Siberian Craton (Smethurst et al. 1998). Only 10 Siberian palaeopoles, with estimated ages between 300 and 260 Ma, are present in the latest version of the Global Palaeomagnetic Database (Pisarevsky 2005), and all of them are poorly dated and derived from blanket cleaning experiments. Because of their dubious reliability, Smethurst et al.(1998) did not include any of them in their compilation and construction of the Siberian Apparent Polar Wander Path (APWP). More recently, Kravchinsky et al. (2002) reported a palaeomagnetic pole from the Alentuy Formation (the sampling location is shown in Fig. 1b) of the Trans-Baikal area, supported by a positive fold test. As nearly all of their samples yielded steep upward remanence, they suggested that the Alentuy Formation corresponds to the end of the Kiaman superchron (>265 Ma according to the new geologic timescale of Gradstein 2004). Kravchinsky et al. (2002), assuming that the Siberian Craton was already an integrated part of Eurasia, compared their pole with the Eurasian APWP of Van der Voo (1993) constructed from European poles. They suggested a possible ~29° rotation of the Alentuy area with respect to the Siberian Craton. However, the difference between the Alentuy pole and the Eurasian APWP may also reflect that the Siberian Craton was not yet completely integrated into Eurasia.
Large-scale magmatic events of Carboniferous–Permian age are reported from Trans-Baikalia (e.g. Zorin 1999; Litvinovsky et al. 2002; Yarmolyuk et al. 2002). Granitoids and bimodal volumecanics of ~300–260 Ma are found in wide NE striking strips in Trans-Baikalia (Fig. 1b), including two large dyke belts (Shadaev et al. 2005), but no traces of this magmatic event has yet been reported from the cratonic part of south Siberia.
Here we present the results of a palaeomagnetic study and U–Pb zircon geochronology of a series of mafic dykes exposed near the southwestern corner of Baikal Lake (Figs 1c and d). Our data show that these dykes are coeval with the Trans-Baikalian 300–260 Ma magmatic event, and their palaeomagnetism provides the first precisely dated 275 Ma Siberian palaeopole.
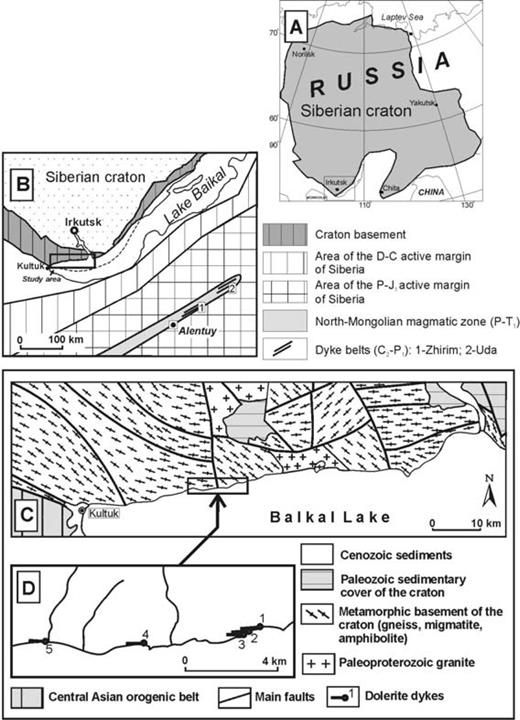
(a) general scheme of the Siberian craton; (b) sketch of the main unites of southern Siberia craton and adjacent areas of Tran-Baikalia; areas of the Siberian margins are from Zorin (1999); positions of 300–285 Ma dyke belts are from Shadaev et al.(2005); North Mongolian magmatic zone—from Yarmolyuk et al. (2001); Alentuy stands for the locality of the palaeomagnetic study of Kravchinsky et al. (2002); (c) geological scheme of the southern flank of the Siberian craton and (d) locations of dykes.
2 Geology and Sampling
The studied area (Fig. 1) belongs to the Sharyzhalgai metamorphic massif, which comprises Archaean protoliths metamorphosed around 1880–1860 Ma (Sklyarov et al. 2003; Didenko et al. 2003; Poller et al. 2005). Five dolerite dykes intruded into the metamorphic basement are exposed along the Baikalian coast between Kultuk and Port Baikal (Figs 1c and d). They are 1–3 m thick, subvertical, striking E–W (260°–280°), and are undeformed. The dolerites are coarse grained in central parts and fine grained along their chilled margins. They have porphyritic textures with olivine and feldspar phenocrysts mainly between 0.03 and 0.8 mm in size. Eskin et al. (1988) published a ~500 Ma K–Ar age for these dykes. 43 oriented block samples were collected from the dykes, baked contacts and country rocks using magnetic compass for orientation. Two 2 cm side cube specimens were cut from each sample.
