-
PDF
- Split View
-
Views
-
Cite
Cite
David M Brinkley, Karen C Smith, Emma C Fink, Woohyun Kwen, Nina H Yoo, Zachary West, Nora L Sullivan, Alex S Farthing, Valerie A Hale, Caroline Goutte, Notch signaling without the APH-2/nicastrin subunit of gamma secretase in Caenorhabditis elegans germline stem cells, Genetics, Volume 227, Issue 3, July 2024, iyae076, https://doi.org/10.1093/genetics/iyae076
- Share Icon Share
Abstract
The final step in Notch signaling activation is the transmembrane cleavage of Notch receptor by γ secretase. Thus far, genetic and biochemical evidence indicates that four subunits are essential for γ secretase activity in vivo: presenilin (the catalytic core), APH-1, PEN-2, and APH-2/nicastrin. Although some γ secretase activity has been detected in APH-2/nicastrin-deficient mammalian cell lines, the lack of biological relevance for this activity has left the quaternary γ secretase model unchallenged. Here, we provide the first example of in vivo Notch signal transduction without APH-2/nicastrin. The surprising dispensability of APH-2/nicastrin is observed in Caenorhabditis elegans germline stem cells (GSCs) and contrasts with its essential role in previously described C. elegans Notch signaling events. Depletion of GLP-1/Notch, presenilin, APH-1, or PEN-2 causes a striking loss of GSCs. In contrast, aph-2/nicastrin mutants maintain GSCs and exhibit robust and localized expression of the downstream Notch target sygl-1. Interestingly, APH-2/nicastrin is normally expressed in GSCs and becomes essential under conditions of compromised Notch function. Further insight is provided by reconstituting the C. elegans γ secretase complex in yeast, where we find that APH-2/nicastrin increases but is not essential for γ secretase activity. Together, our results are most consistent with a revised model of γ secretase in which the APH-2/nicastrin subunit has a modulatory, rather than obligatory role. We propose that a trimeric presenilin-APH-1-PEN-2 γ secretase complex can provide a low level of γ secretase activity, and that cellular context determines whether or not APH-2/nicastrin is essential for effective Notch signal transduction.
Introduction
Notch signaling is a direct cell-to-cell signaling mechanism that is used repeatedly by multicellular animals to coordinate cell identity and behavior among neighboring cells. Aberrant signaling results in severe developmental defects and a range of human diseases (Siebel and Lendahl 2017). Notch signal transduction relies on regulated proteolysis of the Notch receptor in response to its interaction with ligand from an adjacent cell. First, a ligand-induced conformational change of the Notch receptor allows access and cleavage by a metalloprotease of the ADAM family (A Disintegrin And Metalloprotease domain) to remove the receptor extracellular domain (S2 cleavage). Second, the remaining transmembrane domain becomes a substrate for S3 cleavage by the integral membrane protease γ secretase, releasing the Notch intracellular domain (NICD) to translocate to the nucleus where it associates with a DNA-binding protein of the CSL family, and elicits transcriptional regulation of target genes (CBF1/Su(H)/LAG-1) (reviewed in Kopan and Ilagan 2009). In addition to cleaving Notch receptor, γ secretase has additional type I membrane protein substrates, including E-cadherin, ErbB4, and the amyloid precursor protein (APP), whose proteolytic derivative is associated with the pathology of Alzheimer's disease (Guner and Lichtenthaler 2020).
Unlike other intramembrane cleaving proteases, γ secretase is a multipart complex, found to consist of four integral membrane subunits in a 1:1:1:1 stoichiometry: presenilin (the catalytic core), APH-1, PEN-2, and APH-2/nicastrin (Sato et al. 2007; and reviewed by Wolfe 2019). The current model states that all four subunits are required for Notch activation. However, this assertion has only been tested in a limited number of in vivo Notch signaling events for which the biological outcomes can be measured. Thus, a remaining open question is whether there exist cellular conditions in which any of the γ secretase subunits is dispensable. Identification of such cellular contexts would provide key insight into the precise role of the three noncatalytic subunits of γ secretase, and would reveal as yet unexplored context-dependent variations in the final step of Notch activation.
The intrinsic catalytic activity of presenilin has been demonstrated with purified protein reconstituted in liposomes (Ahn et al. 2010). The requirement for three additional subunits for in vivo γ secretase activity has puzzled the field since their discovery, particularly because the presenilin-related signal peptide peptidase functions without additional subunits (Weihofen et al. 2002). Cryo-EM structural analysis, subcellular localization studies, and cell-based enzyme activity measurements suggest that the three noncatalytic subunits facilitate γ secretase assembly, stability, and/or trafficking (reviewed by Wolfe 2019).
The role of the single-pass APH-2/nicastrin subunit has been especially controversial, with some studies suggesting a direct involvement in enzyme activity (Dries et al. 2009), and others suggesting only an indirect role in maturation and stabilization of the γ secretase complex (Zhang et al. 2005; Chavez-Gutierrez et al. 2008). Moreover, its large glycosylated extracellular domain has been specifically implicated in substrate selectivity, either by facilitating substrate docking (Shah et al. 2005) or alternatively, by sterically limiting substrate access to the γ secretase active site (Bolduc et al. 2016 ). A requirement for APH-2/nicastrin in Notch signaling has been supported by the description of aph-2/nicastrin mutant phenotypes that resemble Notch loss-of-function phenotypes in Caenorhabditis elegans (Goutte et al. 2000; Yu et al. 2000; Levitan et al. 2001), Drosophila (Chung and Struhl 2001; Hu et al. 2002; Lopez-Schier and St Johnston 2002), and mammals (Li et al. 2003). Nonetheless, two results hint that the APH-2/nicastrin subunit may be dispensable for γ secretase proteolytic activity. First, analysis of nicastrin-deficient mouse embryonic fibroblasts revealed some γ secretase cleavage of introduced APP or Notch substrates (Zhao et al. 2010). Second, mutant derivatives of the human presenilin gene PS1 were shown to support nicastrin-independent activity in a γ secretase reconstitution experiment in Saccharomyces cerevisiae (Futai et al. 2009). However, there remains no evidence that such APH-2/nicastrin-independent γ secretase activity is biologically consequential.
The requirement for γ secretase activity in Notch activation predicts that depletion of an essential γ secretase subunit will cause a Notch null phenotype. For the C. elegans embryo, this is indeed the case for each of the four γ secretase subunits (Li and Greenwald 1997; Westlund et al. 1999; Goutte et al. 2000; Yu et al. 2000; Francis et al. 2002; Goutte et al. 2002). However, similar evidence for postembryonic Notch signaling events remains incomplete, particularly for germline Notch signaling, which is complicated by the participation of both maternal and zygotic gene products. Here, we aimed to uncover previously elusive evidence for APH-2/nicastrin involvement in germline Notch signaling. We use new deletion alleles, presenilin-sensitized backgrounds, and RNAi, and we separately test the larval and adult phases of germline Notch signaling. Surprisingly, our analyses reveal that unlike the other γ secretase subunits, APH-2/nicastrin is not essential for Notch signaling in germ cells. To better understand this novel APH-2/nicastrin-independent Notch signaling, we probe induction of the downstream target gene sygl-1, and we test aph-2 mutants with compromised GLP-1/Notch receptor. As an independent approach, we use a yeast-based reconstitution system to test the requirements of individual C. elegans γ secretase subunits and find that APH-2/nicastrin increases γ secretase activity but is not essential. Altogether, our results suggest that in some cellular contexts, a tripartite γ secretase complex lacking APH-2/nicastrin can exert sufficient Notch cleavage to elicit a Notch signaling response. These results expand our understanding of γ secretase, which is currently limited to a quaternary composition.
Background on germline Notch signaling in C. elegans
A critical role for GLP-1/Notch signaling in C. elegans is to establish and maintain a pool of germline stem cells (GSCs) in the distal portion of the gonad (Austin and Kimble 1987; and reviewed in Hubbard and Schedl 2019). The somatic distal tip cell (DTC) serves as a stem cell niche, presenting Notch ligand to the underlying germ cells. Under the influence of ligand, GSCs are prevented from entering meiosis and instead remain undifferentiated and proliferative. Germ cells that are positioned farther proximally in the gonad are no longer exposed to ligand and, therefore, begin differentiation, entering meiosis and progressing through gametogenesis. Two phases of germline Notch signaling can be examined: First, an “expansion phase” (early larval development) during which the gonad is small, and all germ cells experience GLP-1/Notch activation, thus proliferating to expand the pool of germ cell progenitors. Second, a “maintenance phase” (late larval development through adulthood), during which only the distal-most subset of germ cells stays in contact with the stem cell niche, thus remaining proliferative. Continued proliferation of the GSC pool replenishes the differentiating germ cells that move proximally and ultimately out of the gonad as gametogenesis and fertilization proceed. In adults, the distal-most region of the gonad contains a distinct progenitor zone (PZ) that contains proliferating germline stem and progenitor cells as well as germ cells in meiotic S phase. In contrast, the remainder of the gonad is filled with germ cells at various stages of meiosis and gametogenesis.
Defects in Notch signaling during the larval and adult phases of germline function yield distinct phenotypes: In glp-1/Notch null hermaphrodites, larval germline expansion fails to occur, and instead larval germ cell progenitors prematurely enter meiosis and differentiate into spermatids. With no further supply of germline progenitor cells, the resulting adults are sterile (Austin and Kimble 1987). Conditional glp-1/Notch alleles can allow early larval Notch signaling to establish a proliferative germ cell population, but within 10 h of glp-1/Notch inactivation by temperature shift, all germ cells enter meiosis, and the distinct PZ is eliminated, resulting in animals that cannot maintain fertility (Austin and Kimble 1987; Fox and Schedl 2015; Agarwal et al. 2018). Because maintenance of the PZ relies on continuous GLP-1/Notch activation, measurement of PZ size has been used as a sensitive biological read-out of Notch activation in the adult gonad (Berry et al. 1997; Pepper et al. 2003; Fox and Schedl 2015).
The involvement of γ secretase subunits in the germline has only been extensively characterized for the presenilin catalytic subunit. Larval germline expansion is mediated by either of two presenilin encoding genes, hop-1 or sel-12, and maternally supplied sel-12 is sufficient (Li and Greenwald 1997; Westlund et al. 1999). Adult germline maintenance is mediated by zygotically provided hop-1 presenilin (Agarwal et al. 2018). The lack of obvious germline phenotype (sterility) in homozygous aph-1, aph-2/nicastrin, or pen-2 animals (M + Z−, see below) has been attributed to maternally contributed gene product that act during early larval germline expansion (Francis et al. 2002; Goutte et al. 2002). Indeed, depletion of both maternal and zygotic aph-1 by RNAi treatment abrogates germline expansion and causes a highly penetrant sterile phenotype (Goutte et al. 2002). Here, we directly test for this phenotype using aph-2 RNAi, and also provide the first report of aph-1, aph-2, and pen-2 requirements for adult germline maintenance.
Materials and methods
C. elegans handling and genetic alleles
All strains were derived from the Bristol N2 strain and maintained according to standard procedures at 20°C unless otherwise noted (Brenner 1974). Deletion alleles aph-1(tm4743) and aph-2(tm6471) were obtained from the National BioResource Project of Japan, part of the International C. elegans Gene Knockout Consortium. Deletion alleles were outcrossed a minimum of five times before use. aph-1(tm473) has an internal 605-bp deletion (I: 9,232,557–9,233,161) predicted to cause a frameshift after codon 91 and a premature stop codon at position 108. aph-2(tm6471) has an internal 1,239-bp deletion (I: 10,341,431–10,342,669; Supplementary Fig. 1). Full genotypes of strains used in this work are provided in Supplementary Table 1. The glp-1(e2144) allele used here was previously sequence verified (Hale et al. 2012). All males analyzed in this study were derived from homozygous him-8(e1489) hermaphrodites. Population synchronization was carried out by hatch off on solid media, as previously described (Agarwal et al. 2018). The following times were used, unless otherwise noted: L4: 42–46 h post-hatch; Day 1 adults: 54–62 h post-hatch; Day 2 adults: 72–80 h post-hatch; Day 3 adults: 96–104 h post-hatch; and Day 5 adults: 144–152 h post-hatch. Specific genotypes were selected as L4 larvae and maintained at 20°C until the desired age.
Phenotypic analysis
Egg-laying defect (Egl) and protruding vulva phenotypes were scored on two successive days after the L4 stage. The maternal-effect embryonic lethal (Mel) phenotype was assessed by counting the percent of embryos that hatch from laid eggs or from eggs dissected out of gravid Egl animals. The arrested embryonic phenotype was assessed by Nomarski microscopy; the lack of hypodermal enclosure and the presence of a differentiated posterior pharynx without the anterior pharynx were used as diagnostic of defective early embryonic Notch signaling (Goutte et al. 2000; Hale et al. 2012). Germline sterility was scored by the failure to produce embryos (laid or present in the uterus); sterile animals appear dark (Supplementary Fig. 2), and devoid of oocytes, as further analyzed by Normarski microscopy at 400× magnification with a Zeiss Axioscope. Due to adult-onset sterility, hop-1 animals were examined for sterility on Day 1 of adulthood; all other genotypes were scored both on Day 1 and Day 2 of adulthood. Analysis of aph-1 and aph-2 effects in reduced presenilin backgrounds was conducted by analyzing hop-1(ar179) aph-x homozygous animals derived from heterozygous hop-1(ar179) aph-x/hop-1(ar179) unc-13(e51) hermaphrodites and by analyzing aph-x; sel-12(ok2078) homozygous animals derived from heterozygous aph-x/+; sel-12(ok2078) hermaphrodites so that both zygotic and maternal presenilin contributions were removed.
