-
PDF
- Split View
-
Views
-
Cite
Cite
N. Halim, Y. Chen, J. P. Cogné, A first palaeomagnetic study of Jurassic formations from the Qaidam basin, Northeastern Tibet, China—tectonic implications, Geophysical Journal International, Volume 153, Issue 1, April 2003, Pages 20–26, https://doi.org/10.1046/j.1365-246X.2003.01860.x
- Share Icon Share
Summary
We present a new palaeomagnetic study on Upper Jurassic red beds collected at nine sites near Huatugou (38.46°N, 90.75°E) in the Qaidam basin. Thermal demagnetization up to 690 °C shows both low- and high-temperature components (LTC and HTC, respectively). LTC seems to be a recent overprint, HTC, carried principally by haematite and presenting a sole reverse polarity, passes the fold test at the 95 per cent confidence level. The palaeomagnetic pole calculated from the tilt-corrected overall mean direction (Ds= 220.4°, Is=−34.8°, α95= 7.5°) is situated at 50.1°N, 198.0°E (dp/dm= 5.0/8.6). The comparison of this result with coeval palaeomagnetic poles from Tarim, the North China Block (NCB) and the apparent polar wander path of Eurasia reveals a large-scale tectonic evolution of the Asian continent since the late Jurassic. This study suggests that the northward convergence of the Qaidam block is statistically negligible with respect to Tarim (4.5°± 8.2°) since Late Jurassic times. However, a significant relative clockwise rotation of the studied area with respect to Tarim seems to have occurred (16.2°± 11.2°). Whether this rotation is representative of the whole Qaidam basin or is of a local character is yet to be confirmed. The important feature of this study resides in the difference of northward displacement of the Qaidam block with respect to Eurasia on one hand and with respect to the NCB on the other hand. The overall NS convergence absorbed between Qaidam and Eurasia is 19.3°± 9.2°, whereas that absorbed between the Qaidam and the NCB is 8.0°± 6.2°. We suggest that the latter value is the result of the India–Asia collision, while its difference with respect to the former would be absorbed during the Mongol–Okhotsk ocean closure at the end of the Jurassic or at the Jurassic–Cretaceous boundary at the latest.
1 Introduction
Eurasia offers a good opportunity to examine plate convergences and block accretions of the modern tectonics at the lithospheric scale. From India to the south to Siberia to the north, paleomagnetism has allowed the Eurasian continent to be divided into smaller continental blocks that evolved independently from one another, and where the past relative assemblage was different from the present one. The continuous increase in geological and especially in palaeomagnetic data progressively improves our knowledge of Asian history. It is striking, however, that the palaeomagnetic sampling density of the different Eurasian blocks is far from uniform. An illustrative example is the contrast between the Cretaceous cover of the South China Block (SCB), which is based on sampling spread all around the block boundaries and inside the block, and the coeval cover of the Qaidam on which nothing is available in concrete terms in the literature. This inequality of the palaeomagnetic sampling density of the different Asian blocks is not limited to Cretaceous times, but can be observed for the Jurassic as well. Indeed, the North China Block (NCB) and the South China Block are the two main blocks that have been targeted by Jurassic palaeomagnetic studies. For example, Huang & Opdyke (1991) and Yokoyama et al. (2001) worked on Jurassic samples from the SCB, whereas Gilder & Courtillot (1997) studied a Jurassic collection from the NCB. These studies aimed to better constrain the timing of the collision between these two blocks, which was thought to have ended by the Late Jurassic, but for which the recent study by Yokoyama et al. (2001) now suggests a Lower Cretaceous age. Other efforts have focused on the Jurassic using of Eurasia such as those of Yang et al. (1992) or Gilder et al. (1993), but the general tendency of the Jurassic palaeomagnetic studies in Asia resides mainly in the understanding of the SCB–NCB collision.