A sample (sample 6077) was collected from the coarse–grained central part of dyke 2 for geochronology.
3 Analytical Techniques
3.1 Geochronology
Zircons were separated from the sample 6077 using standard techniques, then hand picked under a binocular microscope, and mounted together with grains of BR266 zircon standard in an epoxy resin mount. The mount was polished to expose the grains, thoroughly cleaned and coated with a thin gold coat. The mounted zircons were imaged using optical, backscatter electron (BSE) and cathodoluminescence (CL) techniques, to reveal internal features. The mount was loaded in the sensitive high resolution ion microprobe (SHRIMP) sample lock, and held at high vacuum 24 hr prior to analysis to allow it to degas. U–Th–Pb ratios were determined relative to the standard zircon (BR266: age 559 Ma, 909 ppm 238U, see Stern 2001). Analyses on standard were interspersed with unknowns at a 1:3 ratio. Decay constants used are those recommended by Steiger & Jäger (1977). Measured compositions are corrected using measured 204Pb. Extremely small amounts of 204Pb resulted in small corrections, and a common Pb composition using an age of 1800 Ma and following the model of Stacey & Kramers (1975) was used. Each analysis spot was rastered with the primary beam for 3 min to minimize surface related Pb contamination. Each analysis was conducted in six cycles of mass scans, using a set of primary beam apertures resulting in a slightly elliptical spot of 30 μm. Several analyses were abandoned after the first scan, based on high counts on 204Pb indicating high proportions of non radiogenic (common) lead. The data was reduced using Squid and Isoplot programs (Ludwig 2001a,b). Individual analyses are reported at 1σ confidence level, while pooled analyses [concordia ages (Ludwig 1998), weighted mean 207Pb/206Pb and 206Pb/238U ages] are reported at 95 per cent confidence level. The 2σ error on standard is reported in the data table, but not included in the age calculations. All error bars on the Tera–Wasserburg U–Pb evolumeution plot are at 2σ confidence level.
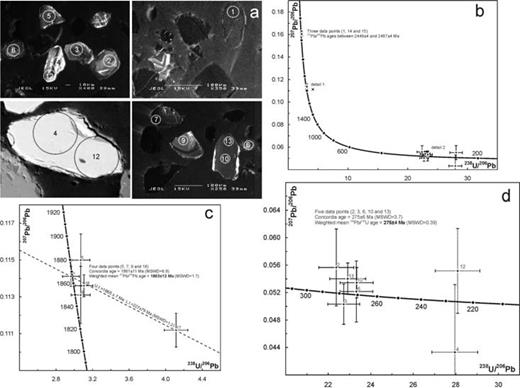
(a) CL and BSE imagery for zircons of sample 6077 showing analysed SHRIMP spots; (b–d) zircon U–Pb data for sample 6077, error crosses are at 2σ confidence level, dashed error crosses denote excluded data points.
3.2 Palaeomagnetism and Rock Magnetism
Remanence composition was determined by detailed stepwise thermal demagnetization (≤20 steps, to 600°C), using a Magnetic Measurements thermal demagnetizer and the 2G-755R cryogenic magnetometer. Stepwise alternating field (AF) demagnetization (≤26 steps, up to 160 mT) was also applied, using the 2G-600 automated degaussing system. To monitor possible mineralogical changes during heating, magnetic susceptibility was measured in selected samples after each heating step using a Bartington MS2 susceptibility meter. Magnetic mineralogy was investigated from demagnetization characteristics and, in selected samples, from detailed variation of susceptibility versus temperature (20° to 700°C) obtained using the Bartington meter in conjunction with an automated Bartington furnace. Magnetization vectors were isolated using Principal Component Analysis (Kirschvink 1980). All vectors were defined with a minimum of four data points and a maximum angular deviation (MAD) of 10 degrees.
