-
PDF
- Split View
-
Views
-
Cite
Cite
Lisa E Kursel, Kaan Goktepe, Ofer Rog, The structural role of Skp1 in the synaptonemal complex is conserved in nematodes, Genetics, Volume 228, Issue 3, November 2024, iyae153, https://doi.org/10.1093/genetics/iyae153
- Share Icon Share
Abstract
The synaptonemal complex (SC) is a meiotic interface that assembles between parental chromosomes and is essential for gamete formation. While the dimensions and ultrastructure of the SC are conserved across eukaryotes, its protein components are highly divergent. Recently, an unexpected component of the SC has been described in the nematode Caenorhabditis elegans: the Skp1-related proteins SKR-1/2, which are components of the Skp1, Cullin, F-box (SCF) ubiquitin ligase. Here, we find that the role of SKR-1 in the SC is conserved in Pristionchus pacificus. The P. pacificus Skp1 ortholog, Ppa-SKR-1, colocalizes with other SC proteins throughout meiotic prophase, where it occupies the middle of the SC. Like in C. elegans, the dimerization interface of Ppa-SKR-1 is required for its SC function. A dimerization mutant, ppa-skr-1F105E, fails to assemble SC and produces almost no progeny. Interestingly, the evolutionary trajectory of SKR-1 contrasts with other SC proteins. Unlike most SC proteins, SKR-1 is highly conserved in nematodes. Our results suggest that the structural role of SKR-1 in the SC has been conserved since the common ancestor of C. elegans and P. pacificus, and that rapidly evolving SC proteins have maintained the ability to interact with SKR-1 for at least 100 million years.
Introduction
The synaptonemal complex (SC) is a conserved interface that organizes chromosomes during meiosis. The SC aligns parental chromosomes end-to-end and regulates genetic exchanges between them, ultimately allowing for the proper segregation of chromosomes during the meiotic divisions. First identified by electron microscopy over 60 years ago, the SC is made up of 2 parallel axes (also called lateral or axial elements) separated by repeating striations that make up the central region of the SC (throughout, we refer to the central region of the SC simply as “the SC” (Page and Hawley 2004; Zickler and Kleckner 2023)).
Despite its essential role in reproduction, SC components have diverged beyond recognition in multiple eukaryotic clades (Hemmer and Blumenstiel 2016; Kursel et al. 2021). Indeed, new SC components are still being identified, and we likely still lack the full complement of SC components in most model organisms. Further complicating molecular studies, SC components exhibit near-complete co-dependence for assembly onto chromosomes, in worms and in other organisms (MacQueen et al. 2002; Colaiácovo et al. 2003; Smolikov et al. 2007; Page et al. 2008; Smolikov et al. 2009; Schramm et al. 2011; Collins et al. 2014).
Recently, two unexpected SC proteins were identified in Caenorhabditis elegans: the Skp1-related proteins SKR-1 and SKR-2 (Blundon et al. 2024). SKR-1/2 are members of the Skp1, Cullin, F-box (SCF) ubiquitin ligase complex, which plays a part in virtually all eukaryotic cellular processes including germline designation (DeRenzo et al. 2003), transcriptional regulation (Ouni et al. 2010), and hormone signaling in plants (Gray et al. 1999), to name a few (Willems et al. 2004). Within the SCF complex, Skp1 acts as an adapter by binding the N-terminus of Cul1 and the F-box motif in the F-box protein, linking the core scaffold to the substrate of the ubiquitin ligase machinery. In C. elegans, SKR-1/2 co-purify with all other SC proteins in vivo and in vitro, localize to the SC, and are required for its assembly. Notably, the SCF Cullin subunit CUL-1 does not localize to the SC and is not required for SC assembly. These data support the conclusion that SKR-1/2 are bona fide SC proteins in C. elegans (Blundon et al. 2024).
Here we address 2 outstanding questions regarding the role of SKR-1 in the SC: (1) Is the structural role of SKR-1 in the SC conserved in other nematodes? And (2) does SKR-1 share a similar evolutionary signature to other SC proteins? To address these questions, we identify Ppa-SKR-1, the single SKR-1 ortholog in the distantly related nematode Pristionchus pacificus. Our results indicate that Ppa-SKR-1 is a structural, essential component of the SC, suggesting that its role in the SC originated at least 100 million years ago, in the common ancestor of Pristionchus and Caenorhabditis nematodes. Interestingly, we find that the primary sequence of SKR-1 is conserved, setting it apart from other SC proteins and shedding light on the pressures that shape SC evolution.