The Cretaceous of the Eurasian continent appears to be the most studied by paleomagnetism; see, for instance the studies on Tibet (e.g. Achache et al. 1984), on the NCB (e.g. Ma et al. 1993), on Amuria (e.g. Halim et al. 1998a), on the SCB (e.g. Enkin et al. 1991), on Indochina (e.g. Huang & Opdyke 1993), on the Junggar basin (e.g. Chen et al. 1991) and on Tarim (e.g. Li et al. 1988). These earlier palaeomagnetic studies on Eurasian blocks show that all of the Tibetan and Central Asian blocks have been subjected to palaeomagnetic investigations with the exception of one; the Qaidam block. A preliminary study by Frost et al. (1995) and a subsequent one by Halim et al. (1998b) focused on Cretaceous formations in the Xining–Lanzhou basin on the southern border of the Qilian Shan. It was doubtful, however, to what extent this area would represent the entire Qaidam block. The work of Halim et al. (1998b) suggests that the Xining–Lanzhou basin during Cretaceous times is likely to have belonged to a Qaidam–Kunlun–Tarim landmass of which it formed the northeastern edge. This reinforces the idea that no palaeomagnetic data exist from the Qaidam sensu stricto. While a few studies proposing a Cretaceous configuration of Asia are available, no such relatively large-scale reconstructions based on data from all of the Asian blocks are available for Jurassic times. Given that some palaeomagnetic Jurassic data are available from the NCB, the SCB and to a lesser extent from Tarim, we decided to cover one more basin and to carry out, during the autumn of 1996, a field trip to northern Tibet. During this fieldwork, we sampled Upper Jurassic rocks in the neighbouring area of Huatugou town (38.46°N, 90.75°E), south of the Altyn Tagh fault, in the Qaidam basin.
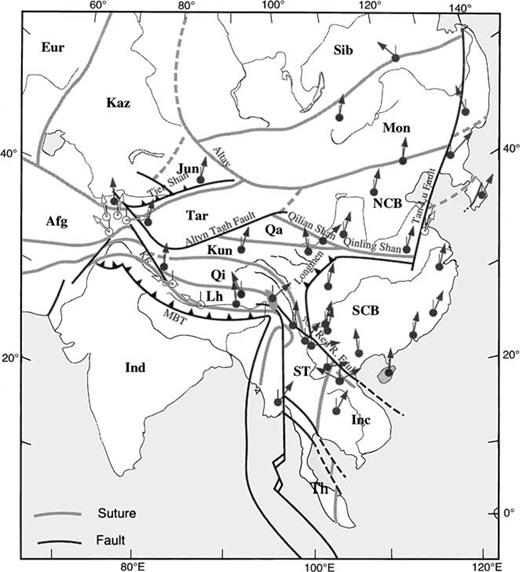
Simplified map of southeast Asia showing the main sutures and faults (KF, Karakorum fault; MBT, Main Boundary Thrust). The major blocks are: Afg, Afganistan; Eur, Eurasia; Inc, Indochina; Ind, India; Jun, Junggar; Kaz, Kazakhtan; Kun, Kunlun; Mon, Mongolia; NCB, North China Block; Qa, Qaidam; Qi, Qiangtang; Sib, Siberia; SCB, South China Block; ST, Shan Tai; and Tar, Tarim. Also indicated are the main locations where Cretaceous palaeomagnetic data are available. Declination is indicated by arrows (a thin line indicates geographic north, it is vertical because of the projection selected). Open arrows are for areas suspected of major local scale deformation or unreliable palaeomagnetic data. Solid arrows are thought to be representative of the individual blocks at a larger scale.
2 Palaeomagnetic Sampling and Laboratory Techniques
Standard cores were drilled in situ by a gasoline-powered drill around Huatugou town (Fig. 2) at a place located at (38.46°N, 90.75°E). Nine sites were sampled in the Jurassic red and soft Hongshuigou formation that consists of an intercalation of brown-red and green sandy mudstones. Each site represents a sedimentary thickness of 5–10 m. The Upper Jurassic age has been essentially assigned on the basis of ostracods (BGMRQP 1982). Although this Upper Jurassic formation seems to be conformably overlain by the Lower Cretaceous Quanyagou formation and to conformably overlie the Middle Jurassic Shimengou formation, sedimentary gaps exist between all of them. The variable bedding of the sampled strata present the possibility for a fold test. Both magnetic and solar azimuths have been measured in the field; the difference between them is negligible.