4 U–PB Dating
13 zircons were extracted from sample 6077, and they are sub to euhedral in shape (Fig. 2a). The zircons range in size from 50 to 150 μm and have length to width ratios between 1:1 and 2:1. U and Th values fall within the ranges 129–1093 and 5–804 ppm, respectively, resulting in highly variable Th/U ratios between 0.01 and 2.28. The lowest Th/U ratios are recorded in the analyses corresponding to the oldest zircon (analyses 1, 14 and 15), which define concordant 207Pb/206Pb ages of 2449 ± 4, 2460 ± 10 and 2487 ± 4 Ma(1σ confidence level) (Fig. 2b). Four concordant analyses of a second cluster of five data points (5, 7, 9, 16 and 17) define a concordia age of 1861 ± 11 Ma (MSWD = 6.9) and weighted mean 207Pb/206Pb age of 1863 ± 12 Ma (MSWD = 1.7) (Fig. 2c). Analysis 17, plots well away from the concordia curve, allows a regression with the four concordant points yielding an upper intercept of 1865 ± 7 Ma, and an imprecise lower intercept at 227 ± 79 Ma (MSWD = 1.2).
The youngest cluster of ages define a concordant group corresponding to a concordia age of 275 ± 6 Ma (MSWD = 3.7) and a weighted mean 206Pb/238U age of 275 ± 4 Ma (MSWD = 0.39) (Fig. 2d). Analyses 4 and 12 were taken on a single small zircon, displaying similar growth patterns and CL response as those corresponding to an age of 275 ± 4 Ma, but have 206Pb/238U ratios defining a much younger age of ca. 227 Ma. A closer look at these two analyses reveals: (1) the SHRIMP spot was larger than the width of the zircon in both cases, resulting in an overlap of the spot with the resin (Fig. 2) and (2) the zircon is overgrown by a small, micronsized high-CL rim that was analysed within each of the two spots. Based on these observations, we doubt the validity of these analyses on this particular zircon.
We conclude that xenocrystic components provide the ages of basement of 2.49–2.45 Ga and 1863 ±12 Ma. These xenocrystic ages correspond to known Archaean and Palaeoproterozoic igneous and metamorphic events in the area (Poller et al. 2004, 2005; Donskaya et al. 2002; Didenko et al. 2003; Gladkochub et al. 2005). We interpret the weighted mean 206Pb/238U age of 275 ± 4 Ma, calculated from the younger group of analyses, but excluding two dubious analyses on one zircon (analyses 4 and 12), to represent the best age estimate of crystallization of primary zircon in sample 6077. The complete set of SHRIMP results is shown in Table 1.
5 Magnetic Minerals and Rock Magnetism
The natural remanent magnetization (NRM) of the dolerites ranges from 0.2 to 4.5 A m−1, and their magnetic susceptibility from about 8 to 30 × 10−3 SI units. Susceptibility versus temperature curves (Fig. 3) show that Curie points are distributed between 430° and 550°C, which corresponds to a stoichiometric titanomagnetite Fe3–xTixO4 with x ≈ 0.08–0.28 (fig. 3.11 in Dunlop & Özdemir 1997).
Thin section and microprobe analyses (LEO1430VP) show that the main minerals are olivine, diopside, plagioclase (labradorite—andesine in composition) and minor amounts of biotite and feldspar (including ternary feldspar). Accessories are titanomagnetite, ilmenite, chromium-spinel, and zircon.
6 Palaeomagnetism
6.1 Dykes
Both thermal and AF demagnetizations isolated a single stable remanence component carried by low-titanium titanomagnetite. After removal of a low-stability, randomly oriented overprint from most samples, all samples exhibit a steep upwards magnetization (Figs 4a–e and 5a). The relatively high coercivity of these dolerites also indicates that some single domain (SD) or pseudo–single domain titanomagnetite is the carrier of the most stable part of remanence—typically 60 to 120 mT was required for the AF–demagnetization (Fig. 4a), but sometimes the required value increased up to 160 mT. Exclusive reverse polarity of the stable remanence suggests that these dykes were magnetized during the Kiaman magnetic superchron (312–265 Ma), which is confirmed by the 275 Ma U–Pb age of the dykes. Mean remanence directions and virtual geomagnetic poles (VGPs) for all dykes are shown in Table 2 and in Figs 5(a) and 6.
