-
PDF
- Split View
-
Views
-
Cite
Cite
Michelle K Smith, William B Wood, Teaching Genetics: Past, Present, and Future, Genetics, Volume 204, Issue 1, 1 September 2016, Pages 5–10, https://doi.org/10.1534/genetics.116.187138
- Share Icon Share
Abstract
Genetics teaching at the undergraduate level has changed in many ways over the past century. Compared to those of 100 years ago, contemporary genetics courses are broader in content and are taught increasingly differently, using instructional techniques based on educational research and constructed around the principles of active learning and backward design. Future courses can benefit from wider adoption of these approaches, more emphasis on the practice of genetics as a science, and new methods of assessing student learning.
Past
One hundred years ago when the journal GENETICS was first published, undergraduate genetics classes were primarily focused on patterns of inheritance. Mendel’s principles had been recently rediscovered and verified by contemporary researchers (reviewed in Mawer 2006). One widely used text, Robert Lock’s 1906 book (Lock 1906), is said to have inspired many notable geneticists including H. J. Müller, A. H. Sturtevant, and R. A. Fisher (Edwards 2013). In a section entitled “Mendelism,” Lock details crosses in a variety of organisms and concludes with a practical discussion of applying Mendel’s principles to agricultural breeding, including a plea for using precise scientific methods rather than guesswork. The desire to improve agricultural varieties helped fuel the rise of eugenics, and courses on this topic began to be offered at several land grant institutions in the mid-1910s (Glenna et al. 2007).
Little is known about the instructional strategies that were used in 1916, but we can assume that teaching was primarily by lecturing, the traditionally accepted mode of instruction in universities since their origin (Brockliss 1996). Many U.S. biology classes were described as “lecture with conventional laboratory” (Hollister 1939) and genetics courses were described in catalogs as surveys with “general treatment of facts and theories” (University of Wisconsin 1915; University of Maine 1915).
Present
The subject matter and our ways of thinking about and teaching genetics have broadened tremendously since 1916. Then a young, emerging field, genetics is now the foundation for understanding biological “Information Flow, Exchange, and Storage,” one of the five core concept areas that all undergraduate biology students should master according to the Vision and Change report (American Association for the Advancement of Science 2011). Genetics has also become highly relevant to students’ lives, with news stories nearly every day about its impacts on health and society (Redfield 2012).
A growing awareness of problems with traditional instruction
In addition to the explosion of genetics content knowledge, important changes are taking place in how undergraduate genetics and other science courses are taught, largely in response to two recent developments. First, despite the national need for more scientists and engineers in the workforce, nearly half of all students who enter undergraduate degree programs in science, technology, engineering, or mathematics (STEM) either switch into a non-STEM field or leave college altogether (Chen 2013), most often within the first 2 years (Watkins and Mazur 2013). As a prominent reason, they identify ineffective instructional practices, such as lecturing “straight from the book” (Seymour and Hewitt 2000). Second, a large body of education research has shown that teaching exclusively by lecturing is not the most effective way to help undergraduate students master STEM concepts (reviewed in Handelsman et al. 2004; Wood 2009; American Association for the Advancement of Science 2011). A recent, comprehensive meta-analysis (Freeman et al. 2014) indicates that student learning is substantially increased and the drop-out rate decreased in STEM courses that devote class time to “active learning” through student-centered instructional techniques, such as clicker questions with peer discussion and other group activities requiring analytical thinking (Mazur 1997; Wood 2004; Vickrey et al. 2015). Although systematic collection of data on undergraduate faculty instructional practices is still in its infancy (Wieman and Gilbert 2014), recent surveys and observation studies show that STEM faculty are gradually moving toward supplementing or replacing lectures with active-learning activities in their classrooms (Eagan et al. 2014; Smith et al. 2014; Lewin et al. 2016).
Implementing active learning
The emerging active-learning instructional strategies, derived from work in the learning sciences, are being brought into STEM classes with the help of scientist educators who have expertise in their discipline as well as in pedagogy and have been trained in discipline-based education research (National Research Council 2012; Kober 2015). For example, biology education research, which is becoming recognized as a biological subdiscipline, has identified common persistent conceptual difficulties among biology students and evaluated novel teaching strategies to help students master difficult concepts.
Several evidence-based active-learning instructional strategies lend themselves well to undergraduate genetics courses. An example is clicker questions, which give students the opportunity to practice solving genetics problems in class and allow instructors to monitor student learning in real time. Research on the instructional value of clicker questions, much of it initially performed in genetics classes (e.g., Smith et al. 2009), demonstrated that students learn from talking to their peers during group discussion of the questions. Moreover, when students whose interest has been “primed” by such a discussion, are then given an instructor’s explanation of the answers learning can be increased still further (Smith et al. 2011).