Results
Identifying P. pacificus SKR-1
C. elegans and P. pacificus are a useful species pair for comparative studies. Like C. elegans, P. pacificus is a free-living, hermaphroditic nematode that has 6 pairs of chromosomes. Previous studies identified 2 SC proteins in P. pacificus: Ppa-SYP-1 (Kursel et al. 2021) and Ppa-SYP-4 (Rillo-Bohn et al. 2021). Given the recent identification of SKR-1/2 as a structural component of the SC in C. elegans (Blundon et al. 2024), we wondered whether SKR-1 plays a similar SC role in P. pacificus.
We used C. elegansSKR-1 as a BLASTp query against P. pacificus proteome. We identified a single strong hit which we refer to as Ppa-SKR-1. Ppa-SKR-1 clusters with C. elegans SKR-1/2 on a strongly supported branch to the exclusion of all other Skp1-related proteins in P. pacificus (Supplementary Fig. 1, Supplementary Data 2 and 3). While the C. elegans genome contains a recent duplication of SKR-1 called SKR-2 (Blundon et al. 2024), our phylogenetic analysis reveals that P. pacificus contains only 1 copy of SKR-1. We similarly queried 7 additional Pristionchus proteomes and found that most species have a single SKR-1 ortholog (Supplementary Fig. 2). We note that P. pacificus, like C. elegans, encodes many predicted Skp1-related proteins: 32 in P. pacificus and 21 in C. elegans (Supplementary Fig. 1; Nayak et al. 2002). While the expansion of the Skp1 family in nematodes complicates comprehensive tracing of their evolutionary history, SKR-1 orthologs appear to be the most conserved among Skp1-related proteins, and cluster together in a well-supported clade (Supplementary Fig. 2).
Ppa-SKR-1 localizes to the center of the SC
We used CRISPR/Cas9 to insert an OLLAS tag on the N-terminus of Ppa-SKR-1 and examined its localization (Fig. 1). OLLAS::Ppa-SKR-1 appears as threads on meiotic chromosomes from the time of SC assembly at meiotic entry, throughout pachytene (the stage when the SC is completely assembled), and to diplotene (the extended stage of SC disassembly; Fig. 1a). This pattern matches that of other SC proteins (Kursel et al. 2021; Rillo-Bohn et al. 2021). The axis component HOP-1 (Rillo-Bohn et al. 2021) localizes to meiotic chromosomes slightly before OLLAS::Ppa-SKR-1 as faint lines indicative of unpaired chromosomes (Fig. 1b). As OLLAS::Ppa-SKR-1 signal begins to overlap with HOP-1, the lines of HOP-1 are brighter, reflecting paired, synapsed chromosomes (Fig. 1b, see transition zone inset). During diplotene, OLLAS::Ppa-SKR-1 remains on the bright-staining regions of HOP-1 until the SC fully disassembles.
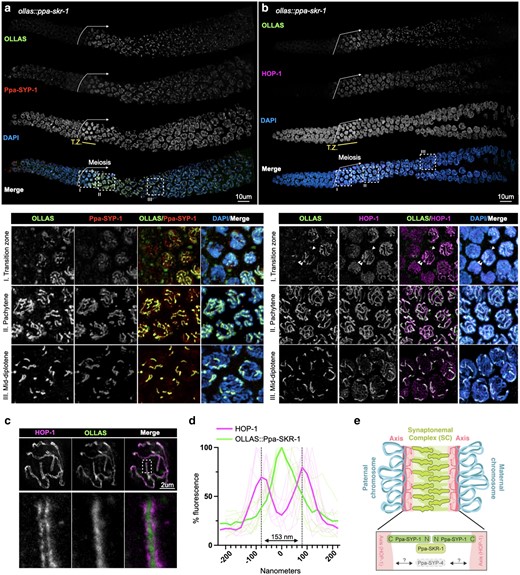
Ppa-SKR-1 localizes to the middle of the SC. a) Top, confocal image of whole gonads from ollas::Ppa-skr-1 worms stained with anti-OLLAS, anti-SYP-1, and DAPI. Bottom, zoom in on transition zone (I), pachytene (II) or mid-diplotene (III) nuclei. b) Confocal image as in a) except with HOP-1 staining. In the bottom panel of b), transition zone, the single arrowhead points to an unpaired chromosome (thin line of HOP-1) and the double arrowhead points to paired chromosomes (brighter HOP-1 staining). In both a) and b), the beginning of the meiotic gonad is indicated with a white arrow and the transition zone is labeled below the DAPI channel in yellow (T.Z.). c) Super-resolution STED image of a single pachytene nucleus from ollas::Ppa-skr-1 worms stained with anti-OLLAS and anti-HOP-1. Zoom-in panels show OLLAS::Ppa-SKR-1 between parallel HOP-1 tracks. d) Plot of line scans of pixel intensity for anti-HOP-1 and anti-OLLAS across parallel axes in ollas::Ppa-skr-1 worms. The average distance between parallel axes is 153 nm. e) Cartoon of the P. pacificus SC with the orientation and position of Ppa-SYP-1 and Ppa-SKR-1 relative to HOP-1 indicated in the bottom panel. The relative position of Ppa-SYP-4 is not known. Adapted from Kursel et al. (2023).