Gonad analysis and PZ measurements
Gonad dissections, fixation, and DAPI staining were carried as previously described (Agarwal et al. 2018). Only data collected under identical conditions are compared within the same plot. Slight variations exist between trials, such as the experimenter, the incubator, and the exact aging protocol. For example, independent trials shown in Fig. 1b were aged to 55 ± 1 h in the left set of experiments and 18 h post-mid L4 in the right set of experiments (both of these protocols yield Day 1 adults aged 54–62 h post-hatch). Whole worms and dissected gonads were analyzed under 400× magnification with a Zeiss Axioscope equipped with epifluorescence and photographed with a digital Spot Camera (Diagnostic Instruments). The size of the PZ was quantified as previously described (Hansen, Albert Hubbard, and Schedl 2004). A Student's 2-tailed t-test, assuming unequal variances, was used to compare measurements of mutant vs. wild-type gonads aged and processed in parallel.
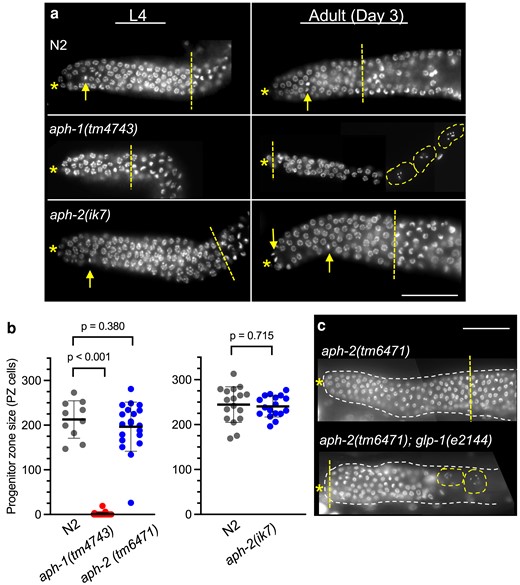
aph-2, but not aph-1, hermaphrodites maintain a GLP-1/Notch-dependent germline PZ in adulthood. a) Examples of dissected DAPI-stained gonads from staged hermaphrodites. The PZ contains undifferentiated germ cell nuclei between the distal end of the gonad (asterisk) and the distal side of the meiotic transition zone (straight dashed line) where leptotene nuclei are visible. Metaphase nuclei (arrow) are observed in the PZ. At the L4 stage (left), aph-2 and N2 have a robust PZ. In contrast, most aph-1 animals lack a PZ, and the remainder have a PZ that is reduced in size, as in the example shown here. Only N2 and aph-2 animals continue to maintain a PZ through adulthood (right). Adult aph-1 gonads lack a PZ and usually contain only oocytes (dashed outline) and spermatids; late-stage pachytene nuclei are sometimes observed, as in the example shown here. All mutant aph animals are M + Z−. Scale bar is 50 µm. b) The number of germ cells in the adult Day 1 PZ was measured in gonads dissected from N2 (left, n = 10; right, n = 17), aph-1(tm4743) (n = 29), aph-2(tm6471) (n = 20) or aph-2(ik7) (n = 17) (left and right experimental conditions differ slightly; see Materials and methods); mean (horizontal line) and SD (vertical line) are indicated. P-values are calculated from A Student's 2-tailed t-test, assuming unequal variances. c) PZ maintenance in aph-2-deficient adult gonads requires glp-1/Notch function. aph-2(tm6471) M + Z− hermaphrodites with glp-1(+) (top) or glp-1(e2144) (bottom) were shifted to restrictive temperature (25°C) after larval germline establishment (L4), and analyzed 48 h later; aph-2(tm6471); glp-1(+) animals maintain undifferentiated germ progenitor/stem cells, while aph-2(tm6471); glp-1(e2144) animals do not, and instead, the gonad contains germ cells in varying stages of meiotic development. A single distal gonad is shown (dashed outline) within the animal; labeling is as in (a).
Temperature-shifted animals
Strains carrying temperature-sensitive glp-1(e2144): For the restrictive temperature analysis (Fig. 1c), worms were synchronized and grown at 15°C, then shifted to 25°C in late L4, and maintained for 48 h at 25°C before fixation. For the semipermissive temperature analysis (Fig. 5a), worms were maintained at 20°C and analyzed 55–72 h post-hatch (10–27 h post-mid-L4).
Strains carrying temperature-sensitive glp-1(ar202): homozygotes were derived from glp-1(ar202)/+ heterozygous parents. Worms were maintained at 15°C and shifted to 25°C two days after the L4 stage. Worms were fixed and analyzed by whole-mount DAPI staining after 24–48 h at 25°C. These procedures were designed to minimize the effects of glp-1(ar202) on larval germline expansion, including the proximal tumor phenotype (Pepper et al. 2003).
RNAi treatments
Generation of dsRNA for RNAi
DNA templates for T7 polymerase transcription were generated by PCR amplification (OneTaq New England Biolabs) of aph-1 or aph-2 cDNA sequences with primers containing T7 RNA polymerase promoters as follows (primer sequences are listed in Supplementary Table 2): CEG-98F and CEG-99R (2.2-kb aph-2), CEG-98F and APH-2MR (0.96-kb carboxyl half of aph-2), CEG-99R and APH-2MF (1.26-kb amino half of aph-2), and CEG-78F and CEG-79R (1.0-kb aph-1). 200–800 ng of cleaned amplicon was transcribed using HiScribe T7 High Yield RNA Synthesis Kit (New England Biolabs) and cleaned with Ambion TURBO DNA-free Kit. RNA quality was verified by gel electrophoresis: The two smaller aph-2 RNA products were generated in order to match the size of the aph-1 dsRNA product; all three aph-2 dsRNA products were tested separately in pilot assays, and no differences were observed in their effects. All aph-2 RNAi experiments described here used either the larger aph-2 dsRNA product alone or an equal mixture of the two shorter aph-2 dsRNA products. dsRNA was used at 0.3–0.5 µg/µl for injections and 0.8–1.6 µg/µl for soaking.
RNAi soaking
dsRNA soaking was based on standard methods (Maeda et al. 2001). Briefly, ten 50-h-old N2 hermaphrodites (late L4) were soaked in 10 µl of soaking buffer with dsRNA for 18 h at 20°C. Worms were then transferred to fresh seeded plates every 3 h for a total of 15 h. The number of laid eggs (F1) on each plate was counted, and 17–24 h later, the number of unhatched eggs was counted. Viable L3 progeny were moved to individual plates and then scored for sterility (no progeny), fecundity (<10 embryos was the criteria for “low fecundity”), and Mel (presence of arrested F2 embryos). The number of F1 progeny that arrested as early larvae was calculated by subtracting the number of live progeny and unhatched eggs from the number of eggs laid. A sampling of dead embryos (≥30) was analyzed by Nomarski microscopy (see below). Worms that appeared sterile were fixed and DAPI stained to visualize germ cells; the absence of undifferentiated germ cells along with the presence of sperm was used as an indicator of glp-1-like sterility (Austin and Kimble 1987). For each experiment, treatments of aph-1, aph-2, and a no-RNA control were carried out in parallel. A separate set of RNAi soaking experiments used heterozygous aph-2(tm6471)/unc-13(e1091) lin-11(n566) hermaphrodites. In addition to scoring for dead eggs, and fertile vs. sterile live progeny, in this experiment, the progeny were also characterized as aph-2(+) homozygotes according to the Unc phenotype and aph-2(−) homozygotes according to the Pvl phenotype and/or the Egl Mel phenotype. In general, dsRNA soaking resulted in more penetrant results than did dsRNA injection.
RNAi injections
Young adult hermaphrodites were injected once in the posterior half of the body and transferred to fresh plates every 10–20 h following injections. Progeny were analyzed only from injected animals that yielded more than 40% dead embryos by 27 h post-injection and were analyzed as described above for RNAi soaking. A minimum of 200 live progeny from 4–10 injected animals were analyzed for each experiment. The injected animals were N2 or aph-2(ik7)/dpy-5(e61) unc-13(e51) heterozygotes.
Construction of aph-2(ik7) deletion allele by CRISPR-Cas9 genome editing
The dpy-10 co-CRISPR technique (Arribere et al. 2014) was used as previously described (Paix et al. 2015). sgRNA sequences were chosen so as to maximize the size of the aph-2 deletion and avoid interfering with adjacent genes ears-1 and coa-6 (Supplementary Fig. 1). Primers AFsg2 and AFsg3 (Supplementary Table 2) were used to introduce sgRNA sequences adjacent to the tracrRNA sequences of pDD162 [a gift from Bob Goldstein, Addgene # 47549 (Dickinson et al. 2013)] using Q5 site-directed Mutagenesis (New England Biolabs E0554S) generating plasmids pAFsg2 and pAFsg3, respectively. Young adult N2 hermaphrodites were injected with a 300-ng/µl mix of three plasmids [pAFsg2, pAFsg3, and AP568-2, a gift from Geraldine Seydoux, Addgene# 70047 (Paix et al. 2015)] and a ssODN repair template designed with 60 bases of homology flanking the aph-2 deletion (AFhrt1) as well as a ssODN repair template for dpy-10 [dpy10(cn64)-rt], according to previous concentration guidelines (Paix et al. 2014). F1 progeny generated within the first 40 h post-injection were singled from injected mothers that yielded at least 25% F1 progeny with the co-CRISPR Rol or Dpy phenotype. F2 progeny were then screened for expected aph-2 null phenotypes (Egl, Mel, and possible sterility, although the latter was not observed). Twenty-three out of 203 singled F1s yielded F2s with the Egl Mel phenotype (11%). Single worm lysis PCR and Sanger sequencing (GeneWiz) identified two large deletion alleles. Sequences were assembled and analyzed in Benchling. The aph-2(ik7) allele contains a 3,321-bp deletion (I: 10,342,135–10,345,455; see Supplementary Fig. 1) and an insertion of 23 bp from the repair template including an engineered BamH1 site (TGGCTAATAGGCTAATAGGATCC). aph-2(ik7) was outcrossed four times against N2 and then balanced as indicated in Supplementary Table 1.
Construction of epitope-tagged aph-2(ik10) by CRISPR-Cas9 genome editing
The same CRISPR/Cas-9 methods were used to introduce an OLLAS epitope at the carboxyl end of APH-2 between E716 and G717. The same sgRNA corresponding to exon 8 (primer AFsg3) was used as above. The ssODN repair template (ollas2aph2sg3) contains 60 bases of homology on each end, the OLLAS encoding sequence, and an introduced SacI site (Supplementary Table 2). F1 progeny were screened by single worm lysis PCR, followed by SacI digestion. Homozygous F2 progeny were verified by sequencing as above; the resulting allele, aph-2 (ik10[APH-2 Glu716::ollas]), was outcrossed against tmc18 four times.
Immunofluorescence
Genotype selection for distinguishing maternal vs zygotic APH-2::OLLAS
The recessive dpy-5(e61) allele was linked to aph-2(ik7) and placed opposite aph-2(ik10[aph-2::OLLAS]). The only source of APH-2::OLLAS protein in dpy-5 aph-2(ik7) progeny derived from these heterozygous mothers would be maternal product (recombinants were eliminated by removing Dpy animals that did not display the aph-2 Egl phenotype). As comparable animals that contain both maternal and zygotic APH-2::OLLAS, we used dpy-5 aph-2(ik10) progeny obtained from dpy-5(e61) aph-2(ik10)/aph-2(+) heterozygous mothers. Parallel analysis of dpy-5 aph-2(+) homozygous animals provided the negative control for APH-2::OLLAS staining (see Supplementary Table 1 for full genotypes). Desired genotypes were chosen at the L4 stage and allowed to age for 18–24 h before gonad dissection and immunofluorescence.
Genotype selection for OLLAS::SYGL-1 analysis
Homozygous aph-x sygl-1(q983) animals were identified by a 2-step process: first nonbalancer containing progeny of heterozygous parents (see Supplementary Table 1 for full genotype) were singled to individual plates at the L4 stage. After 24 h, aph-x homozygosity was verified by the Egl phenotype. For aph-1(tm4743) sygl-1(q983), this step was critical because the tmc27 balancer does not fully cover the aph-1–sygl-1 interval; 20% aph-1 sygl-1(q983)/aph-1(+) sygl-1(q983) recombinants were thus eliminated before immunofluorescence. For aph-2(ik7) sygl-1(q983), this step was not critical because the tmc27 balancer covers the aph-2–sygl-1 interval; less than 1% non-Egl recombinants were eliminated by this method.
Fixation, staining, and microscopy
Worms were harvested and washed three times with M9 and transferred in 0.2 mM levamisole to a poly-lysine-dubbed slide where they were cut to extrude the gonads. The M9 was replaced with 3% formaldehyde in 0.1 M potassium phosphate and incubated for 10–20 min at room temperature. After washing with PBS + 0.1% Tween, slides were incubated either in methanol for 5–10 min at −20°C (APH-2 visualization) or PBS + 0.1% Tween + 0.2% Triton X (SYGL-1 visualization), washed as above, and incubated in PBS + 0.1% Tween + 1% BSA for 90 min at room temperature. Slides were incubated at 15°C with anti-OLLAS antibody (Novus Biologicals) at 1:1,000 for 15 h (SYGL-1 visualization) or at 1:200 for 1 h (APH-2 visualization). After washing with PBS + 0.1% Tween, slides were incubated for 90 min with Goat anti-rat IgG Alexa Fluor-488 or Donkey anti-rat IgG Cy3 (1:200, Jackson ImmunoResearch). Slides were washed and mounted in VectaShield Mounting Media with DAPI or Prolong Diamond Antifade Mountant with DAPI (Invitrogen). SYGL-1 imaging was carried out on a Zeiss Axioscope using a 40×/1.3NA objective, photographed with a digital Spot Camera, and analyzed in Fiji/ImageJ (Schindelin et al. 2012). APH-2 imaging was carried out on a Zeiss LSM980 using a 40×/1.4NA objective.