For instructors who wish to learn more about incorporating active learning into their classrooms, a few resources are listed in Table 1. Additional materials can be found at Genetics Society of America Peer-Reviewed Education Portal (GSA PREP), in biology education research journals such as CBE-Life Sciences Education and Journal of Microbiology and Biology Education, and occasionally in genetics research journals.
Resources for evidence-based, active-learning teaching strategies
Resource . | Website URL . | Description . |
---|---|---|
Carl Wieman Science Education Initiative resources webpage | http://www.cwsei.ubc.ca/resources/instructor_guidance.htm | Concise documents about how to integrate active-learning instructional strategies into the classroom, videos about how to use clickers and in-class small-group activities, references to research studies about these instructional strategies, etc. |
CourseSource: GSA Genetics Learning Framework | http://coursesource.org/courses/genetics | Sample genetics learning goals and links to active-learning activities. |
iBiology Scientific Teaching Series | http://www.ibiology.org/scientific-teaching/active-learning.html | A collection of videos about active learning. |
Partnership for Undergraduate Life Sciences Education (PULSE) resources webpage | http://www.pulsecommunity.org/page/resources | Frameworks for encouraging departmental transformation toward evidence-based teaching, rubrics for assessing departmental progress, etc. |
CUREnet webpage | https://curenet.cns.utexas.edu/ | Resources for designing course-based undergraduate research experiences (CUREs). |
Resource . | Website URL . | Description . |
---|---|---|
Carl Wieman Science Education Initiative resources webpage | http://www.cwsei.ubc.ca/resources/instructor_guidance.htm | Concise documents about how to integrate active-learning instructional strategies into the classroom, videos about how to use clickers and in-class small-group activities, references to research studies about these instructional strategies, etc. |
CourseSource: GSA Genetics Learning Framework | http://coursesource.org/courses/genetics | Sample genetics learning goals and links to active-learning activities. |
iBiology Scientific Teaching Series | http://www.ibiology.org/scientific-teaching/active-learning.html | A collection of videos about active learning. |
Partnership for Undergraduate Life Sciences Education (PULSE) resources webpage | http://www.pulsecommunity.org/page/resources | Frameworks for encouraging departmental transformation toward evidence-based teaching, rubrics for assessing departmental progress, etc. |
CUREnet webpage | https://curenet.cns.utexas.edu/ | Resources for designing course-based undergraduate research experiences (CUREs). |
Resource . | Website URL . | Description . |
---|---|---|
Carl Wieman Science Education Initiative resources webpage | http://www.cwsei.ubc.ca/resources/instructor_guidance.htm | Concise documents about how to integrate active-learning instructional strategies into the classroom, videos about how to use clickers and in-class small-group activities, references to research studies about these instructional strategies, etc. |
CourseSource: GSA Genetics Learning Framework | http://coursesource.org/courses/genetics | Sample genetics learning goals and links to active-learning activities. |
iBiology Scientific Teaching Series | http://www.ibiology.org/scientific-teaching/active-learning.html | A collection of videos about active learning. |
Partnership for Undergraduate Life Sciences Education (PULSE) resources webpage | http://www.pulsecommunity.org/page/resources | Frameworks for encouraging departmental transformation toward evidence-based teaching, rubrics for assessing departmental progress, etc. |
CUREnet webpage | https://curenet.cns.utexas.edu/ | Resources for designing course-based undergraduate research experiences (CUREs). |
Resource . | Website URL . | Description . |
---|---|---|
Carl Wieman Science Education Initiative resources webpage | http://www.cwsei.ubc.ca/resources/instructor_guidance.htm | Concise documents about how to integrate active-learning instructional strategies into the classroom, videos about how to use clickers and in-class small-group activities, references to research studies about these instructional strategies, etc. |
CourseSource: GSA Genetics Learning Framework | http://coursesource.org/courses/genetics | Sample genetics learning goals and links to active-learning activities. |
iBiology Scientific Teaching Series | http://www.ibiology.org/scientific-teaching/active-learning.html | A collection of videos about active learning. |
Partnership for Undergraduate Life Sciences Education (PULSE) resources webpage | http://www.pulsecommunity.org/page/resources | Frameworks for encouraging departmental transformation toward evidence-based teaching, rubrics for assessing departmental progress, etc. |
CUREnet webpage | https://curenet.cns.utexas.edu/ | Resources for designing course-based undergraduate research experiences (CUREs). |
Setting learning goals and assessing what students have learned
Although biology educators agree on the value of active-learning activities to replace lecturing in the classroom, this change alone will not meet the broader goal of greater student science literacy without improvements in course design and assessments. Rather than a syllabus listing the topics and factual knowledge that a course will cover, educators advocate using the principle of “backward design” to formulate specific learning goals at the start of a course, in a form such as: “after completing this unit, semester, etc., students should be able to calculate the probability that an individual in a pedigree has a particular genotype” (Wiggins and McTighe 2005; Simon and Taylor 2009; Smith and Perkins 2010). These goals should go beyond memorizing factual information, aiming instead for higher levels of conceptual understanding as demonstrated by the ability to apply knowledge to new situations.