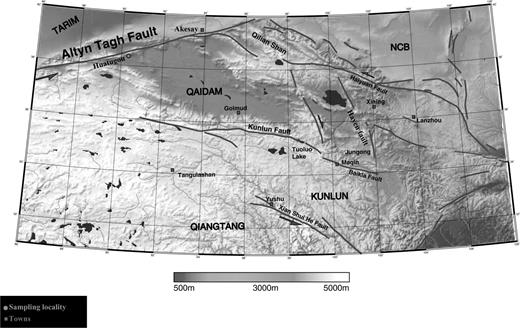
Simplified topographic map with the major faults. Squares indicate town locations, the circle is the palaeomagnetic sampling locality, Huatugou.
The standard cores were cut in the laboratory into 2.2 cm long specimens. Specimens have been thermally demagnetized in the palaeomagnetic laboratory of the Institut de Physique du Globe de Paris/Université Paris 7. Demagnetization was carried out in a magnetically shielded room using μ-metal-protected ovens and 15–20 heating steps, up to 690 °C. The natural remanent magnetization (NRM) was measured with a three-axis 2G DC cryogenic magnetometer. Isothermal remanent magnetization (IRM) acquisition was carried out on a few selected samples to help determining the magnetic carriers. Demagnetization results were plotted in orthogonal vector diagrams (Zijderveld 1967) and equal-area projections. Palaeomagnetic directions were determined using the principal-component analysis (Kirschvink 1980) and site mean directions were calculated using Fisher (1953) statistics.
3 Palaeomagnetic Analysis
A representative IRM acquisition is presented in Fig. 3(a). The IRM contribution can be divided into two parts: a first part with a steep slope below 0.1 T, and a second one with a relatively weak slope in fields higher than 0.1 T. No saturation is observed at 1.2 T. We interpret these observations as indicators of two principal magnetic carriers. The first one with a low saturation field that probably concerns magnetite in low fraction, and a second one with high saturation field that seems to be dominant in the samples, will be identified by analysing the demagnetization paths.
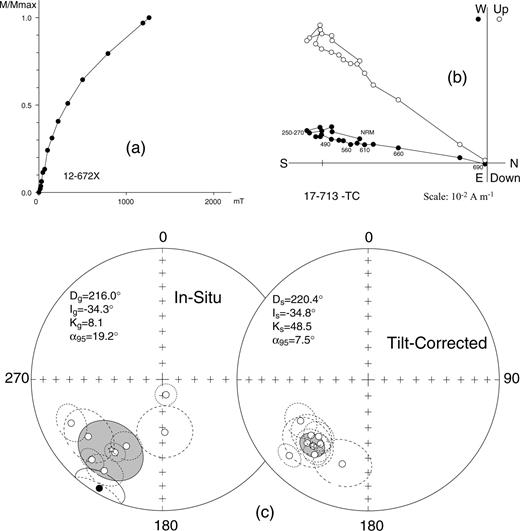
Results of magnetic mineralogy experiments and thermal demagnetization for the Upper Jurassic samples of Huatugou locality. (a) Isothermal remanent magnetization (IRM) acquisition curve. (b) Typical orthogonal vector plots (Zijderveld 1967), in tilt-corrected coordinates. Closed (open) symbols: projection on to the horizontal (vertical) plane. Temperatures are indicated in °C. (c) Equal-area projection of the HTC site means with their α95 cones of confidence before and after bedding correction. The star represents the overall mean direction.