Quantitative comparison of APH-2::OLLAS staining in three genotypes
For each gonad, DAPI staining was used to choose a top and bottom focal plane for imaging. APH-2::OLLAS intensity in the distal gonad was measured using Zeiss ZEN software: the polygon tool was used to define the distal-most 350µm2 ± 50 µm of the gonad, avoiding the DTC, which shows background staining. For each gonad, the mean pixel intensity of the top and bottom focal plane was averaged and values were compared among the three genotypes without background subtraction (Supplementary Fig. 4b). For visual analysis, background staining was removed by using the mean value of all negative control measurements as the black display setting (Supplementary Fig. 4a).
Quantitative analysis of OLLAS::SYGL-1 staining
Only gonads that were stained and photographed in parallel with identical conditions and camera settings were compared. Three different measurements were made using Fiji/ImageJ software (Schindelin et al. 2012).
Cells expressing SYGL-1
First, the boundary of SYGL-1 expression was defined by drawing a line to separate the most proximal SYGL-1 staining from background levels; for this purpose, anything above background was defined as SYGL-1 staining. The Fiji/Image J Cell Counter plug-in (ImageJ. http://imagej.nih.gov/ij/) was used on the corresponding Z stack of DAPI images to count all the germ cell nuclei distal to the defined boundary.
Highest SYGL-1-expressing cells
The above process was repeated with one difference: the boundary was drawn just proximal to the first drop-off in staining intensity (all gonads showed the highest intensity staining in the distal-most region, gradually decreasing in more posteriorly positioned cells).
SYGL-1 intensity in distal germ cells
For each gonad, the Z stack position with the brightest SYGL-1 staining was chosen and photographed below saturation (400 ms). The ImageJ lasso tool was used to define and measure mean pixel intensity for three sample cytoplasmic areas within the distal gonad (distal-most 5-cell diameter region). The lasso tool was also used to measure background intensity by sampling a region positioned just proximal to the boundary of SYGL-1 staining above the background. The mean pixel intensity for this control region was subtracted from an average of three distal intensity measurements to obtain a corrected mean intensity for the distal-most cells.
Yeast reconstitution
Reconstitution of C. elegans γ secretase was based on published methods (Edbauer et al. 2003). Yeast strain PJ69-4a, with genotype MATa trp1-901 leu2-3,112 ura3-52 his3-200 gal4Δ gal80Δ LYS2::GAL1-HIS3 GAL2-ADE2 met2:: GAL7-lacZ (James et al. 1996) was provided by Peter Pryciak, UMASS Chan Medical School. Bidirectional expression vectors, pBEVY-L and pBEVY-T, were gifts from Charles Miller [Addgene plasmid # 51226 and # 51232 (Miller et al. 1998)], and each contains two promoters: ADH1 and GPD with cloning sites. cDNAs for aph-1, aph-2, sel-12, hop-1, and pen-2 were amplified with primers listed in Supplementary Table 2 and cloned into pCR2.1 (Invitrogen). Primers were designed so as to introduce yeast translational initiation sequences and XmaI, SacI, XbaI, or Sbf1 restriction sites. XmaI-SacI or SbaI-Sbf1 restriction fragments from these plasmids were then cloned into pBEVY-L and pBEVY-T downstream of the ADH1 or GPD promoter. Constructed plasmids were verified by sequencing. pCL-1, which encodes GAL4 (Sikorski and Hieter 1989), was provided by Harold Steiner (Edbauer et al. 2003). Supplementary Table 3 contains a list of all yeast expression plasmids built for this work, with the indicated γ secretase subunit coding sequences.
The hybrid GLP-1-GAL4 substrate was designed to mimic the predicted γ secretase GLP-1 substrate after ectodomain shedding by S2 cleavage. The S2 cleavage site of Mus musculus Notch1 (mmNotch1) occurs between A1710 and V1711 (Hemming et al. 2008). We aligned the C. elegans GLP-1 sequence and used the corresponding A750 and V751 of GLP-1 as a likely S2 cleavage site. Following the substrate design of Edbauer et al. (2003) and Futai et al. (2009), we preceded the GLP-1 coding sequence with sequences encoding the signal sequence of the yeast Suc2 gene. Signal peptide cleavage of this chimeric protein is predicted to generate a truncated GLP-1-GAL4 protein that contains 15 residues before the GLP-1 transmembrane domain, which is fused to GAL4 after GLP-1 residue 799. pJK414 containing glp-1 cDNA was provided by Judith Kimble (Kodoyianni et al. 1992). The GLP-1-GAL4 substrate was generated by combining DNA fragments encoding the ADH1 promoter (amplified from pCL-1 with primers EF25 and EF26), yeast translational initiation sequence and the Suc2 signal sequence (introduced in nested primers EF27a and EF29), the GLP-1 transmembrane domain (amplified from pJK414 with primers EF27a and EF28), Gal4, and ADH1 terminator (both amplified from pCL1 with EF31 and EF32). The combined product was cloned into the URA3 integrating yeast shuttle vector pRS306 (Sikorski and Hieter 1989) provided by Peter Pryciak and integrated into the genomic URA3 locus of PJ69-4a. The resulting strain “yNYC2” contains the integrated pADH1-Suc2 signal-GLP-1(751-799)-GAL4 construct and URA3+. yNYC2 was transformed with different combinations of the plasmids listed in Supplementary Table 3 to generate the strains tested in Fig. 6 and Supplementary Fig. 6.
Yeast strain yNYC2 expressing C. elegans γ secretase components was tested for reconstituted γ secretase activity by measuring expression of GAL4-dependent reporters shown in Fig. 6a. For HIS3 and ADE2 expression, two independent transformants were patched onto synthetic defined (SD) Leu Trp dropout solid media (dropout powders from Clontech Laboratories, Inc.) and then replicated onto SD Leu Trp His Ade or SD Leu Trp His or Leu Trp dropout solid media. The most stringent selection was achieved by supplementing growth media with 25 mM 3-aminotriazole, which inhibits the HIS3 protein and raises the minimum amount of HIS3 gene expression necessary for growth on His dropout medium. For LacZ expression, liquid β-galactosidase assays were carried out according to standard procedures, as previously reported (Hale et al. 2012). The positive control used in all assays was the YNC2 strain transformed with the empty pBEVY-T vector and pCL1, which encodes soluble GAL4 that can activate reporter expression without the need for γ secretase activity. The negative control was the YNC2 strain transformed with empty pBEVY-T and pBEVY-L vector plasmids. A Student's 2-tailed t-test, assuming unequal variances, was used for statistical analysis.
Results
Generation and analysis of new aph-1 and aph-2 null mutations
The lack of true null alleles for aph-1 and aph-2 has limited the interpretation of previous phenotypic analyses. Here, we analyze three new deletion alleles: aph-1(tm4743) and aph-2(tm6471), generated by the National BioResource Project of Japan, and aph-2(ik7), which we engineered using CRISPR/Cas9 to delete most of the aph-2 coding region (see Materials and methods and Supplementary Fig. 1). The two aph-2 deletion alleles behaved similarly in our phenotypic analyses. Hermaphrodites that are homozygous for either aph-1(tm4743), aph-2(tm6471), or aph-2(ik7) display two fully penetrant Notch-like phenotypes consistent with prior point mutation analysis (Priess et al. 1987; Goutte et al. 2000; Levitan et al. 2001; Francis et al. 2002; Goutte et al. 2002). First, they display a fully penetrant egg-laying defect (Egl) resulting in the accumulation of embryos in the uterus (Table 1 and Supplementary Fig. 2). Associated with this defect is a partially penetrant protruding vulva (Pvl) phenotype (Table 1 and Supplementary Fig. 2) that is reminiscent of mutants with reduced lin-12/Notch or presenilin function (Sundaram and Greenwald 1993; Westlund et al. 1999; Levitan et al. 2001). Second, they display a fully penetrant maternal-effect embryonic lethality (Mel) in which their embryos fail to complete embryogenesis, arresting with the hallmarks of failed Notch signaling at the early stages of blastomere fate specification (Table 1). Due to the Mel phenotype, analysis of postembryonic phenotypes must be carried out in zygotic null animals (Z−) that are derived from heterozygous mothers, so that maternally contributed aph(+) gene product (M+) can drive successful embryogenesis. The resulting animals are designated here as M + Z− to remind the reader of the embryonic maternal contribution. Together, these phenotypes are consistent with the established roles of APH-1 and APH-2/nicastrin as essential components of Notch signaling in vulval/uterine precursor cells and in the early embryo, and were not further investigated in this study.
Notch-like phenotype: . | Egg-laying defect . | Protruding vulvaa . | Maternal-effect embryonic lethalityb . | glp-1/Notch-like germline sterilityc . | ||
---|---|---|---|---|---|---|
in wild-type background . | in hop-1(ar179) backgroundd . | in sel-12(ok2078) background . | ||||
N2 | 0% (50) | 0% (120) | 0% (>500) | 0% (100) | 0% (316) | 0% (152) |
aph-2(tm6471)e | 100% (94) | 53% (94) | 100% (>500) | 0% (345) | 0% (104) | 0% (187) |
aph-2(ik7)e | 100% (106) | 79% (106) | 100% (>500) | 0% (180) | 0% (106) | 0% (165) |
aph-1(tm4743)e | 100% (93) | 82% (93) | 100% (>500) | 0% (157) | 0% (122) | 100% (190) |
Notch-like phenotype: . | Egg-laying defect . | Protruding vulvaa . | Maternal-effect embryonic lethalityb . | glp-1/Notch-like germline sterilityc . | ||
---|---|---|---|---|---|---|
in wild-type background . | in hop-1(ar179) backgroundd . | in sel-12(ok2078) background . | ||||
N2 | 0% (50) | 0% (120) | 0% (>500) | 0% (100) | 0% (316) | 0% (152) |
aph-2(tm6471)e | 100% (94) | 53% (94) | 100% (>500) | 0% (345) | 0% (104) | 0% (187) |
aph-2(ik7)e | 100% (106) | 79% (106) | 100% (>500) | 0% (180) | 0% (106) | 0% (165) |
aph-1(tm4743)e | 100% (93) | 82% (93) | 100% (>500) | 0% (157) | 0% (122) | 100% (190) |
aVulval protrusions range in size and were broadly defined as any protrusion apparent on day 1 or day 2 of adulthood.
bArrested embryogenesis characterized by failed hypodermal enclosure and lack of anterior pharynx.
cSterility characterized by a gonad that lacks undifferentiated germ cells and instead contains only meiotic cells (spermatids).
dTo distinguish from the late-onset sterility that hop-1(ar179) causes on its own (Agarwal et al. 2018), only young adults are scored for sterility (see Materials and methods).
eHomozygous null adult derived from heterozygous parent (M + Z−).
Notch-like phenotype: . | Egg-laying defect . | Protruding vulvaa . | Maternal-effect embryonic lethalityb . | glp-1/Notch-like germline sterilityc . | ||
---|---|---|---|---|---|---|
in wild-type background . | in hop-1(ar179) backgroundd . | in sel-12(ok2078) background . | ||||
N2 | 0% (50) | 0% (120) | 0% (>500) | 0% (100) | 0% (316) | 0% (152) |
aph-2(tm6471)e | 100% (94) | 53% (94) | 100% (>500) | 0% (345) | 0% (104) | 0% (187) |
aph-2(ik7)e | 100% (106) | 79% (106) | 100% (>500) | 0% (180) | 0% (106) | 0% (165) |
aph-1(tm4743)e | 100% (93) | 82% (93) | 100% (>500) | 0% (157) | 0% (122) | 100% (190) |
Notch-like phenotype: . | Egg-laying defect . | Protruding vulvaa . | Maternal-effect embryonic lethalityb . | glp-1/Notch-like germline sterilityc . | ||
---|---|---|---|---|---|---|
in wild-type background . | in hop-1(ar179) backgroundd . | in sel-12(ok2078) background . | ||||
N2 | 0% (50) | 0% (120) | 0% (>500) | 0% (100) | 0% (316) | 0% (152) |
aph-2(tm6471)e | 100% (94) | 53% (94) | 100% (>500) | 0% (345) | 0% (104) | 0% (187) |
aph-2(ik7)e | 100% (106) | 79% (106) | 100% (>500) | 0% (180) | 0% (106) | 0% (165) |
aph-1(tm4743)e | 100% (93) | 82% (93) | 100% (>500) | 0% (157) | 0% (122) | 100% (190) |
aVulval protrusions range in size and were broadly defined as any protrusion apparent on day 1 or day 2 of adulthood.
bArrested embryogenesis characterized by failed hypodermal enclosure and lack of anterior pharynx.
cSterility characterized by a gonad that lacks undifferentiated germ cells and instead contains only meiotic cells (spermatids).
dTo distinguish from the late-onset sterility that hop-1(ar179) causes on its own (Agarwal et al. 2018), only young adults are scored for sterility (see Materials and methods).
eHomozygous null adult derived from heterozygous parent (M + Z−).
aph-2 null alleles do not cause glp-1/Notch-like sterility in presenilin-reduced backgrounds
A role for APH-1 or APH-2 in fertility cannot be ascertained in zygotic null (M + Z−) adults because of potential maternal rescue during the early larval germline proliferation that establishes fertility. However, depletion of sel-12, one of two partially redundant presenilins, causes a presenilin hypomorph that is fertile, but sensitized to zygotic gene contributions during larval germline proliferation. Thus, although aph-1 or pen-2 null animals (M + Z−) are fertile, a synthetic sterile phenotype is observed in aph-1; sel-12 or pen-2; sel-12 double mutants, similar to loss of glp-1/Notch, in which germ cells precociously enter meiosis during larval development (Francis et al. 2002). Curiously, aph-2; sel-12 double mutants have not been reported to cause germline sterility (Francis et al. 2002). One explanation for this difference could be that previously tested aph-2 mutations were nonnull alleles that did not fully remove gene function.