SC proteins occupy stereotypical positions in the ∼150 nm space separating the 2 parental chromosomes. Ppa-SYP-1, like its C. elegans counterpart, spans the width of the SC in a head-to-head manner (N-terminus in, C-terminus out) such that staining with a C-terminal epitope produces 2 parallel lines and N-terminal staining produces a single thread in the middle of the SC (Schild-Prüfert et al. 2011; Köhler et al. 2020; Kursel et al. 2021). Using STED super-resolution microscopy, we found that the axis protein HOP-1 formed parallel tracks that are 153 nm wide on average (Fig. 1, c and d) and that Ppa-SKR-1 localized to the central region of the SC, midway between the parallel HOP-1 tracks. These cytological data indicate that, like in C. elegans, Ppa-SKR-1 occupies the middle of the SC ladder, where the N-terminus of SYP-1 is located (Fig. 1e, Blundon et al. 2024).
The Ppa-SKR-1 dimerization interface is required for SC assembly
The essential functions of Skp1 make it challenging to study its role in the SC. C. elegans worms lacking both SKR-1 and -2 fail to hatch, reflecting the essential roles of SCF in embryogenesis (Nayak et al. 2002; Blundon et al. 2024). We therefore wished to generate a separation-of-function allele of ppa-skr-1.
Previous studies found that Skp1 dimerizes via a conserved hydrophobic interface that is not essential for F-box binding (Henzl et al. 1998; Kim et al. 2020). In C. elegans, mutations that disrupt SKR-1/2's ability to dimerize in vitro (skr-1F115E) cause a complete failure of SC assembly and prevent SKR-1/2 recruitment to an already formed SC. Importantly, these mutations do not abolish SCF activity, suggesting that SKR-1/2 dimerization is necessary specifically for SC function (Blundon et al. 2024).
We used structural homology to predict the dimerization interface in Ppa-SKR-1 (Fig. 2a; see alignment in Fig. 4). We found that a residue critical for dimerization in Dictyostelium Skp1, F97 (Kim et al. 2020), aligns closely with F105 in Ppa-SKR-1 (Fig. 2a). To test the function of the putative dimerization interface, we used CRISPR/Cas9 to generate ollas::ppa-skr-1F105E worms. Gratifyingly, we easily obtained ollas::Ppa-skr-1F105E homozygous animals. Out of 46 F2s singled from heterozygous ollas::Ppa-skr-1F105E F1 parents, 12 were homozygous wildtype, 22 were heterozygous, and 12 were homozygous for ollas::Ppa-skr-1F105E, matching expected Mendelian ratios. This suggests that the F105E muation does not disrupt SCF functions during development.