To know what students are learning, instructors need multiple assessments aligned with their learning goals. On a day-to-day basis, clicker questions and other classroom activities provide ongoing (formative) assessment of whether students are “getting it,” in addition to promoting student learning.
To measure learning over an entire unit or course, many instructors are now using published assessments known as concept inventories (Adams and Wieman 2010). Typically given to students at the beginning and end of an undergraduate genetics course, these assessments are designed to test for common conceptual difficulties, measure changes in students’ higher-order conceptual mastery over time, and help faculty identify areas where improvements in their instruction are needed (Smith and Knight 2012). Concept inventories target a range of topics from common learning goals in genetics courses to specific topics, such as meiosis, within a course (Table 2). Sample questions from the Genetics Concept Assessment are shown in Figure 1 (Smith et al. 2008).
Concept inventories that target learning goals typically aligned with genetics courses
Concept inventory name . | Reference . |
---|---|
Genetics Literacy Assessment | Bowling et al. 2008 |
Genetics Concept Assessment | Smith et al. 2008 |
Genetics Diagnostic | Tsui and Treagust 2009 |
Meiosis Concept Inventory | Kalas et al. 2013 |
Genetic Drift Inventory | Price et al. 2014 |
Molecular Biology Capstone Assessment | Couch et al. 2015 |
Central Dogma Concept Inventory | Newman et al. 2016 |
Lac Operon Concept Inventory | Stefanski et al. 2016 |
Concept inventory name . | Reference . |
---|---|
Genetics Literacy Assessment | Bowling et al. 2008 |
Genetics Concept Assessment | Smith et al. 2008 |
Genetics Diagnostic | Tsui and Treagust 2009 |
Meiosis Concept Inventory | Kalas et al. 2013 |
Genetic Drift Inventory | Price et al. 2014 |
Molecular Biology Capstone Assessment | Couch et al. 2015 |
Central Dogma Concept Inventory | Newman et al. 2016 |
Lac Operon Concept Inventory | Stefanski et al. 2016 |
Concept inventory name . | Reference . |
---|---|
Genetics Literacy Assessment | Bowling et al. 2008 |
Genetics Concept Assessment | Smith et al. 2008 |
Genetics Diagnostic | Tsui and Treagust 2009 |
Meiosis Concept Inventory | Kalas et al. 2013 |
Genetic Drift Inventory | Price et al. 2014 |
Molecular Biology Capstone Assessment | Couch et al. 2015 |
Central Dogma Concept Inventory | Newman et al. 2016 |
Lac Operon Concept Inventory | Stefanski et al. 2016 |
Concept inventory name . | Reference . |
---|---|
Genetics Literacy Assessment | Bowling et al. 2008 |
Genetics Concept Assessment | Smith et al. 2008 |
Genetics Diagnostic | Tsui and Treagust 2009 |
Meiosis Concept Inventory | Kalas et al. 2013 |
Genetic Drift Inventory | Price et al. 2014 |
Molecular Biology Capstone Assessment | Couch et al. 2015 |
Central Dogma Concept Inventory | Newman et al. 2016 |
Lac Operon Concept Inventory | Stefanski et al. 2016 |
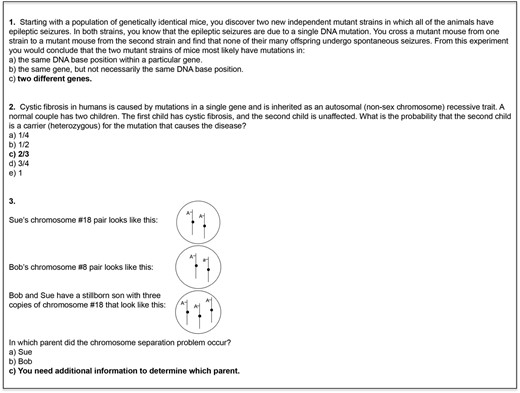
Three sample questions from the Genetics Concept Assessment (Smith et al. 2008). Correct answers are shown in boldface type.