We tested the new deletion alleles of aph-1 and aph-2 in a sel-12(null) background and confirmed that zygotic aph-1 depletion causes sterility in a sel-12(null) background. In contrast, neither of the aph-2 null alleles has this effect (Table 1; Supplementary Fig. 2). We also tested for sterility in double mutant combinations with hop-1(null) but found that hop-1 depletion does not sensitize the worms to the presence or absence of zygotic aph-1 or zygotic aph-2, as both types of double mutants are fertile (Table 1; Supplementary Fig. 2). These analyses confirm that reduced presenilin reveals a critical requirement for the APH-1 subunit but fails to reveal such a requirement for the APH-2/nicastrin subunit, even when deletion alleles are tested.
In the course of these analyses, we noticed that aph-2 (M + Z−) hermaphrodites often appear more bloated with embryos than do aph-1 hermaphrodites, suggesting higher fecundity for aph-2 than aph-1 animals (Supplementary Fig. 2). Together, with the presenilin-sensitized results, these observations hint at unexpected differences between aph-1 and aph-2 function in the germline. The experiments described below were designed to directly compare the roles of aph-1 and aph-2 in GSC Notch signaling.
Adult aph-1 hermaphrodites lack GSCs, but adult aph-2 hermaphrodites successfully maintain a proliferating germline
We first investigated adult germline Notch signaling, which can be assessed in zygotic aph-1 and aph-2 mutants (M + Z−). Maintenance of an adult GSC pool is essential for fecundity and extended reproductive span (Austin and Kimble 1987; Agarwal et al. 2018), but these features could not be measured in aph-1 and aph-2 hermaphrodites because of their Egl phenotype. Instead, gonads were dissected out of aph-1 and aph-2 adult hermaphrodites and directly assessed for the presence and size of the distal germline PZ. aph-1(tm4743) hermaphrodites reveal a highly penetrant adult “loss of GSCs” phenotype, in which the PZ does not persist in adults, and instead, all germ cells are in meiotic stages of gametogenesis [90% of Day 1 adult gonads (n = 31), 84% of Day 2 adult gonads (n = 37), and 100% of Day 3 adult gonads (n = 20); Fig. 1a]. The loss of GSCs begins even before the onset of adulthood, as 39% of aph-1(tm4743) L4-staged gonads lack a PZ, and the remaining 61% have a PZ that is significantly reduced in cell number relative to those of wild-type gonads (Supplementary Fig. 3). These results suggest that maternally supplied aph-1 gene product is expended by the end of larval development, and in the absence of zygotic aph-1, germ cells switch from the stem cell fate to meiosis, as is observed upon inactivation of glp-1/Notch receptor function (Austin and Kimble 1987; Fox and Schedl 2015). The requirement for APH-1 in adult germline maintenance is consistent with the role of APH-1 as an obligatory subunit of γ secretase.
In striking contrast, the majority of gonads dissected from aph-2(tm6471) or aph-2(ik7) adult hermaphrodites display a robust PZ (Fig. 1a). Metaphase nuclei, indicating active cell division, are detectable, and the size of the aph-2 Day 1 adult PZ is indistinguishable from wild-type gonads analyzed in parallel (Fig. 1b). In aph-2 gonads, as in wild type, morphological signs of meiosis appear only in more proximal positions, as germ cells move away from the DTC (Fig. 1a). The contrasting effects of aph-1 and aph-2 adult germline phenotypes contradict the simple expectation that removal of any one subunit of γ secretase should cause the same phenotype.
We considered three alternative explanations for this surprising result: (1) APH-2/nicastrin is dispensable for adult germline Notch signaling; (2) APH-2/nicastrin is required for germline Notch signaling, but the animals analyzed in these experiments do not exhibit a phenotype because they have retained maternally supplied aph-2(+) product beyond larval development; or (3) the maintenance of undifferentiated proliferative germ cells in aph-2(null) adults is achieved through a mechanism other than Notch signaling. To address the third possibility, we tested whether the germ cell population we observe in aph-2 adult gonads still relies on GLP-1/Notch signaling. To this end, we used the temperature-sensitive glp-1(e2144) loss-of-function allele to remove GLP-1/Notch activity from aph-2 adult gonads after the larval establishment of a GSC pool. Temperature shifted aph-2(tm6471); glp-1(e2144) hermaphrodites lost the ability to maintain a robust PZ: none of the observed gonads had a PZ of more than 100 cells, and in 54% of the observed gonads, a PZ could barely be detected (fewer than 50 cells, n = 87; a representative example is shown in Fig. 1c). In comparison, temperature-shifted aph-2(tm6471); glp-1(+) control animals continued to maintain a robust PZ of greater than 100 cells (91%, n = 70). These results confirm that the germline PZ observed in adult aph-2 mutant hermaphrodites is dependent on GLP-1/Notch function.
To test whether aph-2 M + Z− adults contain maternally contributed APH-2 protein that could be responsible for promoting GSC fate in aph-2(null) M + Z− adults, we first asked whether maternal APH-2/nicastrin protein could be detected in the distal gonads of aph-2(null) M + Z− adults. We used CRISPR/Cas9 to tag the carboxyl terminus of endogenously encoded APH-2/nicastrin with an OLLAS epitope [aph-2(ik10)]. The tagged APH-2/nicastrin protein remains functional, as homozygous aph-2(ik10) hermaphrodites are viable and produce live progeny. First, we demonstrate that APH-2::OLLAS protein can be readily detected in the distal gonad of homozygous aph-2(ik10) adult hermaphrodites (Supplementary Fig. 4a, first column). Then, we tested the gonads of homozygous aph-2(null) adults derived from aph-2(null)/aph-2(ik10) mothers (M + Z−) but did not find evidence of maternally contributed APH-2::OLLAS protein (Supplementary Fig. 4a, second column). Immunofluorescence intensity of distal gonads from M + Z− adults was indistinguishable from that of negative control animals that contain no source of maternal or zygotic epitope-tagged protein (Supplementary Fig. 4b). Although we cannot rule out low levels of protein that are below detection sensitivity, this analysis demonstrates that aph-2(null) M + Z− adult gonads are significantly depleted of APH-2 protein relative to aph-2(+) M + Z+ adults, and yet this difference does not compromise Notch signaling in the distal germline.
aph-2(null) adults maintain a germline PZ through at least 5 days of adulthood, as assayed in males, but depletion of any other γ secretase subunit causes loss of GSCs
As another way to overcome confounding effects of possible persistent maternal aph-2(+) product, we sought to extend our analysis of aph-2(null) M + Z− animals through later adulthood, to allow more time for full depletion of possible residual maternal products. However, we faced a challenge due to their egg-laying defect: beginning in early adulthood, aph-2 hermaphrodites accumulate embryos that gradually expand the uterus, crowding out other tissues such as the gonads. Gonads dissected from these older bloated aph-2 adults were found to have smaller gonad PZs than those of age-matched wild-type hermaphrodites, and in a few rare cases lacked a PZ altogether (Supplementary Fig. 5). We could not disentangle whether this PZ reduction was a direct consequence of eliminating aph-2/nicastrin function in the distal gonad or an indirect consequence of accumulating pressure from the uterus that might negatively impact germline maintenance. Indeed, adult germline homeostasis has been shown to be highly dependent on the dynamic flux of material from distal to proximal regions of the gonad (Wolke et al. 2007; Nadarajan et al. 2009; Atwell et al. 2015; Chartier et al. 2021). As an alternative way to assess germline maintenance in the later stages of adulthood, we turned to male aph-2 gonads, which are free from the confounding effects of an egg-laying defect and can be assessed through several days of adulthood. Like hermaphrodite gonads, male gonads rely on Notch signaling for maintaining a distal pool of undifferentiated proliferative GSCs throughout adulthood (Austin and Kimble 1987; Morgan et al. 2010).
We focused our analysis on late adulthood (Day 3 and Day 5 adults), since these later stages could not be clearly assessed in hermaphrodites, and compared the requirement for all four γ secretase subunits. Whole worm DAPI staining and germ cell nuclear morphology were used to monitor the status of germ cells, focusing on the maintenance of a PZ through adulthood (Fig. 2). aph-1(tm4743) M + Z− and pen-2(ep220) M + Z− Day 3 adult males exhibit a dramatic loss of GSCs, comparable to that described previously for hop-1(ar179) males (Agarwal et al. 2018). Not only is a germline PZ absent from these Day 3 adults, but virtually all aph-1(tm4743), pen-2(ep220), or hop-1(ar179) gonads contain only mature spermatids [95% (n = 38), 98% (n = 40), and 97% (n = 36), respectively; Fig. 2a]. The absence of germ cells in earlier stages of meiosis or spermatogenesis indicates that the switch from stem cell fate to meiosis must have occurred more than 24 h earlier [the minimum time required for meiotic prophase in males (Jaramillo-Lambert et al. 2007)]. This phenotype suggests that germline proliferation was successfully initiated during early larval development but then ceased by the end of larval development or early adulthood. These results are consistent with a model in which maternally supplied gene products support Notch signaling in the early larval germline, but as maternal products are exhausted, germline maintenance becomes reliant on zygotic expression of aph-1, pen-2, and hop-1 presenilin for PZ maintenance in the adult.
![APH-2/nicastrin is the only γ secretase subunit that is dispensable for adult GSCs, as assayed in older males. a, b) Representative DAPI-stained adult males are shown with gonads outlined in red (the entire worm is not shown). Worms were aged to Day 3 (a) or Day 5 (b) of adulthood. Males are wild type or homozygous null (M + Z−) for one of the four γ secretase subunits [aph-2(ik7), aph-1(tm4743), pen-2(ep220), or hop-1(ar179)]. The male gonad is reflexed back upon itself, with both distal (asterisk) and proximal ends oriented to the right. Wild-type and aph-2 male gonads contain four distinct stages of germ cell development (demarcated with yellow underscoring): the oldest nuclei, located most proximally, are differentiated spermatids (single yellow dotted line); they are followed by primary spermatocytes (double yellow dotted line), early meiotic germ cell nuclei (single dashed yellow line), and most distally, the PZ (thick yellow line). Examples of metaphase germ nuclei (red arrowhead) are detected in wild-type and aph-2 gonads. aph-1, pen-2, and hop-1 gonads contain only spermatids. Animals are positioned with anterior to the left; scale bar is 25 µm. c) The number of germ cells in the PZ is plotted for adult male gonads dissected from wild-type and aph-2(ik7) M + Z− as described for Fig. 1b (n = 10–13 gonads per genotype per time point).](https://oup.silverchair-cdn.com/oup/backfile/Content_public/Journal/genetics/227/3/10.1093_genetics_iyae076/2/m_iyae076f2.jpeg?Expires=1749798376&Signature=3MjxZwWrg7Kb~BBCey8ebq-KCl6kTrPetjLdhRAXr11VxlKoRnEg5~Uk0cciIedxSzL312D7Gc7iwO1WM6SLLNKJHxhgXAfysmDpT82ujYZajyG0POUJJ~bx1sJLF-c7l6roE7csuhDrNcSAJcQpbik~qnoNV0q2308CmjM3sofxwIjn2JJHn1fJw2GJUyVIjROdPQZKLLTj-dm9~afS5CsghXajvLeTTTXRKrJLKZpzcTI0VhZLembSLgFt1nyhpw40kSvJIt3eYzbIFRfo0f95FiKs2~zBxlEN1aS6wfnNQlIyEiIn6RUYREsKgXvehKsAdaEBNb6nfBcPHzumoA__&Key-Pair-Id=APKAIE5G5CRDK6RD3PGA)
APH-2/nicastrin is the only γ secretase subunit that is dispensable for adult GSCs, as assayed in older males. a, b) Representative DAPI-stained adult males are shown with gonads outlined in red (the entire worm is not shown). Worms were aged to Day 3 (a) or Day 5 (b) of adulthood. Males are wild type or homozygous null (M + Z−) for one of the four γ secretase subunits [aph-2(ik7), aph-1(tm4743), pen-2(ep220), or hop-1(ar179)]. The male gonad is reflexed back upon itself, with both distal (asterisk) and proximal ends oriented to the right. Wild-type and aph-2 male gonads contain four distinct stages of germ cell development (demarcated with yellow underscoring): the oldest nuclei, located most proximally, are differentiated spermatids (single yellow dotted line); they are followed by primary spermatocytes (double yellow dotted line), early meiotic germ cell nuclei (single dashed yellow line), and most distally, the PZ (thick yellow line). Examples of metaphase germ nuclei (red arrowhead) are detected in wild-type and aph-2 gonads. aph-1, pen-2, and hop-1 gonads contain only spermatids. Animals are positioned with anterior to the left; scale bar is 25 µm. c) The number of germ cells in the PZ is plotted for adult male gonads dissected from wild-type and aph-2(ik7) M + Z− as described for Fig. 1b (n = 10–13 gonads per genotype per time point).
In striking contrast, the gonads of Day 3 adult aph-2(tm6471) and aph-2(ik7) M + Z− males continue to support germline proliferation in the distal PZ and maintain the distinct assembly-line characteristic of a wild-type gonad in which mature spermatids are only observed in the proximal region (100%, n = 24; 100%, n = 49, respectively, and 100% for wild type, n = 31; Fig. 2a). Even after an additional two days of adulthood, all aph-2 male gonads still contain a robust PZ [n = 44 for aph-2(tm6471); n = 38 for aph-2(ik7); n = 40 for the wild type; Fig. 2b]. In a separate experiment, we measured the number of germ cells in the PZ of gonads dissected from aph-2 males aged through two, three, or five days of adulthood and found them to be largely comparable to those of age-matched wild-type gonads (Fig. 2c).