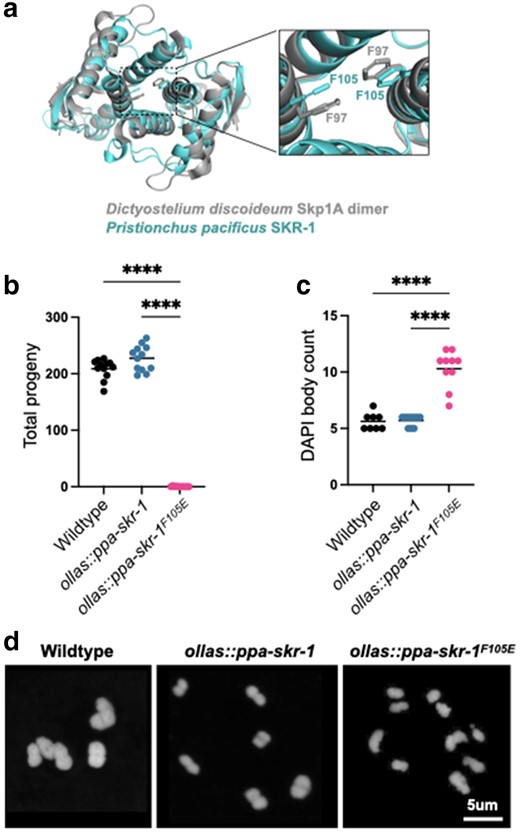
A conserved dimerization interface in SKR-1 is required for P. pacificus meiosis. a) Alignment of P. pacificus SKR-1 AlphaFold model (cyan) to Dictyostelium Skp1A dimer NMR structure (PDB structure 6V88, gray). Conserved phenylalanines required for dimerization are labeled in the zoom-in panel. Dot plot depicting total progeny b) and DAPI body count c) for wild-type P. pacificus, ollas::ppa-skr-1, and ollas::ppa-skr-1F105E. Asterisks reflect P-values from Tukey's multiple comparison test where **** indicates P < 0.0001. d) Representative images of DAPI-stained Meiosis I bivalents (DAPI bodies) from the indicated genotypes.
We used Alphafold-Multimer (Evans et al. 2022) to evaluate the dimerization ability of Ppa-SKR-1F105E. The highest scoring dimer model gave only a low confidence prediction (ipTM + TM = 0.30; compared to 0.55 for the wild type) and aligned poorly to the NMR structure of Dictyostelium Skp1 (Supplementary Fig. 3, Ppa-SKR-1F105E RMSD = 5.946 vs wild-type RMSD = 2.753). Based on these modeling results and on sequence homology, we refer to Ppa-SKR-1F105E as the “dimerization mutant”.
We found that Ppa-SKR-1 dimerization is necessary for reproduction. Total progeny produced by ollas::Ppa-skr-1 worms were comparable to that of the wild-type P. pacificus, indicating that the OLLAS insertion did not interfere with meiosis. In contrast, ollas::Ppa-skr-1F105E worms mimicked other SC null mutants and had very few progeny (Fig. 2b). Notably, several homozygous hermaphrodites produced 1 to 2 progeny, further indicating that OLLAS::Ppa-SKR-1F105E can carry out the non-meiotic functions of Skp1 proteins.
To examine meiotic dysfunction in more detail, we monitored successful formation of crossovers. Chromosomes that form a crossover are joined at metaphase of Meiosis I as “bivalents”. Since P. pacificus has 6 chromosome pairs, successful generation of a crossover on each pair yields 6 DAPI-staining bodies. We found no significant difference in DAPI body counts between wild-type and ollas::Ppa-skr-1 worms. They averaged 5.6 and 5.7 DAPI bodies, respectively (Fig. 2c). However, ollas::Ppa-skr-1F105E worms had a significantly elevated DAPI body count (mean = 10.5) suggesting that failure in crossover formation underlies the reduced progeny count in ollas::Ppa-skr-1F105E worms (Fig. 2d).
Cytological examination established that Ppa-skr-1F105E worms lack an SC. Ppa-SYP-1 antibody staining was absent (Fig. 3a). HOP-1 appeared as thin tracks throughout the gonad in Ppa-skr-1F105E worms, indicative of chromosomes that were unable to assemble an SC (Fig. 3b). Like other SC null mutants, meiotic nuclei in Ppa-skr-1F105E worms spent an extended duration in the transition zone (Fig. 3c)—the region of the gonad where the SC assembles, marked by crescent-shaped nuclei (Fig. 3, compare to Fig. 1, a and b).