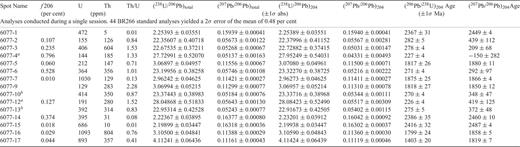
Analyses conducted during a single session. 44 BR266 standard analyses yielded a 2σ error of the mean of 0.48 per cent. f 206 denotes the proportion (per cent) of non radiogenic 206Pb in total 206Pb. Ratios are reported as total (including non-radiogenic Pb) and as 204-corrected. Ages are reported as 204-corrected.
Superscripts ‘a’ and ‘b’ refer to a couple of analyses conducted on two single zircon grains.
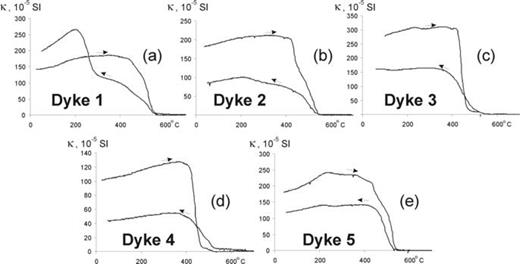
Analysis of Curie points using magnetic susceptibility versus temperature curves. Sample numbers: (a) 1–7; (b) 2–7; (c) 3–4; (d) 4–4 and (e) 5–6.
6.2 Baked Contact Test
Eight samples (16 specimens) were collected from the country rocks. Three of them (1–9, 2–8, and 2–9) were located within 15 cm from contacts with dykes 1 and 2 correspondingly. These samples are completely remagnetized by the dykes (Figs 4f and 5b) and yield stable SD magnetite-bearing remanence with directions close to those of the dykes. Two samples (1–8, 1–10) were collected at some distance (>2 m) from the dykes. Their remanence direction is significantly different from the dykes (Figs 4g and 5c). Three other samples (3–8, 4–8 and 5–8) were taken at distances between 30 and 50 cm from dyke contacts. Specimens 3-8-1 and 4-8-1 are completely remagnetized by the dykes, specimen 5-8-1 yields the direction characteristics of the country rocks, and specimens 3-8-2 and 4-8-2 posses a two-component remanence—a less stable steep upwards component, characteristic for the dykes, and a more stable ‘country’ direction (Fig. 4h). The remaining specimen 5-8-2 yields an unstable remanence.
Stereoplots of baked and unbaked country rocks are shown in Figs 5(b) and (c), respectively. The remanence direction of not remagnetized country rocks is similar to the mean direction of ~1854 Ma (39Ar-40Ar, hornblende) granitoids of the Sharyzhalgai massif (Didenko et al. 2003), shown also in Fig. 5(c) with a ‘diamond’ symbol D = 177.4°, I = 24.6°. The studied country rocks also belong to the Sharyzhalgai massif, and the similar metamorphic age is indirectly confirmed from inherited zircons in the studied dykes (see above). Hence, the positive bake contact test confirms that the remanence of the dykes is primary.
7 Discussion
Our new palaeopole lies near the Permian part of the Siberian APWP of Smethurst et al. (1998) (Fig. 6). However, Smethurst et al. (1998) did not find any reliable Siberian poles between ~360–370 Ma (poles of Kamysheva 1973 in Smethurst et al. (1998); Pisarevsky & Iosifidi (1981)) and ~250 Ma (several poles from the Siberian traps). Their APWP is the result of a spline interpolation between these two time slices. Fig. 6 shows this fragment of the Siberian APWP as a dashed line. It also shows two mean poles from the Siberian traps calculated in two independent compilations (54.7°N, 140.5°E, A95= 5.0° calculated by Torsvik & Andersen 2002; and 49.9°N, 144.8°E, A95= 3.8° calculated by Gurevitch et al. 2004). The circle of confidence of our pole does not overlap with that of the coeval European pole, which means that Siberia probably did not dock with Eurasia until after 275 Ma. This is in accord with the 280 Ma global reconstruction of Torsvik & Cocks (2004) and most other reconstructions. However, we realize that our pole is averaging palaeomagnetic data from five dykes, which may result in incomplete averaging of the secular variations and causing a relatively large circle of confidence. Unfortunately, no other dykes of similar composition and age have yet been found in the area. Both mean poles for the Siberian traps are closer to their European 250 Ma counterpart, but their circles of confidence are still not overlapping, which means that the docking of Siberia was not completed by ~250 Ma. The reconstruction of Europe and Siberia, based on the fit of our new Siberian pole and the mean 275 Ma European pole of 43.58°N, 166.06°E (Torsvik et al. 2001) is shown in Fig. 7 (option A). However, the configuration similar to the one of Torsvik & Cocks (2004) at 280 Ma is also permissible (Fig. 7, option B), as circles of confidence of the poles are still overlapping in this case.