Despite relatively normal PZ maintenance, we noticed that some aph-2(ik7) gonads appeared to have slightly shortened meiotic regions, with the appearance of differentiated spermatocytes and spermatids shifted slightly distally, as compared to wild-type gonads (for example, Fig. 2a). Further characterization of this phenotype is beyond the scope of this study, but we note that a possible later role for γ secretase and Notch signaling components in the progression of spermatogenesis would have been masked in prior analyses of presenilin or Notch mutants, due to the severe germ cell proliferation defects of these mutants (for example, see hop-1 in Fig. 2a).
By analyzing late adults, these experiments allowed extensive time for the depletion of possible residual maternal gene products remaining in early adult GSCs. However, aph-2 M + Z− adults still failed to exhibit the loss of GSCs phenotype that was readily observed for the other three γ secretase subunits, supporting an essential role for APH-1, PEN-2, and HOP-1, but not APH-2/nicastrin.
Larval germline expansion can be eliminated by aph-1 RNAi but not by aph-2 RNAi
The analyses above fail to find evidence of persistent maternal aph-2(+) gene product as the explanation for continued Notch-dependent germline stem fate in adult aph-2(null) adults. As a final analysis, we used RNAi, which can deplete both maternal and zygotic gene products. Removal of both maternal and zygotic sources of γ secretase is expected to cause a severe glp-1/Notch-like sterility because a proliferative GSC pool is not established during early larval development. Indeed, this has been reported for presenilin (hop-1 and sel-12) and aph-1 depletion by RNAi (Westlund et al. 1999; Goutte et al. 2002). Thus, in these experiments, we scored for sterility and used aph-1 RNAi as a control.
In order to separately assess the effects of RNAi treatment on embryonic and postembryonic events, we collected timed batches of progeny from dsRNA-soaked hermaphrodites. We expected full depletion of aph-1 or aph-2 gene product to cause glp-1/Notch-like embryonic arrest, as is observed in aph-1 or aph-2 (M−Z−) embryos. Arrested embryos with characteristics of failed Notch signaling were first observed in a small number of progeny produced 6–9 h after RNAi treatment and then increased in penetrance in later batches of embryos (Fig. 3, black bars). The similarity in penetrance and timing for the aph-1 and aph-2 RNAi-induced embryonic arrest suggests that the depletion of mRNA and turnover of preexisting protein follow a similar time course for these two maternally supplied gene products and that their depletion causes a similar block to Notch signaling in the early embryo.
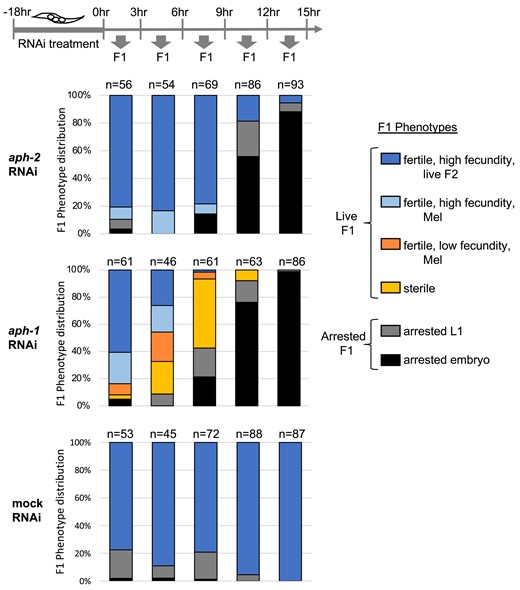
aph-1 RNAi treatment, but not aph-2 RNAi treatment, causes germline sterility among the progeny of treated hermaphrodites. Stacked bar graphs indicate the phenotypes observed among F1 progeny collected in successive 3-h windows after soaking young adult N2 hermaphrodites in dsRNA corresponding to aph-2 (top), aph-1 (middle), or buffer alone (bottom). Combined data from two independent trials (each with 6–8 treated hermaphrodites) are plotted; the total number of progeny analyzed in each time window is indicated above the bars. F1 animals were characterized according to six phenotypic categories shown at right. F1s that displayed low fecundity (<10 embryos, dark orange) or sterility (0 embryos, light orange) were observed among the progeny of aph-1(RNAi)-treated hermaphrodites, but not those of aph-2(RNAi)-treated hermaphrodites. Either aph-1 or aph-2 RNAi treatment ultimately leads to penetrant embryonic lethality (black) among progeny laid 12 h after treatment.
We used the viable progeny produced in the first 10 h after treatment to assess postembryonic aph-1 and aph-2 requirements. Analysis of this early window of viable progeny revealed a dramatic difference between aph-1 and aph-2 RNAi treatments. In the case of aph-1 RNAi-treated mothers, the viable progeny developed into sterile adults or adults with very low fecundity, producing fewer than 10 embryos (Fig. 3, yellow and orange bars). Analysis of a sample of sterile worms by DAPI staining revealed a glp-1/Notch-like mutant phenotype in which one or both gonad arms were devoid of undifferentiated germ cells, and instead the distal gonad was occupied by oocytes (5/14) or spermatids (9/14), consistent with a premature switch from the GSC fate to meiotic development. The highest penetrance of aph-1(RNAi) sterility was observed among progeny laid 6–12 h after RNAi treatment, where 97.5% of viable progeny are sterile or extremely reduced in fecundity (n = 40 viable progeny). In sharp contrast, none of the viable early progeny from aph-2 RNAi-treated hermaphrodites were sterile or severely reduced in fecundity (0%, n = 80 viable progeny laid between 6 and 15 h after RNAi treatment), despite the effectiveness of the aph-2 RNAi treatment at causing penetrant embryonic lethality (Fig. 3).
Additional trials of aph-2 RNAi treatment failed to yield sterile progeny or progeny with low fecundity. This included a trial using a shorter aph-2 dsRNA species (see Materials and methods; 0% sterile progeny, n = 66 viable progeny) or the use of injection as the means of dsRNA delivery. Injected N2 hermaphrodites that yielded more than 40% arrested embryos by 27 h post-injection failed to yield sterile progeny (n = 1153 total viable progeny scored from 18 injected hermaphrodites). Interestingly, many of these viable progeny produced arrested embryos, suggesting that the RNAi effect was transmitted to the next generation, passing over any effect on germline proliferation. In contrast, the sterile phenotype resulting from aph-1 RNAi was robust and repeatable: 100% of aph-1 dsRNA-injected hermaphrodites yielded some live progeny that were sterile (average of 37% sterile progeny per mother; n = 884 total viable progeny scored from 18 injected hermaphrodites).
In a separate set of experiments, we depleted aph-2 simultaneously by RNAi treatment and by zygotic null mutation but still failed to observe a glp-1/Notch-like sterility phenotype. In one approach, aph-2 dsRNA was administered by soaking heterozygous aph-2(tm6471)/aph-2(+) hermaphrodites. A combined total of 46 viable homozygous aph-2(tm6471) progeny were identified, but none were sterile. When the same experiment was repeated using an aph-1 RNAi treatment, 18 out of 19 viable homozygous aph-2(tm6471) progeny were sterile (homozygous aph-2(tm6471) progeny from control mock RNAi treatment yielded 0% sterility, n = 62). In a second approach, aph-2 dsRNA was administered by injection of heterozygous aph-2(ik7)/aph-2(+) hermaphrodites. Among all collected viable progeny, one quarter were identified as fertile Egl Mel aph-2(ik7) homozygotes (211/862) suggesting that virtually all aph-2(ik7) M + Z− animals derived from aph-2 RNAi-injected aph-2(ik7)/aph-2(+) mothers were able to establish a proliferative GSC pool during larval development. In summary, aph-1 and aph-2 RNAi treatment cause an equally penetrant embryonic lethality, but only aph-1 RNAi treatment prevents germline expansion in the larval gonad. This analysis of larval germline establishment corroborates the analysis of adult germline maintenance, together supporting the existence of APH-2/nicastrin-independent Notch signaling in germ cells.
aph-2 null gonads display robust activation of the downstream Notch target gene sygl-1
To probe the mechanism underlying APH-2/nicastrin-independent Notch signal transduction, we asked whether aph-2(ik7) M + Z− gonads could properly induce expression of the GLP-1/Notch target gene sygl-1. In wild-type adult gonads, Notch-dependent sygl-1 transcription is observed in GSCs located in the distal gonad stem cell niche, where Notch ligand is available (Kershner et al. 2014; Lee et al. 2016). The resulting distal-to-proximal gradient of SYGL-1 protein along the gonad provides a readout of Notch activity, as its distribution becomes extended or contracted by glp-1 hypermorphic or hypomorphic mutations, respectively (Lee et al. 2016; Shin et al. 2017). We monitored SYGL-1 protein in aph-2(ik7) and aph-1(tm4743) adult gonads using the endogenously tagged sygl-1(q983[3xOLLAS::sygl-1]) allele (Shin et al. 2017).
aph-2(ik7) gonads display SYGL-1 expression in the distal-most subset of germ cells, consistent with ligand-regulated local activation of Notch receptor. The distal-to-proximal gradient of SYGL-1 protein is comparable to that of wild-type gonads and is observed even through five days of adulthood (Fig. 4a and b). In contrast, aph-1 gonads fail to express SYGL-1 protein above background levels (Fig. 4a and b) and thus resemble GLP-1/Notch-deficient gonads (Kershner et al. 2014).
![aph-2 GSCs have robust expression of Notch downstream target gene sygl-1. a, b) Representative images of distal gonads dissected from Day 1 adult hermaphrodites and Day 5 adult males immunostained for SYGL-1::OLLAS protein. Germ cell nuclei are visualized by DAPI staining. aph-1(tm4743) and aph-2(ik7) animals are M + Z−, and all animals are homozygous for sygl-1(q983[3xOLLAS::sygl-1]). Gonads are oriented with distal end to the left; scale bar is 25 µm. All germ cells in aph-1 gonads are spermatids. c) SYGL-1::OLLAS staining is quantified and plotted according to staining intensity measured in the distal-most five rows of germ cells (n = 20–22 gonads for N2 and aph-2, and n = 15–17 gonads for aph-1), and d) in terms of the number of germ cells that have SYGL-1::OLLAS staining above background (n = 15–16 gonads per genotype for hermaphrodites and n = 19 gonads per genotype for males). Mean (horizontal line) and SD (vertical line) are indicated. P-values are calculated from a Student's 2-tailed t-test, assuming unequal variances.](https://oup.silverchair-cdn.com/oup/backfile/Content_public/Journal/genetics/227/3/10.1093_genetics_iyae076/2/m_iyae076f4.jpeg?Expires=1749798376&Signature=qL3ARGiXVlYhDVLXGyqzaY-zTgyQY9zLRI3-3G5RVeec8eN-z0mf0o9mGhI8sDgY5NZPF7RCExQx--vxX23aMMcNjeIXCRbwkhyct1k7RPVTb6~nETsRFfzZbcye05Rp0csvaZkuf1qG8w7CsPpxFCI89LNPLyE2piQ4gLCvms7SZWi0ubWX3hvV5w81ubkEKcVNT2E-EEvzxlrWIbgv5ZcYnZmmbmi76SZf1exhYV3yUTkiB6hSow6mAJAgoqjBV~MiudmydeuLbmEqspSaaA9MU77J8uFXPFy8~m5DM6xJ7AbJ8Jdt0VOBewV7Ejb2eJYHQVEQvt4dcA1GcxGW1Q__&Key-Pair-Id=APKAIE5G5CRDK6RD3PGA)
aph-2 GSCs have robust expression of Notch downstream target gene sygl-1. a, b) Representative images of distal gonads dissected from Day 1 adult hermaphrodites and Day 5 adult males immunostained for SYGL-1::OLLAS protein. Germ cell nuclei are visualized by DAPI staining. aph-1(tm4743) and aph-2(ik7) animals are M + Z−, and all animals are homozygous for sygl-1(q983[3xOLLAS::sygl-1]). Gonads are oriented with distal end to the left; scale bar is 25 µm. All germ cells in aph-1 gonads are spermatids. c) SYGL-1::OLLAS staining is quantified and plotted according to staining intensity measured in the distal-most five rows of germ cells (n = 20–22 gonads for N2 and aph-2, and n = 15–17 gonads for aph-1), and d) in terms of the number of germ cells that have SYGL-1::OLLAS staining above background (n = 15–16 gonads per genotype for hermaphrodites and n = 19 gonads per genotype for males). Mean (horizontal line) and SD (vertical line) are indicated. P-values are calculated from a Student's 2-tailed t-test, assuming unequal variances.
We asked whether the level or extent of SYGL-1 expression in aph-2 gonads might be reduced compared to that of wild-type gonads but found that aph-2 gonads were able to mount a SYGL-1 response that is indistinguishable from the wild-type response. First, we compared the level of SYGL-1::OLLAS in the distal-most five rows of germ cells (Fig. 4c), which have been shown to have the highest level of sygl-1 transcription and display the most direct contact with the DTC (Lee et al. 2016; Shin et al. 2017). Most aph-2 gonads displayed SYGL-1 levels that were within the range observed for wild-type gonads. Two subtle differences are noted: aph-2(ik7) gonads show greater variability, with 20% exhibiting SYGL-1 expression levels outside of the wild-type range (either higher or lower), and older aph-2(ik7) gonads (male Day 5 adults) exhibit slightly less SYGL-1 expression on average, although individuals often reached wild-type-like SYGL-1 levels (Fig. 4c).
aph-2(ik7) and wild-type gonads were also found to be similar with respect to the total number of germ cells that express SYGL-1::OLLAS: For Day 1 adult hermaphrodites: 164 ± 71 cells for aph-2(ik7) vs 160 ± 27 cells for the wild type (n = 15, n = 16, respectively; Fig. 4d); for Day 5 adult males: 114 ± 32 cells for aph-2(ik7), vs 126 ± 32 cells for the wild type (n = 19 for each; Fig. 4d). Given the distal-to-proximal gradient of SYGL-1 protein, we also asked if aph-2 gonads contain as many high-expressing germ cells as wild-type gonads, and here too found no difference between aph-2 and the wild type: Day 1 adult hermaphrodites: 77 ± 39 cells for aph-2(ik7) vs 72 ± 17 cells for the wild type (n = 14, n = 15, respectively; P = 0.68); Day 5 adult males: 60 ± 29 cells for aph-2(ik7) vs 79 ± 24 for the wild type (n = 19 for each; P = 0.69). Overall, these analyses demonstrate that most aph-2(ik7) gonads are capable of a high level of sygl-1 expression that achieves and maintains a distal-to-proximal gradient of SYGL-1 protein in the adult germline, suggesting that APH-2/nicastrin is not essential for GSCs to achieve a full Notch transcriptional response.