A typical thermal demagnetization path is shown in Fig. 3(b), from which two magnetization components can easily be isolated. A low-temperature component (LTC) is resolved between the NRM 100 and 250 °C steps, and a higher-temperature component (HTC) is isolated between 490 and 680–690 °C, which clearly decays towards the origin during the final steps of thermal cleaning. The 680–690 °C unblocking temperature of the HTC shows that the second magnetic carrier is haematite, which is in agreement with the high saturation field pointed out by the IRM acquisition experiments. The LTC has been resolved in sevem out of nine sites. Its overall mean direction at the site level is Dg= 6.2°, Ig= 60.1°(kg= 57.2, α95= 8.0) in in situ (before tilt-correction) coordinates. We performed a stepwise unfolding that displayed a maximum precision parameter at 6 ± 26 per cent unfolding (Watson & Enkin 1993) demonstrating a post-folding origin for the LTC. Moreover, when compared with either the Geocentric Axial Dipole (GAD) or the IGRF field directions, the LTC appears to be indistinguishable from both. Therefore, we interpret it as a viscous remagnetization of recent origin.
The HTC, isolated from almost all samples, shows, in tilt-corrected coordinates, a southward declination with upward inclination (Fig. 3b). Fig. 3(c) presents site mean directions both before and after tilt corrections, which show that the site mean directions clustering is much better after unfolding. Watson & Enkin's (1993) fold test displays a maximum precision parameter at 93.2 ± 16.1 per cent total unfolding. We thus interpreted the HTC as a primary pre-folding magnetization. Its overall mean direction in tilt-corrected coordinates is Ds= 220.4°, Is=−34.8°(ks= 48.5, α95= 7.5°) (Table 1). The corresponding palaeomagnetic pole lies at λp= 50.1°N, φp= 198.0°E, (dp/dm= 5.0°/8.6°) (Table 2), and the Late Jurassic palaeolatitude of the Huatugou region is 19.2°N (Table 2).
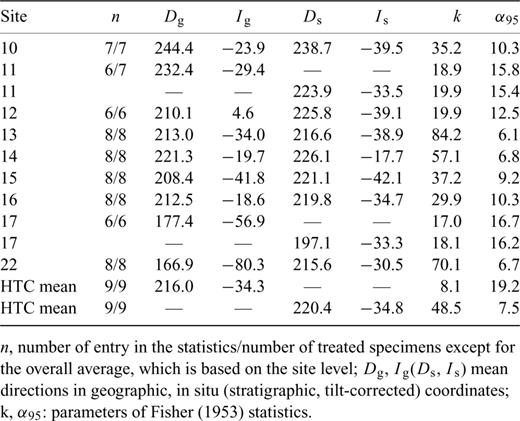
Site mean palaeomagnetic directions for the high-temperature components (HTC) from the Upper Jurassic samples.
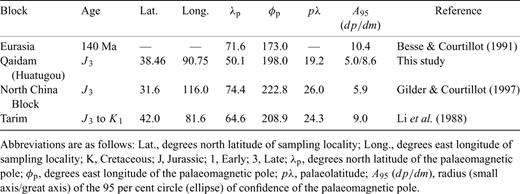
4 Discussion and Conclusion
The primary origin of the magnetic remanence isolated from the Huatugou J3 red beds makes it possible to discuss the tectonic implication of this result with other coeval ones such as Tarim and NCB and the reference Eurasian apparent polar wander path (APWP) (Besse & Courtillot 1991).