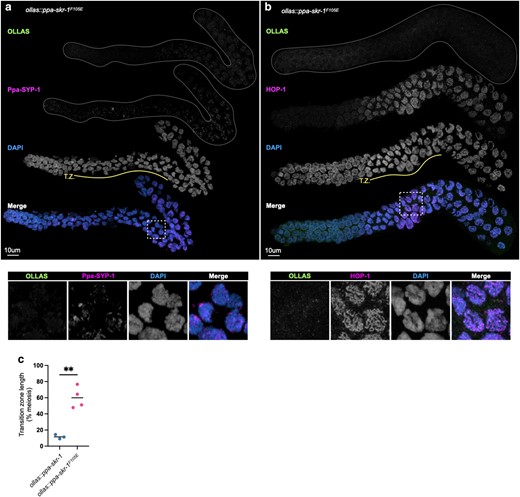
Ppa-SKR-1F105E fails to assemble the SC. a) and b), Confocal images of whole gonads from ollas::ppa-skr-1F105E stained with anti-OLLAS, anti-Ppa-SYP-1, a), or anti-HOP-1, b), and DAPI. The transition zone is labeled below the DAPI channel in yellow (T.Z.). Lower panels in a) and b) show zoom-in on regions indicated by white, dashed boxes. c) Dot plot showing transition zone length as percent of meiosis. Asterisks reflect the P-value from an unpaired t-test where ** indicates P < 0.01.
When the SC cannot load onto chromosomes, SC material forms large aggregates called polycomplexes (Hughes and Hawley 2020). However, polycomplexes are not present in null mutants in SC components in C. elegans and other species, suggesting all SC components are required for each other's stability. Notably, polycomplexes are absent in ollas::ppa-skr-1F105E worms (Fig. 3a) and in C. elegansskr-1F115E worms (Blundon et al. 2024), suggesting other SC component are not able to assemble in the dimerization mutant. Importantly, OLLAS::Ppa-SKR-1F105E itself is not destabilized since it has a similar nuclear and cytoplasmic localization pattern to OLLAS::Ppa-SKR-1 in somatic gut cells (Supplementary Fig. 4).
Our data indicate that ppa-skr-1F105E behaves like other known SC null mutants. It has a reduced brood size, SC assembly failure, an extended transition zone, and elevated DAPI body counts, much like C. elegansskr-1F115E; skr-2(kim66), syp-1, syp-2, syp-3, syp-4 or syp-5/6 mutants, or ppa-syp-1 or ppa-syp-4 mutants in P. pacificus (MacQueen et al. 2002; Colaiácovo et al. 2003; Smolikov et al. 2007; Smolikov et al. 2009; Blundon et al. 2024). Taken together with Ppa-SKR-1 localization, we conclude that Ppa-SKR-1 is a structural component of the P. pacificus SC.
Unlike other SC components, SKR-1 sequence is conserved in nematodes
We previously found that SC proteins in nematodes, Drosophila and mammals have a distinct evolutionary signature: diverged protein sequence but conserved length and position of coiled-coil domains and conserved overall protein length (Kursel et al. 2021). We hypothesized that this evolutionary signature is a consequence of SC's mode of assembly, which likely relies on weak multivalent interactions mediated by coiled-coils. Since the sequence requirements for coiled-coils are loose (typically defined as a heptad repeat where the first and fourth residues are hydrophobic and the fifth and seventh are charged or polar), selection to maintain coiled-coils could allow for significant sequence divergence. At the time of our analysis, SKR-1 had not been identified as an SC protein. Therefore, we wished to compare the evolutionary signature of SKR-1 to the other SC proteins.
Unlike the other SC proteins in Caenorhabditis and Pristionchus, the sequence of SKR-1 is conserved in both clades, ranking in the bottom 1 percentile for amino acid substitutions per site (Fig. 4a). Unsurprisingly, residues involved in CUL-1 binding, F-box protein binding, and the dimerization interface are highly conserved, even between C. elegans, P. pacificus, and H. sapiens (Fig. 4b). We also found that SKR-1 does not contain conserved coiled-coil domains (Fig. 4c, Supplementary Fig. 5a). Pristionchus SKR-1 does have a low-scoring predicted coiled-coil domain from amino acids 20–47 (Supplementary Fig. 5a). However, AlphaFold does not predict a coiled-coil formed by Ppa-SKR-1 and this coiled-coil signature is not conserved in Caenorhabditis (Supplementary Fig. 5a) or in Dictyostelium, where the corresponding residues are mostly disordered in the NMR structure (Kim et al. 2020). Together, this argues against the functional importance of coiled-coils in SKR-1 (Supplementary Fig. 5b). Lastly, the length of SKR-1 is conserved, like other SC proteins (Fig. 4d). Taken together, our analysis indicates that the evolutionary trajectory of SKR-1 is distinct from other SC proteins in Caenorhabditis and Pristionchus and suggests that its interaction with other SC proteins is mediated by domains other than coiled-coils.