APH-2/nicastrin becomes essential in conditions of compromised GLP-1/Notch functionality
Notch signal transduction requires γ secretase to release NICD from its membrane tether. The current model of γ secretase is that of a complex with four obligatory subunits. We considered two alternative ways of revising this model to account for the apparent dispensability of the APH-2/nicastrin subunit in germline Notch signal transduction: First, APH-2/nicastrin could be an optional γ secretase subunit that is not required in some cell types, such as GSCs. Second, APH-2/nicastrin could serve as a modulatory subunit that functions to boost γ secretase activity, and the normal physiological conditions of GSCs allow sufficient release of GLP-1/Notch NICD without this extra boost. The first model predicts that APH-2/nicastrin removal will always be inconsequential to germline Notch signaling. The second model predicts that altering the abundance or activity of GLP-1/Notch might cause GSCs to require APH-2/nicastrin participation in order to achieve biologically relevant Notch signaling. To distinguish between these two mechanistic models, we tested two different genetic backgrounds that are known to alter GLP-1/Notch functionality.
The temperature-sensitive glp-1(e2144) allele encodes a GLP-1/Notch receptor with an altered ankyrin (ANK) motif in its intracellular domain, which normally interacts with DNA-binding proteins (Priess et al. 1987; Kodoyianni et al. 1992; Tamura et al. 1995; Petcherski and Kimble 2000). At the semipermissive temperature of 20°C, the encoded GLP-1/Notch protein has reduced functionality, as evidenced by a germline PZ that is half the size of wild-type gonads (compare PZ size in controls of Figs. 1 and 5c). If APH-2/nicastrin does not contribute to γ secretase cleavage of GLP-1/Notch in the germline, we expected that removing aph-2 function from 20°C glp-1(e2144) animals would have no effect, analogous to removing aph-2 function from glp-1(+) animals. However, we observed a penetrant adult loss of GSCs phenotype: 70% of aph-2(ik7); glp-1(e2144) and 82% of aph-2(tm6471); glp-1(e2144) Day 1 adult hermaphrodites (M + Z− for aph-2) lack a detectable PZ, and instead contain meiotic cells in the distal gonad (Fig. 5a and b). By contrast, virtually, all Day 1 adult glp-1(e2144); aph-2(+) animals maintain a germline PZ at 20°C. When a PZ is observed in the double mutant, it is much smaller than those of glp-1(e2144) control animals (Fig. 5c). Thus, under these conditions of limited GLP-1/Notch function, the APH-2/nicastrin subunit is now essential for PZ maintenance in the adult germline.
![Sensitized conditions reveal aph-2(null) germline phenotype in adults. a–c) With reduced GLP-1/Notch function [glp-1(e2144) at the semipermissive temperature (20°C)], aph-2/nicastrin is required for adult GSC maintenance. a) Gonads of Day 1 adults (trial 1: 14–27 h post-mid-L4; trial 2: 14–16 h post-mid-L4) were scored for the presence and size of the PZ as in Fig. 1. The distal end of the gonads in the “No PZ” category typically contained germ cells entering meiosis, as evidenced by leptotene nuclear morphology (example in bottom panel of b). aph-2 animals are M + Z− for the indicated allele. b) Representative Day 1 adult gonads labeled as in Fig. 1a; scale bar is 25 µm. c) The number of germ cells in the PZ of Day 1 adult gonads is plotted and analyzed as in Fig. 1b for glp-1(e2144) (n = 34) and glp-1(e2144); aph-2(ik7) M + Z− (n = 41). d) With ectopic GLP-1/Notch function [partially ligand-independent glp-1(ar202)], aph-2/nicastrin is required for high penetrance tumor formation. Animals were raised at the permissive temperature (15°C) to allow larval germline establishment, shifted to the restrictive temperature (25°C) as young adults, and then fixed and DAPI-stained after 24 h (trial 1) or 48 h (trial 2). The “tumor” designation is used for gonads in which all germ cells appear undifferentiated; excessive proliferation of these cells has filled the gonad, and no meiotic germ cells are observed. Gonads were scored as “no tumor” if meiotic germ cells were observed (leptotene or pachytene nuclear morphology), indicating that not all germ cells are maintained in an undifferentiated state. This category includes gonads with an expanded PZ as well as gonads with a normal-sized PZ. All animals are homozygous for glp-1(ar202) derived from heterozygous glp-1(ar202) mothers; the double mutants are also homozygous for the indicated aph-2(null) allele (M + Z−).](https://oup.silverchair-cdn.com/oup/backfile/Content_public/Journal/genetics/227/3/10.1093_genetics_iyae076/2/m_iyae076f5.jpeg?Expires=1749798376&Signature=GoOEO1TahaBgK0x4qbo96-eiGBn3wl-eR0gjAZV8L7~0oZzl3nqqnqDP6gGYuvY~boWl4fNEcr59itJvlPjAGkA1vxUBQfjDcA-sMoZ8lS-set~cGTfJg9FQzmjgNaDj0mlST~TDrhOXeDg8754g9cv6kP24m-4ztG2eNdr3714E8j24SHva3Bwr6JBf~Alxh6l4rjbkHd8jBLGf6HfPypPTzmCRF-Hdj-~sMtnBVy0hduAo~WWrMVM6BsE4THmdjMJwKBjn90qJr-QNlFKR8raf14YCp0Kgh~Wro2FPpXJhdWV2ka3Br9Si4lAjYFW~t1ZvSyk2L1mtV3lcrZPJJQ__&Key-Pair-Id=APKAIE5G5CRDK6RD3PGA)
Sensitized conditions reveal aph-2(null) germline phenotype in adults. a–c) With reduced GLP-1/Notch function [glp-1(e2144) at the semipermissive temperature (20°C)], aph-2/nicastrin is required for adult GSC maintenance. a) Gonads of Day 1 adults (trial 1: 14–27 h post-mid-L4; trial 2: 14–16 h post-mid-L4) were scored for the presence and size of the PZ as in Fig. 1. The distal end of the gonads in the “No PZ” category typically contained germ cells entering meiosis, as evidenced by leptotene nuclear morphology (example in bottom panel of b). aph-2 animals are M + Z− for the indicated allele. b) Representative Day 1 adult gonads labeled as in Fig. 1a; scale bar is 25 µm. c) The number of germ cells in the PZ of Day 1 adult gonads is plotted and analyzed as in Fig. 1b for glp-1(e2144) (n = 34) and glp-1(e2144); aph-2(ik7) M + Z− (n = 41). d) With ectopic GLP-1/Notch function [partially ligand-independent glp-1(ar202)], aph-2/nicastrin is required for high penetrance tumor formation. Animals were raised at the permissive temperature (15°C) to allow larval germline establishment, shifted to the restrictive temperature (25°C) as young adults, and then fixed and DAPI-stained after 24 h (trial 1) or 48 h (trial 2). The “tumor” designation is used for gonads in which all germ cells appear undifferentiated; excessive proliferation of these cells has filled the gonad, and no meiotic germ cells are observed. Gonads were scored as “no tumor” if meiotic germ cells were observed (leptotene or pachytene nuclear morphology), indicating that not all germ cells are maintained in an undifferentiated state. This category includes gonads with an expanded PZ as well as gonads with a normal-sized PZ. All animals are homozygous for glp-1(ar202) derived from heterozygous glp-1(ar202) mothers; the double mutants are also homozygous for the indicated aph-2(null) allele (M + Z−).
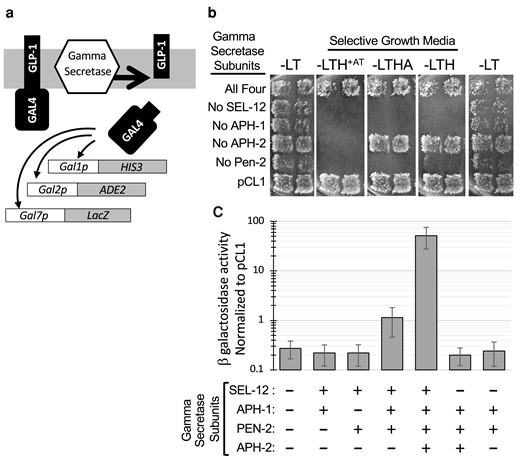
C. elegans γ secretase reconstituted in yeast reveals APH-2/nicastrin-independent enzymatic activity on GLP-1/Notch-like substrate. a) Schematic representation of γ secretase substrate and reporters used to assay γ secretase activity. The substrate is a fusion of the GLP-1/Notch TMD and yeast GAL4. GAL1p, Gal2p, and GAL7p are yeast promoters activated by GAL4. b) Growth assay for expression of HIS3 and ADE2 reporters. Strains were transformed with LEU2 and TRP1 marked plasmids carrying all four γ secretase subunits (SEL-12, APH-1, APH-2/nicastrin, and PEN-2) or only three of these, as indicated. pCL1 is a plasmid that encodes nonmembrane-tethered GAL4, which does not rely on γ secretase activity to access the nucleus. Duplicate patches represent independent transformants assayed in parallel. Growth is shown on four different growth media: −LT (first and last column): no selection for reporter expression; −LTH + AT (3-aminotriazole): highest stringency selection for greatest HIS3 reporter expression; −LTHA: low stringency selection for HIS3 and ADE2 reporter expression; −LTH: low stringency selection for HIS3 reporter expression. c) lacZ reporter expression was quantified by β-galactosidase assay of strains expressing the indicated γ secretase subunits. The average value for 5–10 biological replicates is plotted on log scale as a fraction of the activity measured in a pCL1-containing strain assayed in parallel; error bars represent standard deviation. Significant activity above background is detected in cells that coexpress sel-12, aph-1, and pen-2 (P = 0.0029) and activity is increased roughly 45-fold with the addition of aph-2/nicastrin (P < 0.0001). All other combinations are not significantly different from background (P > 0.25).
In a second set of experiments, we monitored the effect of APH-2/nicastrin removal when GLP-1/Notch signaling occurs in germ cells that are exposed to very little, if any Notch ligand. The temperature-sensitive glp-1(ar202) allele encodes a partially ligand-independent version of GLP-1/Notch that causes a variety of germline patterning defects (Pepper et al. 2003). Here, we use the glp-1(ar202) late-onset tumor phenotype in which germ cells that are outside of the stem cell niche maintain the proliferative GSC fate, in extreme cases causing the entire gonad to fill up with undifferentiated proliferative germ cells at the expense of meiotic cells. GLP-1ar202-induced tumor formation still relies on cleavage by ADAM and γ secretase, as demonstrated by depletion of ADAM-encoding genes adm-4 or sup-17 or the presenilin genes sel-12 and hop-1, which suppresses germline phenotypes of glp-1(ar202) (Pepper et al. 2003; Jarriault and Greenwald 2005; Agarwal et al. 2018). Similarly, we found that late-onset tumor formation was completely suppressed in aph-1(tm4743); glp-1(ar202) hermaphrodites (0% gonads with tumors, n = 52), consistent with the need for APH-1 in activation of GLP-1ar202. If the APH-2/nicastrin subunit is not involved in γ secretase activation of GLP-1/Notch in germ cells, we expected aph-2 depletion not to affect the ability of glp-1(ar202) to cause germline tumors. Interestingly, we found that the penetrance of the glp-1(ar202) germline tumor phenotype was partially suppressed by aph-2(null) alleles (Fig. 5d). Assuming that the glp-1(ar202) mutation allows some, albeit limited, GLP-1/Notch signal transduction in ectopic germ cells (positioned far from the source of ligands), our results suggest that such cells are more likely to achieve Notch activation when the APH-2/nicastrin subunit of γ secretase is present.
Although the above experiments reflect two different nonphysiological conditions, they both uncover a role for the APH-2/nicastrin subunit of γ secretase in facilitating a Notch signaling response in germ cells, a role that appears unnecessary under normal conditions. These results are consistent with the second model proposed above, in which APH-2/nicastrin functions as an accessory subunit that can heighten γ secretase activity, but may not always be required to achieve biologically relevant levels of Notch activation.