Volumeuminous Carboniferous–Permian magmatism in Trans-Baikalia is widely discussed in recent publications (e.g. Zorin 1999; Litvinovsky et al. 2002; Yarmolyuk et al. 2002; Tomurtogoo et al. 2005). In particular, Shadaev et al. (2005) reported two 300–285 Ma dyke belts striking NE (Fig. 1b). Some authors (Yarmolyuk et al. 2000) associate this magmatism with a mantle plume, whilst others suggest an active margin setting involumeving delamination and asthenospheric upwelling (Tomurtogoo et al. 2005). However, both scenarios suggest that this part of Trans-Baikalia was already a part of Siberian continent by the Carboniferous. Consequently, it has always been enigmatic why no traces of this magmatic event were found in the nearby cratonic part of Siberia (Fig. 1). Baikal dykes of this study may represent these missing traces. Their W–E strikes are ~45° different from the NE strike of 300–285 Ma Zhirim and Uda dyke belts (Shadaev et al. 2005) shown in Fig. 1(b).
This orientation of two sets of dykes may look like two branches of the radial dyke swarm characteristic for mantle plumes. On the other hand, both sets roughly follow the contemporary margin of the Siberian continent, which is more in accord with an active margin settings.
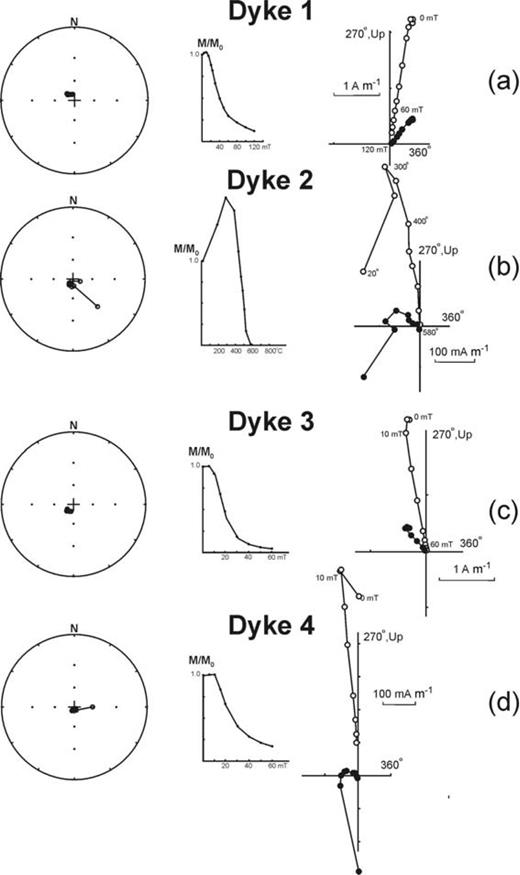
Stereoplots and examples of demagnetization behaviour for studied dykes. In orthogonal plots, open (closed) symbols show magnetization vector endpoints in the vertical (horizontal) plane; curves show changes in intensity during demagnetization. Stereoplots show upwards (downwards) pointing palaeomagnetic directions with open (closed) symbols. Sample numbers: (a) 1-1-1; (b) 2-2-2; (c) 3-7-2; (d) 4-1-2; (e) 5-5-2; (f) 1-9-2; (g) 1-8-2 and (h) 4-8-2.
Kravchinsky et al. (2002) reported palaeomagnetic results from the Permian Alentuy Formation (location is shown in Fig. 1). Their palaeopole (63.1°N, 151.0°E) also plots off the coeval part of the Eurasian APWP (Fig. 6), but it is distinct from the pole of our study. However, the circles of confidence of our new 275 Ma cratonic pole and the Alentuy pole of Kravchinsky et al. (2002) overlap (Fig. 6). This suggests the proximity of the Trans-Baikalian area to the Siberian craton, but also may imply some post–Cretaceous rotational movements as reported by Cogné et al. (2005).