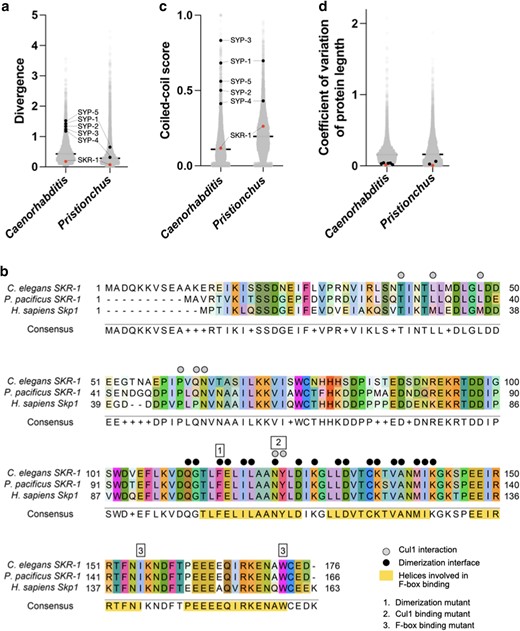
SKR-1 has an evolutionary signature distinct from other SC proteins. a) Dot plot showing protein sequence divergence for the Caenorhabditis and Pristionchus proteomes. SYP proteins and SKR-1 are indicated (black and pink, respectively). b) Alignment of Skp1 orthologs from C. elegans and P. pacificus, and H. sapiens with Cul1 interaction, dimerization, and F-box binding sites labeled (Zheng et al. 2002; Kim et al. 2020). Additionally, 3 mutants generated by Blundon et al. (2024) are indicated by numbered boxes. c) and d), dot plots showing coiled-coil conservation and coefficient of variation of protein length for the Caenorhabditis and Pristionchus proteomes (Kursel et al. 2021). SYP proteins and SKR-1 are indicated as in a).
Discussion
We found that SKR-1 is a structural member of the SC in P. pacificus. Ppa-SKR-1 dynamically localizes to meiotic chromosomes in a manner that is indistinguishable from that of other SC proteins. Like other SC proteins, Ppa-SKR-1 exhibits stereotypic localization relative to the axes (Fig. 1e). SKR-1 is essential for SC assembly and polycomplex formation in both C. elegans and P. pacificus. Such intimate co-dependence suggests the existence of underlying protein–protein interactions that provide specificity and stability. Taken together, our data suggest that the function of SKR-1 as a structural component of the SC has been conserved since the common ancestor of C. elegans and P. pacificus, at least 100 million years ago.
Our work on the conservation of an SC role for SKR-1 in P. pacificus raises the possibility that it extends to Skp1 proteins in other clades. Proteasome-mediated degradation regulates multiple key steps in meiosis (Ahuja et al. 2017; Rao et al. 2017; Guan et al. 2022), and the proteasome itself localizes to the SC in C. elegans and mice (Ahuja et al. 2017; Rao et al. 2017). Skp1 also localizes to the SC in mice (Guan et al. 2020), and in Arabidopsis (Wang et al. 2004). In both cases, its disruption leads to meiotic defects (Yang et al. 2006). However, the essential functions of the proteosome and of Skp1, and the far-ranging effects of their disruption, have made it difficult to parse their roles in protein degradation from any structural role in the SC.
The protein surfaces that mediate interactions between SC proteins must co-evolve to maintain compatibility. In this light, the high conservation of SKR-1 vs the high divergence of other SC components might seem surprising since proteins in complex often have homogenous evolutionary rates (Wong et al. 2008). However, proteins that function in multiple complexes can confound such analyses. SKR-1's role in the highly conserved SCF complex might overwhelm any signal of shared evolutionary trajectories with other SC proteins. In addition, the SC is a condensate (Rog et al. 2017), and many condensates rely on weak, multivalent interactions (Shin and Brangwynne 2017). SC proteins might have multiple, redundant interaction interfaces with SKR-1, each too weak to pose a strong constraint on the primary sequence.