Reconstitution of C. elegans γ secretase in yeast reveals enzymatic activity in the absence of the APH-2/nicastrin subunit
A major implication of the model presented above is that a three-part γ secretase complex (presenlin-APH-1-PEN-2) retains sufficient enzymatic activity to cleave physiologically relevant levels of Notch receptor. As an independent test of this idea, we reconstituted the C. elegans γ secretase complex in a heterologous system in which the requirement for each subunit could easily be compared. By dispensing with the need for prior Notch receptor interaction with ligand and S2 cleavage by ADAM proteases to remove the receptor extracellular domain, we could focus specifically on γ secretase enzymatic activity. To this end, we used S. cerevisiae, which lacks endogenous γ secretase activity or subunit orthologs, and has been used to reconstitute active quaternary human γ secretase (Edbauer et al. 2003; Futai et al. 2009). We constructed yeast plasmids to express the subunits of C. elegans γ secretase (see Materials and methods and Supplementary Table 3). To assay the enzymatic activity of reconstituted enzyme complexes, we generated a hybrid substrate that consists of the transmembrane domain from the C. elegans GLP-1/Notch receptor fused to the yeast transcriptional activator GAL4 (Fig. 6a and Materials and methods). This hybrid GAL4 protein is unable to activate GAL4-responsive reporter genes (HIS3, ADE2, and lacZ) unless it is released from its membrane tether by γ secretase cleavage. Thus, the GAL4-inducible nutritional reporters HIS3 and ADE2 allow growth on selective media only if the GLP-1-GAL4 substrate is successfully cleaved by reconstituted γ secretase. The four C. elegans γ secretase subunits, presenilin (either SEL-12 or HOP-1), APH-1, APH-2/nicastrin, and PEN-2, were coexpressed all together or three at a time, and cells were then tested for released GAL4 activity. An important feature of our engineered hybrid γ secretase substrate is that we designed it to precisely mimic the extracellular truncated Notch receptor (NEXT), as predicted from ADAM cleavage at the S2 cleavage site of GLP-1/Notch, 15 residues from the transmembrane domain.
Coexpression of all four C. elegans γ secretase subunits successfully reconstituted γ secretase activity in yeast cells, as evidenced by successful growth on nutritional media that selects for GAL4 reporter expression. Reconstitution was accomplished using either the SEL-12 presenilin (Fig. 6b) or the HOP-1 presenilin (Supplementary Fig. 6), although results were more robust and reproducible with SEL-12. Under the most stringent nutritional selection, which requires high expression of HIS3 (−LTH + AT; see Materials and methods), growth was entirely dependent on the presence of all four γ secretase subunits (panel 2 of Fig. 6b and Supplementary Fig. 6). However, under less stringent nutritional selection, where lower expression of HIS3 is sufficient for growth, cells that lack APH-2/nicastrin were also able to grow, suggesting that some GLP-1-GAL4 substrate had been successfully cleaved (panels 3 and 4 of Fig. 6b and Supplementary Fig. 6). The APH-2/nicastrin subunit was the only subunit that appeared dispensable under these conditions.
To estimate the relative efficiency of substrate cleavage by γ secretase complexes reconstituted with and without the APH-2/nicastrin subunit, we quantified the expression of the lacZ reporter, as measured by β-galactose activity. Under these assay conditions, the presence of the APH-2/nicastrin subunit increases the apparent γ secretase activity of a SEL-12-APH-1-PEN-2 complex by approximately 45-fold (Fig. 6c). It is important to note that cells expressing only SEL-12 and APH-1 or only SEL-12 and PEN-2 did not have enzymatic levels above background, indicating that the observed APH-2/nicastrin-independent activity requires the APH-1 and PEN-2 accessory subunits (Fig. 6c). The lower and more variable levels of reconstituted activity for HOP-1-based γ secretase prevented a similar quantitative measure of the effect of the APH-2/nicastrin subunit, but qualitative growth assays suggest that here too the activity of a quaternary complex is greater than that of a trimeric complex (Supplementary Fig. 6, panel 4). We note that the lower level of HOP-1-based γ secretase activity in our assays may be a result of poor HOP-1 expression in yeast, as preliminary western analysis of crude yeast extracts reveals barely detectable HOP-1 expression compared to strong SEL-12 expression.
This analysis provides evidence that a C. elegans γ secretase complex consisting of only presenilin-APH-1-PEN-2 is capable of cleaving GLP-1/Notch substrate, albeit less efficiently than the quaternary complex that includes APH-2/nicastrin. These results corroborate our in vivo results that point to APH-2/nicastrin-independent GLP-1/Notch activation in C. elegans GSCs. Furthermore, the difference in reconstituted γ secretase activity measured with and without the APH-2/nicastrin subunit adds mechanistic detail to a revised model of γ secretase in which APH-2/nicastrin has a modulatory rather than essential role for Notch activation.
Discussion
This work reveals a cellular context—the maintenance of a stem cell population in the C. elegans germline—in which the APH-2/nicastrin subunit of γ secretase is surprisingly dispensable for Notch signal transduction. This finding challenges the current model of γ secretase, which states that all four subunits are essential for in vivo γ secretase function. Although ample genetic and biochemical data exist to support a quaternary γ secretase structure as the mediator of Notch activation, our analysis brings caution to the assumption that the quaternary structure is the only biologically active form of γ secretase. Instead, our results suggest that in some cellular contexts, a trimeric complex of presenilin, APH-1, and PEN-2 can provide the γ secretase activity necessary to transduce a Notch signaling response. We propose to redefine the APH-2/nicastrin subunit as a modulatory subunit that increases γ secretase activity but is not always essential. We compare the cellular contexts that display differential dependence on APH-2/nicastrin in C. elegans to suggest specific cellular conditions that may dictate the need for the APH-2/nicastrin subunit (Fig. 7). This analysis will point the way to identifying cellular conditions in other systems where Notch signaling may proceed in the absence of the APH-2/nicastrin subunit of γ secretase.

Model for the cellular response of different levels of γ secretase activity. NICD is released from the membrane by γ secretase activity and travels to the nucleus to associate with DNA-binding proteins. Notch extracellular truncation (NEXT) is the substrate of γ secretase and is generated by ligand-dependent ADAM cleavage of Notch receptor. Here, we propose that in addition to the well-characterized quaternary γ secretase complex, a tripartite complex lacking APH-2/nicastrin also has activity, albeit less than the full complex (catalytic core is indicated with an asterisk). Several cellular factors could influence whether or not this lower γ secretase activity is sufficient to generate a biological response. For example, cell types that generate high levels of NEXT may have a lower threshold (#1) requirement for γ secretase activity to elicit a cellular response, and therefore not require the APH-2/nicastrin subunit. In contrast, cell types that are limited in the amount of available NEXT will require a higher threshold (#2) of γ secretase activity to generate a response. Any variable that increases the concentration of available NEXT or increases the stability/activity of NICD could in theory allow threshold #1 to be sufficient for a cellular response. Previously characterized Notch signaling events in C. elegans and other systems would be represented by threshold #2, while our analysis of C. elegans GSCs reveals an example of threshold #1. Our results suggest that C. elegans GSCs can be shifted to APH-2/nicastrin dependency (threshold #2) by compromising the Notch receptor.
Prior evidence for mammalian APH-2/nicastrin-independent γ secretase complexes
Several lines of experimentation with mammalian γ secretase have pointed at the existence of γ secretase complexes that lack the APH-2/nicastrin subunit; however, the lack of biological relevance has left the significance of these complexes unresolved. Coimmunoprecipitation and colocalization studies in cultured cell lines demonstrate that γ secretase complexes can form in the absence of the APH-2/nicastrin subunit, but that such complexes may differ from the quaternary complex in stability and/or subcellular localization. Whether such complexes represent intermediates to quaternary complex assembly or alternative active complexes has remained unclear (Zhang et al. 2005; Futai et al. 2009; Zhao et al. 2010; Mao et al. 2012). Furthermore, a study of embryonic fibroblast cell lines from APH-2/nicastrin knockout mice demonstrated γ secretase cleavage of introduced Notch and APP substrates, although this activity appeared less stable than that observed in wild-type cells (Zhao et al. 2010). A third line of evidence comes from a yeast-based reconstitution of human γ secretase, following mutagenesis of the presenilin subunit. This study screened for dispensability of any γ secretase subunit, and the only subcomplex that yielded detectable γ secretase activity was an APH-1-PEN-2-presenilin complex (Futai et al. 2009). Although this trimeric γ secretase activity was only observed with modified presenilin (two missense mutations), these results support the formation of an active human trimeric γ secretase complex that lacks the APH-2/nicastrin subunit. Our work with C. elegans γ secretase corroborates these findings by presenting the first in vivo evidence of biologically active trimeric γ secretase activity, thus providing a framework for reconsidering the biological relevance of these earlier mammalian studies.
Structural considerations
Mammalian and C. elegans genomes do not contain a second APH-2/nicastrin homolog that is identifiable by protein sequence (Goutte et al. 2000; Yu et al. 2000). It is conceivable that an as-yet uncharacterized and unrelated protein could substitute for APH-2/nicastrin in the γ secretase complex; however, we note that structural analysis of γ secretase does not predict the need for an APH-2/nicastrin substitute. High-resolution analysis of a size-selected quaternary human γ secretase complex describes the APH-2/nicastrin subunit as occupying one end of the complex, contributing one transmembrane domain to the total of 20 transmembrane domains of the quaternary complex. Interestingly, the presenilin catalytic core makes extensive contacts with the APH-1 and the PEN-2 subunits, but no direct contacts with APH-2/nicastrin (Lu et al. 2014; Bai et al. 2015). Thus, the structure of the quaternary γ secretase complex does not exclude the possibility that γ secretase can function without the APH-2/nicastrin subunit.
Contrast with human γ secretase reconstitution studies
The hypothesis that a trimeric presenilin-APH-1- PEN-2 complex can provide sufficient γ secretase activity in C. elegans germ cells is supported by our results with reconstituted C. elegans γ secretase in yeast. In this heterologous system, we show that a GLP-1/Notch-like substrate can be cleaved by γ secretase without the APH-2/nicastrin subunit, albeit to a lesser degree than in the presence of APH-2/nicastrin (Fig. 6). These results contrast with those of yeast reconstitution experiments with wild-type human γ secretase, where APH-2/nicastrin-independent activity was not detected. A key difference between our assays is that different size γ secretase substrates were used to assess enzyme activity. The GLP-1/Notch substrate used in our assays mimics the predicted product of S2 cleavage by ADAM proteases, while previous assays used a human APP-derived substrate that contained 16 extra residues preceding the ADAM S2 cleavage site (Edbauer et al. 2003; Futai et al. 2009) or a mouse Notch-1-based substrate that contained an extra 8 residues preceding the S2 cleavage site (Futai et al. 2009). Although these differences are slight, an inverse relationship between measured γ secretase activity and the size of the substrate extracellular domain has been reported (Struhl and Adachi 2000). Thus, the extra residues included in the substrates for human γ secretase reconstitution may have reduced the sensitivity of these assays. For example, quantitative measurement of the induced lacZ reporter by the human quaternary γ secretase was recorded as 2% of the positive control [a soluble GAL4 that does not need to be released from the membrane (Edbauer et al. 2003)]. In comparison, in our assay of quaternary C. elegans γ secretase (Fig. 6c), the induced lacZ reporter is 52% of the positive control (the same soluble GAL4 control used by Edbauer et al.). Albeit crude, this comparison indicates that the prior assays of human γ secretase may have had very little room to detect activity from subcomplexes with reduced activity relative to the four-part complex.
C. elegans evidence for APH-2/nicastrin dispensability and consideration of maternal aph-2 gene product
New deletion alleles of aph-2/nicastrin and aph-1 cause expected Notch-like mutant phenotypes in the context of early embryonic development and larval vulval/uterine cell development. However, in the context of germline development, only aph-1(null) mutants fail to support Notch-dependent GSC maintenance in adults (Figs. 1 and 2) or larval germline establishment in a presenilin-sensitized background (Table 1). The germline phenotype of aph-1(null) animals is similar to that reported for pen-2 and presenilin hop-1 mutants (Fig. 2 and Westlund et al. 1999; Francis et al. 2002; Agarwal et al. 2018).
One possible explanation for the lack of aph-2 germline phenotype is that maternal aph-2(+) product persists in the gonads of zygotic aph-2 null (M + Z−) mutants. We cannot rule out the presence of residual maternal product in our experiments; however, several observations suggest that this possibility is unlikely to explain the observed Notch signaling in aph-2/nicastrin mutants. First, the behavior of the other three γ secretase components is consistent with that of other maternally supplied germline-acting genes, where maternal product functions during early larval germline establishment but is depleted during larval development, yielding adult-onset phenotypes in zygotic M + Z− mutants (Fig. 2). For example, maternally supplied mes-2 and mes-4 gene products can be detected in GSCs of L1-stage larvae but become undetectable by the L3 stage (Gaydos et al. 2012). Thus, support for the perdurance of functional maternal aph-2 product for several days beyond larval development would require invoking a novel mechanism to account for the unique stabilization and retention of aph-2 maternal gene product through successive rounds of germ cell division. Second, the use of RNAi to deplete both maternal and zygotic aph-2 gene products causes the expected maternal-effect embryonic lethal phenotype but fails to cause the germline sterility phenotype observed in parallel aph-1(RNAi)-treated animals (Fig. 3). Lastly, direct visualization of APH-2/nicastrin protein in the gonad fails to detect maternal protein, while zygotically encoded protein is readily detected (Supplementary Fig. 4). Although each of these experimental approaches has inherent limitations, in combination they lead us to favor the simpler interpretation that maternal aph-2(+) gene product, like other maternal gene products, is expended during larval development and thus cannot be responsible for the robust Notch signaling observed in adult aph-2 zygotic null gonads. Instead, our data are more consistent with a unique dispensability of the APH-2/nicastrin subunit in the context of GSC Notch signaling.
Model of context-specific requirement for APH-2/nicastrin
Our analysis of reconstituted γ secretase complexes and aph-2/nicastrin mutants provides further insight into understanding APH-2/nicastrin-independent Notch signaling. We use these results to offer a simple unifying model of the APH-2/nicastrin contribution to γ secretase activity, consistent with results in both C. elegans and mammalian cells. We propose that both trimeric and quaternary γ secretase complexes can execute substrate cleavage, but the presence of the APH-2/nicastrin subunit significantly increases activity (Fig. 7). Many cellular Notch signaling events may have a high threshold for γ secretase activity, and therefore, the APH-2/nicastrin subunit will appear essential for Notch signaling. However, we propose that some cellular contexts may have a lower threshold for the necessary γ secretase activity required to elicit a Notch signaling response, and in these contexts, the trimeric γ secretase may generate sufficient activity without APH-2/nicastrin (Fig. 7). Here, we discuss specific results that support this model and then discuss cellular features that could allow a lower threshold for γ secretase activity and hence distinguish conditions that require APH-2/nicastrin from those that do not.