Qaidam versus Eurasia and Tarim
The Jurassic pole of Huatugou plotted in Fig. 4 is assumed in this discussion as a representative of the Qaidam block as a whole. This pole is represented together with the small circle passing through it and centred about the sampling locality (38.46°N, 90.75°E). Compared with the Upper Jurassic part of the Eurasian APWP, the pole of Huatugou is offset from the 140 Ma reference pole. When taking into account the location of the sampling locality, the angle between the two poles can be divided into latitude and rotation components. Translated into tectonic terms, the difference in latitude between the Upper Jurassic pole computed for Huatugou and the 140 Ma reference pole leads to a post-Late Jurassic NS displacement of 19.4°± 9.2° absorbed between Huatugou, or the Qaidam block if represented by this locality, and Eurasia to the north. This value is higher than the value of 7.0°± 4.6° obtained by Halim et al. (1998b) when studying Cretaceous formations further to the east in the Xining–Lanzhou basin. The two results do not contradict one another, and rather seem to indicate that the relative northward drift of at least the area situated north of the Kunlun fault to occupy its present position with respect to the North China Block and Eurasia started at the latest after Upper Jurassic times. However, this northward motion must have started before the Lower Cretaceous as the post-Lower Cretaceous convergence of Qaidam with respect to Eurasia is lower than its post-Upper Jurassic one. This relative NS motion would have been accompanied by a 16.8°± 14.3° clockwise rotation of the Qaidam with respect to Eurasia, which, even though different in magnitude, is in agreement with the post-Cretaceous clockwise rotation of the Xining–Lanzhou basin (31.5°± 5.5°) pointed by Halim (1998b). If we compare the Qaidam palaeopole with the J3−K1 palaeopole from Tarim (Li et al. 1988), the two poles lie within errors on a small circle centred about our sampling locality, suggesting strongly that no significant post-Late Jurassic north–south motion, which would represent a convergence between the Qaidam and Tarim blocks, has occurred: the relative north–south motion between the Qaidam and Tarim blocks is 4.6°± 8.2°, and hence statistically not significant. The accretion of the two blocks to form a single landmass seems thus to have occurred no later than the Late Jurassic. However, given the azimuth of the Altyn Tagh fault that forms the boundary between the two blocks, which is roughly N60, the left-lateral displacement on this fault would certainly be more than the 500 km of its NS convergence component. An exact value cannot be reliably given at present, paleomagnetism being unable to accurately detect longitudinal or oblique motions. In contrast, the two poles of Tarim and Qaidam suggest a relative rotation of the order of 16.0°± 11.2°. This rotation could point out that the Qaidam block, and most probably the Tibetan plateau as a whole, has suffered more intense deformation in its western than in its eastern part following the India collision, which is in agreement with the sinistrial strike-slip motion along the Altyn Tagh fault.
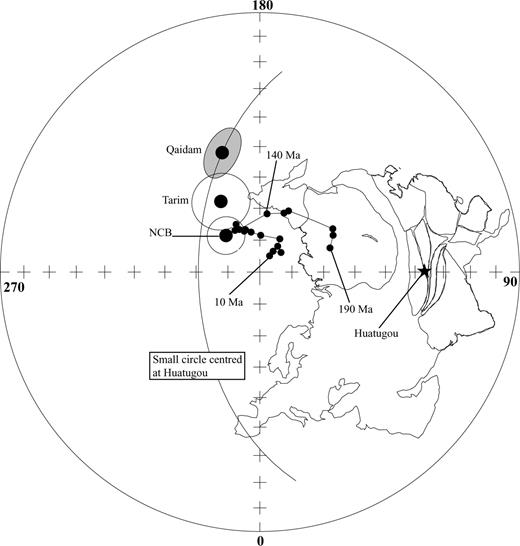
Equal-area projection of Jurassic poles from Huatugou locality (Qaidam basin) compared with those of the Tarim basin, the North China Block and to the reference APWP (small dots) for Eurasia of Besse & Courtillot (1991). References for pole locations are: Tarim (Li 1988), NCB (Gilder & Courtillot 1997).
Qaidam versus NCB
A comparison with only a synthetic APWP of Eurasia could be fairly criticized. Indeed, in two palaeomagnetic studies of Tertiary formations from Qiangtang and Kunlun (Cogné et al. 1999) and from Qaidam and Tarim (Halim 1998), a tectonic explanation of the shallow inclinations was proposed, in which Siberia would have behaved independently from Europe. According to these studies, the Eurasian APWP is not valid for Siberia during the Tertiary. Since it may also be uncertain that the Eurasian APWP is representative for Siberia for periods older than the Tertiary, we also compare our data with observed data from the NCB. The Upper Jurassic palaeomagnetic pole of the NCB (Gilder & Courtillot 1997) indicates that it was at a lower latitude during Jurassic times and underwent a northward displacement since then.