Future
Measuring and rewarding effective teaching
If the move toward more effective, evidence-based instruction is to succeed on a national scale, we will need to have ways to collect systematic data on instructional practices and student learning from a variety of institutions (Wieman and Gilbert 2014). At the same time, we will need to change the reward structure at colleges and universities so that excellence in teaching is recognized. Measuring teaching effectiveness is more difficult than measuring research productivity, and the commonly used method of student evaluations is rightfully suspect (e.g., MacNell et al. 2015). Nevertheless, evaluation of both instructional practices and teaching effectiveness should be possible with a combination of: (1) better-designed student evaluations, (2) faculty self-reporting (Wieman and Gilbert 2014), (3) classroom observations of instructional practice using objective protocols designed for this purpose (e.g., Smith et al. 2013; Hora and Ferrare 2014), and (4) measurements of student learning using published concept inventories (Table 2).
What should we be teaching genetics students?
Of course as the science of genetics progresses through the 21st century, the content we teach must change accordingly. For example, there are now calls for spending less time on simple genetic crosses in which single genes cause phenotypic outcomes and introducing students to complex quantitative traits from the beginning of the course (Redfield 2012).
However, a more fundamental shift of emphasis may also be in order. Over the past 15 years, biology education research and the resulting efforts to transform traditional courses have focused primarily on how we can best teach, but attention is now shifting to include discussion of what we should be asking students to learn. Traditional courses have typically required memorizing large amounts of factual information without going beyond a superficial level of understanding, particularly at the introductory level (National Research Council 2012). In an age where almost all such information is readily found online, this kind of course is no longer optimal. Instead, we should be helping students develop a solid conceptual understanding of principles as well as tools for sifting through the massive amount of information available and evaluating the evidence behind it.
Three recent major national efforts to redesign biology curricula—the Advanced Placement (AP) Biology Curriculum Revision (College Board 2015), Vision and Change (American Association for the Advancement of Science 2011), and the Next Generation Science Standards for K–12 (National Research Council 2011)—largely agree on the “big ideas,” the core areas (e.g., information storage and transmission) in which all biology students should gain conceptual mastery (Table 3). More specialized efforts by several professional societies have sought to identify the most important core concepts within subdisciplines such as genetics. The GSA has developed a Genetics Learning Framework for the use of genetics instructors, which is posted on the websites of the GSA and the teaching journal CourseSource. The framework consists of core categories, core concepts, and more specific course learning goals that instructors can use in place of a standard syllabus (Figure 2).
Placement of genetics concepts in national efforts to redesign biology curricula
. | AP Biology curriculum revision (College Board 2015) . | Vision and change (American Association for the Advancement of Science 2011) . | Next generation science standards for K–12 (National Research Council 2011) . |
---|---|---|---|
Description of genetics concepts | Living systems store, retrieve, transmit, and respond to information essential to life processes. | Information flow, exchange, and storage: the growth and behavior of organisms are activated through the expression of genetic information in context. | Heredity: inheritance and variation of traits. |
Organization of information | One of four “Big Ideas” in the AP Biology course curriculum. | One of the five core concepts that all undergraduate biology students should know about. | One of the four core and component ideas in the life sciences. |
. | AP Biology curriculum revision (College Board 2015) . | Vision and change (American Association for the Advancement of Science 2011) . | Next generation science standards for K–12 (National Research Council 2011) . |
---|---|---|---|
Description of genetics concepts | Living systems store, retrieve, transmit, and respond to information essential to life processes. | Information flow, exchange, and storage: the growth and behavior of organisms are activated through the expression of genetic information in context. | Heredity: inheritance and variation of traits. |
Organization of information | One of four “Big Ideas” in the AP Biology course curriculum. | One of the five core concepts that all undergraduate biology students should know about. | One of the four core and component ideas in the life sciences. |
. | AP Biology curriculum revision (College Board 2015) . | Vision and change (American Association for the Advancement of Science 2011) . | Next generation science standards for K–12 (National Research Council 2011) . |
---|---|---|---|