APH-2/nicastrin as a modulatory subunit
Comparison of enzymatic activity of reconstituted C. elegans γ secretase complexes in yeast cells reveals 45-fold more activity by the quaternary complex than the trimeric complex (Fig. 6c). A range of molecular differences, such as assembly, stability, or trafficking of the complexes could contribute to differences in activity. In wild-type mammalian fibroblast lines, the subcellular localization of γ secretase has been shown to be intimately tied to its assembly (reviewed in Spasic and Annaert 2008). It is conceivable that without the large extracellular domain of APH-2/nicastrin, a trimeric subcomplex may be distributed throughout the endomembrane system differently, or more dynamically, than the quaternary complex. Another distinction between such complexes may lie in their ability to interact directly with substrate. The APH-2/nicastrin subunit has been specifically implicated as a docking site for substrate (Shah et al. 2005) or a site of substrate discrimination (Bolduc et al. 2016).
In vivo support for a modulatory rather than essential role for APH-2/nicastrin is provided by analysis of Notch-dependent GSC maintenance in aph-2-depleted gonads. A trimeric γ secretase complex (presenilin-APH-1-PEN-2) appears to be sufficient for Notch signal transduction in GSCs, as measured by the ability of aph-2 mutant GSCs cells to activate the Notch downstream target gene sygl-1 (Fig. 4), maintain the undifferentiated GSC fate in adults (Figs. 1 and 2), and establish a productive germline during larval development (Fig. 3). Despite its dispensability, we demonstrate that APH-2/nicastrin is expressed in distal GSCs (Supplementary Fig. 4). Two lines of genetic analyses reveal a function for APH-2/nicastrin in GSCs under compromised conditions and are consistent with increased γ secretase activity for a quaternary complex as compared to a trimeric complex.
In the first experiment, the glp-1(e2144) allele introduces a point mutation in the GLP-1/NICD, specifically in one of the ANK domains, which normally interact with DNA-binding proteins (Kodoyianni et al. 1992; Tamura et al. 1995). Interestingly, this single change appears to convert germ cells from APH-2/nicastrin independence to APH-2/nicastrin dependence (compare the lack of effect of aph-2 null mutations in Fig. 1 with the strong effect shown in Fig. 5a–c). ANK domain mutations have been shown to decrease the binding efficiency of GLP-1 NICD with its nuclear binding partners LAG-1/CSL and LAG-3/SEL-8/MAM (Petcherski and Kimble 2000). If GLP-1e2144 NICD has a similar reduced efficiency at interacting with its DNA-binding partners, then it is conceivable that a greater concentration of NICD would be required in the nucleus to ensure sufficient DNA-binding complex formation. Because NICD is released from the membrane by γ secretase, it is reasonable to consider γ secretase activity as a limiting factor in the accumulation of nuclear NICD. Thus, a possible interpretation of our results is that a low level of trimeric γ secretase activity is sufficient for wild-type GLP-1 to elicit a Notch signaling response in germ cells, but for the compromised GLP-1e2144 a higher level of γ secretase activity, achievable with the APH-2/nicastrin subunit, is necessary.
The amount of released NICD is also expected to be limited by the availability of the substrate for γ secretase, which in turn depends on ligand–receptor interaction. Interaction of Notch receptor with its ligand triggers metalloprotease-mediated removal of the Notch extracellular domain; the resulting extracellular truncated Notch receptor (NEXT) is the substrate for γ secretase (illustrated in Fig. 7). In a second experiment, the glp-1(ar202) mutation was used to generate some ligand-independent NEXT in germ cells outside of the stem cell niche. The amount of NEXT generated in ectopic cells likely represents only a fraction of the total available receptor because glp-1(ar202) animals are still sensitive to ligand (McGovern et al. 2009) and exhibit a less severe and less penetrant phenotype than the fully ligand-independent allele glp-1(oz112) (Berry et al. 1997; Hansen, Wilson-Berry, et al. 2004). We found that the propensity of glp-1(ar202) ectopic cells to mount a Notch response (maintain the undifferentiated state) is augmented by the presence of the APH-2/nicastrin subunit (Fig. 5d). A possible explanation of this result is that the limited amount of NEXT generated in ectopic glp-1(ar202) germ cells does not produce sufficient nuclear NICD unless APH-2/nicastrin is present to increase γ secretase activity. In contrast, germ cells residing in the stem cell niche may generate saturating amounts of NEXT such that a lower threshold of γ secretase activity generates sufficient NICD for a Notch signaling response. Interestingly, the transcriptional Notch response of germ cells in the distal stem cell niche does not appear to be limited by ligand or receptor levels, as transcriptional output cannot be increased by the glp-1(oz112) mutation, which renders the notch receptor fully ligand independent (Lee et al. 2016). These results suggest that the amount of NEXT produced in wild-type germ cells residing in the stem cell niche may already be saturated, lending a possible explanation as to why lower levels of γ secretase activity, achievable by a trimeric complex, may still achieve a successful Notch signaling response. Together, these experiments suggest that normal conditions do not require participation of APH-2/nicastrin for a Notch signaling output, but that its presence may be critical under compromised conditions.
Cellular features that may distinguish cells that require APH-2/nicastrin from those that do not
The dispensability of APH-2/nicastrin in GSCs stands in sharp contrast to its essential role in other Notch signaling events in C. elegans (Table 1 and Goutte et al. 2000; Levitan et al. 2001; Francis et al. 2002). We surmise that key cellular differences must be responsible for the differential dependence on the APH-2/nicastrin subunit, and uncovering such differences will help to predict cellular contexts in other systems in which a trimeric γ secretase complex may be sufficient for Notch signal transduction. Here, we draw from our results as well as the extensive knowledge base of Notch signaling in the early embryo and in GSCs (both of which rely on the GLP-1/Notch receptor) to discuss four cellular features that could influence whether or not a Notch signaling response requires the APH-2/nicastrin subunit of γ secretase.
First, the abundance of γ secretase substrate (NEXT) may dictate whether or not a higher threshold of γ secretase activity is needed for Notch signal transduction. Factors that are expected to directly influence the levels of NEXT in a particular cellular context include the concentration of cell-surface Notch receptor, the level and duration of ligand exposure, and the availability and efficiency of resident metalloproteases that cleave the Notch receptor in response to ligand–receptor interaction. For example, C. elegans early embryonic cells, which rely on maternal sources of GLP-1/Notch receptor, may have far less available receptor than germ cells of the distal gonad, which actively transcribe glp-1/Notch (Crittenden et al. 1994; Evans et al. 1994; Mickey et al. 1996; Berry et al. 1997). Similarly, the early embryo may be limited by very low levels of ligand due to the maternal source of ligand as well as the short window of time (less than 1 h) in which interacting cells are juxtaposed (Mickey et al. 1996; Shelton and Bowerman 1996). In comparison, distal germ cells may be exposed to higher levels of ligand due to the longer duration of GSC-stem cell niche association and/or the active transcription of two sources of ligand (lag-2 and apx-1) by the DTC stem cell niche (Henderson et al. 1994; Tax et al. 1994; Nadarajan et al. 2009). Furthermore, ADAM metalloprotease function for germ cells is provided redundantly by at least two genes, sup-17 and adm-4, whereas the early embryo relies solely on maternally encoded sup-17 (Tax et al. 1997; Jarriault and Greenwald 2005). Any one of these differences could result in comparatively less NEXT available for γ secretase in early embryonic cells as compared to germ cells of the distal gonad, thus defining distinct thresholds of necessary γ secretase activity in order to achieve sufficient nuclear NICD.
Second, molecular steps subsequent to γ secretase cleavage may determine whether or not a low threshold of cleavage activity is sufficient to elicit a Notch signaling response. For example, the efficiency with which released NICD can traffic to the nucleus, the efficiency with which NICD is degraded, and the availability of DNA-binding partners for association into stable transcription regulatory complexes all may establish different thresholds for the amount of released NICD necessary to elicit a cellular response. For any of these reasons, a low level of γ secretase activity in C. elegans GSCs may result in sufficient levels of nuclear NICD to sustain the stem cell fate, thus making the APH-2/nicastrin subunit superfluous in this context. In comparison, in early embryonic cells, low levels of APH-2/nicastrin-independent γ secretase activity may exist but may not generate sufficient nuclear NICD to prompt cell fate induction, thus requiring a higher threshold for γ secretase activity. It is intriguing to consider that high thresholds for γ secretase activity may be necessary in circumstances where accuracy is critical, such as in the early embryo, where inappropriate Notch activation in one cell would lead to misspecification of blastomere fate, and embryonic lethality. In contrast, a low threshold for necessary γ secretase activity may offer less distinction from background levels of ligand-independent Notch activation, but such a distinction may be less critical in a population of stem cells where inappropriate induction of any one cell will have little impact on the reproductive fitness of the animal or viability of its progeny.
A third possible determinant in whether Notch signaling relies on APH-2/nicastrin could be the extracellular context in which Notch receptor is activated. Notch signaling events of the early C. elegans embryo occur between rapidly dividing blastomeres that are only transiently in contact, whereas the juxtaposition of GSCs with the ligand-presenting DTC is much more extensive. The DTC extends processes that intercalate between the underlying germ cells, creating an intimate GSC niche (Hall et al. 1999; Byrd et al. 2014; Crittenden et al. 2019). The enwrapment of germline progenitor cells begins early in the development of the gonad and has been shown to promote GLP-1/Notch signaling between the DTC and the germ cell progenitors (Linden et al. 2017; Gordon et al. 2019). It is conceivable that the more stable and elaborate environment of the gonad stem cell niche might allow a lower threshold of γ secretase activity to be sufficient. Although purely speculative, this idea cautions a more context-dependent interpretation of required Notch signaling components.
Another potential influence on γ secretase activity could be the nature of the presenilin subunit; however, our data do not support this distinction as the explanation for dependence on the APH-2/nicastrin subunit. The two C. elegans presenilins, SEL-12 and HOP-1, are functionally redundant for Notch signaling in the embryo and in early larval GSCs (Li and Greenwald 1997; Westlund et al. 1999), but adult GSC Notch signaling relies exclusively on the HOP-1 presenilin (Agarwal et al. 2018). If the HOP-1 presenilin was uniquely responsible for APH-2/nicastrin-independent γ secretase activity, then removal of SEL-12 would be predicted to allow APH-2/nicastrin-independent Notch signaling in the early embryo or in larval GSCs, but neither of these is observed in our experiments: APH-2/nicastrin remains essential for embryogenesis and dispensable for larval GSC expansion, regardless of which presenilin is used (Table 1). Two other results that fail to support this explanation are the yeast reconstitution assays, which reveal APH-2/nicastrin independent activity for both SEL-12 and HOP-1-based complexes (Fig. 6 and Supplementary Fig. 6), and the conversion of adult GSCs to APH-2/nicastrin dependence by compromising GLP-1/Notch functionality. Together, these results suggest that cellular factors other than presenilin identity determine whether or not the APH-2/nicastrin subunit is necessary for in vivo Notch signal transduction.
Our model predicts that APH-2/nicastrin-independent Notch activation may be observed in other characterized Notch signaling events. A common feature will be a low threshold of γ secretase activity required for Notch signal transduction, but the cellular features that allow low γ secretase activity to be consequential may differ, with some possible examples offered above. Interestingly, the C. elegans germline is a highly dynamic tissue that changes over the course of development and aging, and responds to physiological and environmental influences (reviewed in Hubbard and Schedl 2019). We speculate that in order to ensure stem cell proliferation in this dynamic background, cellular features must be tuned for robust Notch signal transduction. Such robustness may allow a low threshold of γ secretase activity to be sufficient for Notch signal transduction, while the presence of APH-2/nicastrin may buffer the system against a range of physiological changes by boosting γ secretase activity beyond this minimal threshold.
Data availability
All strains and plasmids are available upon request. Supplementary Table 1 lists the C. elegans strains used in this work; Supplementary Table 2 lists primer sequences, and Supplementary Table 3 lists yeast expression plasmids used in this work. New deletion alleles generated for this work will be available at the Caenorhabditis Genetics Center (CGC). The authors affirm that all data necessary for confirming the conclusions of the article are present within the article, figures, and tables.
Supplemental material available at GENETICS online.
Acknowledgments
We are grateful to the National BioResource Project of Japan, which is part of the International C. elegans Gene Knockout Consortium, for generating the aph-1(tm4743) and aph-2(tm6471) alleles, and the Caenorhabditis Genetics Center for providing other strains used in this work. We are grateful to Harold Steiner (Munich, Germany), Charles Miller (New Orleans, LA), and Peter Pryciak (Worcester, MA, USA) for providing reagents for the yeast reconstitution. We thank David Nam, Isabel Paine, and Carlos Cosme for contributions to this work, and Lampros Panagis for confocal microscopy assistance. We thank Ken Kemphues, Diane Morton, Iva Greenwald, Jeremy Nance, Niels Ringstad, and Jane Hubbard for valuable discussions. Some C. elegans strains were provided by the Caenorhabditis Genetics Center, which is funded by the National Institutes of Health Office of Research Infrastructure Programs (P40 OD-010440). This work was supported by the Amherst College Gregory S. Call Student Research Fund and the H. Axel Schupf ’57 Fund for Intellectual Life.
Funding
Confocal microscopy was made possible through a Major Research Instrumentation Award from the U.S. National Science Foundation (2117798).
Literature cited
Author notes
Conflicts of interest The authors declare no conflicts of interest.