When we compare their respective poles, it appears that Qaidam has moved to the north and rotated clockwise with respect to the NCB since the Late Jurassic (Fig. 4). This northward displacement is estimated at 8.1°± 6.2°, and is accompanied by a clockwise rotation of 27.4°± 8.5°. Placing the different continental blocks at their respective palaeolatitudes puts both Qaidam and Tarim at comparable palaeolatitudes of ∼20°N, relatively to the south of the NCB, which would be around 26°N.
A sketch of the post-Jurassic block motions in the Asian continent
At this stage, we would like to highlight the importance of the difference between the values obtained for the northward displacement of the Qaidam with respect to the NCB, on the one hand, and with respect to Eurasia on the other hand. Indeed, while the former is of 8.1°± 6.2° the latter is of 19.4°± 9.2°, suggesting that 11.3°± 11.1° of crustal shortening needs to be accounted for somewhere in the different block boundaries that mark Eurasia. We suggest that the post-Jurassic northward motion of Qaidam is accommodated by two different major tectonic events. One is the Mongol–Okhotsk ocean closure that led to the collision between Siberia and the continental landmass(es) south of it and the other is the collision of India that would have made the Qaidam move northward with respect to the NCB.
Thus, it is possible to imagine a scenario with a potential northward displacement of the Qaidam–Tarim on the one hand and NCB-SCB on the other hand with continental convergence absorbed mainly in the Mongol–Okhotsk suture. This motion must have been taking place during the Lower Cretaceous. After the Lower Cretaceous, no more important continental convergence of the NCB-SCB plate could be absorbed to the north by mountain building that led to a roughly rigid area in the extreme east of the Asian continent consisting in SCB, NCB and Amuria. At this stage, the continental landmass to the west, which consists of at least Qaidam and Tarim, could still move to the north by approximately 400–700 km (Halim et al. 1998b). This continental convergence was probably absorbed in the Tien Shan, the Altay, the Sayan Tuva mountain range, and probably the Qilian Shan suture zone as well. In our opinion, this last post-Jurassic motion is not very old and would have happened during the Tertiary, the reason behind it being most probably the India collision.
In this study we have determined a reliable Jurassic palaeomagnetic pole from the northwest of the Qaidam basin. However, no other results from this basin are available in the literature to allow a comparison. Furthermore, the age of the pole we used for the Tarim could be questioned. We have presented these data with a plausible interpretation, wanting to avoid any overinterpretation. It is obvious that more data are needed from northern Tibet if the Jurassic situation of Eurasia were to be clarified. More Jurassic palaeomagnetic samples need to be collected from the Qaidam basin to verify the present results representing it as an entire block. Sampling of Jurassic rocks is also needed from the Kunlun block of which no palaeomagnetic data are available for this period of the Mesozoic. We recognize, however, the scarcity of outcropping Jurassic outcrops in the Kunlun. More Cretaceous data are also needed from the Qaidam to understand the relation of the Qaidam to the Gansu corridor. Only an increase in good quality data will allow a better view of the arrangement of the Eurasian continent during the middle part of the Mesozoic, and to propose a large-scale Jurassic configuration such as those already proposed for the Cretaceous (Chen et al. 1993; Halim et al. 1998b).
Acknowledgments
We thank Randy Enkin and an anonymous reviewer for reviewing this manuscript. Cor Langeris' help in improving the english was appreciated. N. Halim thanks Stuart Gilder for his contribution in the field. This is IPGP contribution no 1863.
References
Author notes
Now at: Department of Earth and Planetary Sciences, Kobe University, 657-8501, Nada, Kobe, Japan.