8 Conclusions
- Mafic dykes from the southwestern coast of the Baikal Lake are precisely dated at 275 ± 4 Ma on SHRIMP. These dykes represent a previously missing indication of the vast 300–260 Ma Trans-Baikalian magmatic event in the southern part of the Siberian craton.Table 2.
Sample mean palaeomagnetic directions from mafic dykes of the south Baikal area.
Figure 5.Stereoplots of the directions and mean directions of ChRM from: (a) dykes; (b) baked contacts and (c) country rocks, the mean direction of Sharyzhalgai granitoids (Didenko 2003) is shown by diamond.
The studied dykes carry a primary steep upward remanence, the palaeomagnetic pole falls near the interpolated Permian fragment of the Siberian APWP. Comparison of this pole with the European APWP suggests that Siberia did not dock with Eurasia by 275 Ma. New palaeomagnetic data support most of Early Permian palaeogeographic reconstructions.
Comparison of our new pole with a recently published result from Trans-Baikalia suggests that most of Trans-Baikalia was the margin of the Siberian continent in the Permian.
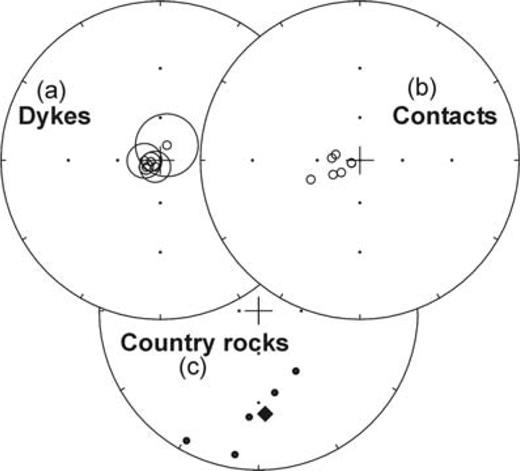
Late Palaeozoic palaeomagnetic poles from Siberia. Star: 275 Ma south Baikal pole (this study); triangle: Alentuy Formation pole (Kravchinsky 2002); square: mean pole for Siberian traps (250–251 Ma) calculated by Torsvik & Andersen (2002); diamond: mean pole for Siberian traps (250–251 Ma) calculated by Gurevitch (2004); circles European poles between 300 and 250 Ma (with 5 Myr step) from the APWP of Torsvik (2001); dashed line with ages in italics—a fragment of Siberian APWP between ~360 Ma and ~250 Ma interpolated by Smethurst (1998).
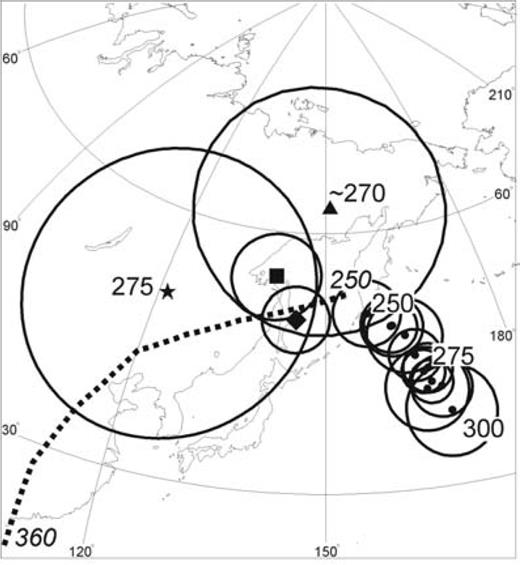
Reconstructions of Siberia and Europe at 275 Ma. Option A—exact match of the Baikal dykes pole (this study, circle of confidence is shown by a solid line) and the 250 Ma pole from European APWP of Torsvik et al. (2001); option B—permissible reconstruction with slightly overlapping circles of confidence (circle of confidence for the Baikal dykes pole is shown by a dashed line).
Acknowledgments
We thank Peter Cawood for the informal review of our manuscript. We are also grateful to Conall Mac Niocaill and Jean–Pascal Cogné for their thorough reviews. This research was supported in part by grants from the Russian Foundation for Basic Research (# 04–05–64412), the Russian Science Support Foundation, and the Russian Ministry of Education (## MD1720.2005.05, NSH7417.2006.5). TSRC publication #383.
References