The recent duplication of SKR-1 in the lineage leading to C. elegans (Blundon et al. 2024) could suggest that gene duplication has allowed Skp1 proteins to adopt a novel function—a structural component of the SC. However, our findings suggest that a single SKR-1 protein likely performed both functions in the common ancestor of C. elegans and P. pacificus. An ancestral dual-function protein suggests that SKR-1 has been subjected to evolutionary pressures to maintain both functions for at least 100 million years. Interestingly, SKR-1's dual roles in SCF and the SC entail that mutations in skr-1 might have pleiotropic effects in development (SCF) vs reproduction (SC). If so, C. elegans may represent a lineage where such intralocus conflict is resolving by gene duplication and specialization (Castellanos et al. 2024). In this scenario, the structural and functional requirements of the SC vs the SCF complex could be divided between SKR-1 and SKR-2, allowing them to eventually differentiate into an SC-dedicated protein and an SCF-dedicated one. Such specialization has likely taken place throughout the broader Skp1-related gene family, which has massively expanded in nematodes (Nayak et al. 2002). Intralocus conflict and related processes provide a leading framework in the evolution of aging (Adler and Bonduriansky 2014), suggesting that the evolutionary trajectory of SKR-1 in nematodes could shed light on the evolution of aging more broadly.
Materials and methods
CRISPR/Cas9 genome editing and immunofluorescence have been described previously (Kursel et al. 2021). See Supplementary methods and Supplementary Table 1 for more details.
Worm maintenance
P. pacificus strain PS312 served as a wild-type control. All strains were grown at 20°C on NGM agar with OP50 bacteria. Since ollas::ppa-skr-1F105E exhibited a strong Emb phenotype, we maintained it as a heterozygous line by singling animals and genotyping each generation.
Alignments and phylogenetic analysis
We used Clustal Omega for protein alignments and Geneious Tree Builder (neighbor-joining method, Geneious Prime version 2023.2.1) with 100× bootstrap resampling to generate the phylogenies. Protein sequences, alignments, and trees are available as Supplementary data (Supplementary Data 1–9).
Structural modeling
We used AlphaFold (Jumper et al. 2021), implemented in ColabFold (Mirdita et al. 2022), to model the structure of full-length Ppa-SKR-1. We used AlphaFold-Multimer (Evans et al. 2022) to model wild-type and Ppa-SKR-1F105E dimerization. We used Pymol ((Schrodinger 2015), version 2.5.7) to visualize and align structures and models.
Progeny counts
Progeny counts of the wild-type and ollas::ppa-skr-1 strains were performed as described in Kursel et al. (2021). For ollas::ppa-skr-1F105E worms, we singled 50 F1s from a heterozygous animal and identified the homozygous F1s by genotyping. We counted progeny from those animals confirmed to be homozygous mutants.
Divergence, coiled-coil conservation, and length conservation
The Caenorhabditis and Pristionchus proteome values (Fig. 4, a, c, and d) were published previously (Kursel et al. 2021). We calculated divergence values, coiled-coil conservation scores, and coefficient of variation of protein length for SKR-1 as we have done previously for SC proteins (Kursel et al. 2021) using SKR-1 orthologs from Caenorhabditis or Pristionchus.
Statistical analysis
We used an ordinary 1-way ANOVA with Tukey's multiple comparisons test to test for differences in total progeny and DAPI body counts between genotypes (Fig. 2, b and c). In Fig. 3a, we used an unpaired t-test to test for differences in transition zone length.
Data availability
Worm strains generated in this study are available by request. Sequence alignments and phylogenies are included as Supplementary data files. Proteome-wide analysis of divergence, coiled-coil scores, and protein length variation in Caenorhabditis and Pristionchus was published previously (Kursel et al. 2021). The authors affirm that all data necessary for confirming the conclusions of the article are present within the article, figures, and tables.
Supplemental material available at GENETICS online.
Acknowledgments
We thank the Rog Lab for discussions, Abby Dernburg for antibodies, and Yumi Kim for discussions and for sharing data prior to publication. Some strains used in this work were provided by the CGC, which is funded by NIH Office of Research Infrastructure Programs (P40 OD010440). We acknowledge the HSC Cell Imaging Core at the University of Utah for use of the STED microscope and The University of Utah Center for High Performance Computing for computational resources.
Funding
KG was supported by the Undergraduate Research Opportunity Program at the University of Utah and by the Biology Research Scholar Award from the School of Biological Sciences. This work was supported by grants R35GM128804 from the National Institute of General Medical Sciences and 2219605 from the National Science Foundation.
Literature cited
Author notes
Conflicts of interest The author(s) declare no conflicts of interest.