Description of genetics concepts | Living systems store, retrieve, transmit, and respond to information essential to life processes. | Information flow, exchange, and storage: the growth and behavior of organisms are activated through the expression of genetic information in context. | Heredity: inheritance and variation of traits. |
Organization of information | One of four “Big Ideas” in the AP Biology course curriculum. | One of the five core concepts that all undergraduate biology students should know about. | One of the four core and component ideas in the life sciences. |
. | AP Biology curriculum revision (College Board 2015) . | Vision and change (American Association for the Advancement of Science 2011) . | Next generation science standards for K–12 (National Research Council 2011) . |
---|---|---|---|
Description of genetics concepts | Living systems store, retrieve, transmit, and respond to information essential to life processes. | Information flow, exchange, and storage: the growth and behavior of organisms are activated through the expression of genetic information in context. | Heredity: inheritance and variation of traits. |
Organization of information | One of four “Big Ideas” in the AP Biology course curriculum. | One of the five core concepts that all undergraduate biology students should know about. | One of the four core and component ideas in the life sciences. |
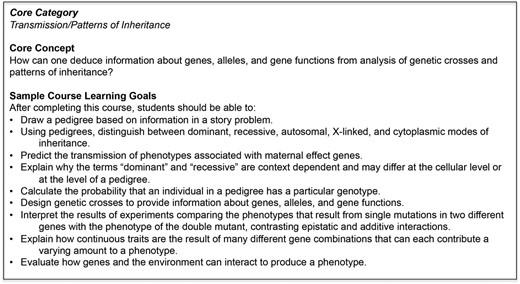
Examples of a core category, a core concept, and course learning goals from the GSA Genetics Learning Framework, intended to help instructors design introductory-level genetics courses.
All three of the major national efforts also emphasize the need to teach students about the practice of science, not just its content. One effective way to meet this need is through Classroom Undergraduate Research Experiences (CUREs), also called discovery-based lab courses, in which groups of students engage in authentic research on an unsolved problem (e.g., Hatfull et al. 2006; Lopatto et al. 2014). In the future, it may be that all genetics students will be required to take at least one such course during their undergraduate career.
How will we know if students have learned and retained what we teach?
Various stakeholders, including university administrators, graduate and professional schools, prospective employers, and others, are increasingly likely to demand accountability from undergraduate programs, in the form of objective evidence regarding what graduating students know about their major field. In biology, departments currently have only a few tools at their disposal to assess this knowledge, such as the Majors Field Tests, the Graduate Record Exams, the Medical College Admission Test (MCAT), and various concept inventories (Table 2). However, these tests must be machine graded to be practical for administration to large numbers of students nationally, and with current technology they are therefore constrained to consist mainly of multiple-choice questions.
Evaluating deeper conceptual knowledge and authentic scientific practices in genetics requires richer forms of assessment. Geneticists display their knowledge by constructing explanations and arguments, not merely selecting from a set of possible answers. Constructed-response assessments, in which students must analyze or model genetic phenomena in response to an open-ended question, reveal how students combine correct and incorrect ideas. Moreover, students use better study strategies and acquire deeper learning in preparation for constructed-response assessments than for multiple-choice exams (Stanger-Hall 2012). Such exams could provide the information that departments need, but their large-scale use will require improvement of computer programs that can evaluate student written responses (Ha et al. 2011; Haudek et al. 2011; Urban-Lurain et al. 2015). Eventually, it would also be valuable to develop mechanisms for assessing program graduates in subsequent years, so that we could measure their retention of genetic conceptual knowledge, the factors that affect retention, and how useful their education was for their careers and their roles in society.
Acknowledgments
We thank members of the Society for the Advancement of Biology Education Research (SABER) listserv for providing helpful information about teaching genetics 100 years ago. We especially thank Marvin O’Neal and Keith Sheppard from Stony Brook University, and Marisa Méndez-Brady from the University of Maine for going out of their way to help us find historical resources. Thank you also to Ken Akiha, Karen Pelletreau, Mindi Summers, Elizabeth Trenckmann, Mark Urban-Lurain, and Erin Vinson for helpful comments on the manuscript.
Footnotes
Communicating editor: M. Johnston
Literature Cited
Brockliss, L., 1996 Curricula. A History of the University in Europe, edited by H. de Ridder-Symoens. Cambridge University Press, Cambridge, UK, Vol II, pp. 565–620.
Chen, X., 2013 STEM Attrition: College Students’ Paths into and out of STEM Fields. Available at: http://nces.ed.gov/pubs2014/2014001rev.pdf. Accessed June 1, 2016.
College Board, 2015 AP Biology Course and Exam Description, Revised 2015. College Board, New York, NY. Available at: https://secure-media.collegeboard.org/digitalServices/pdf/ap/ap-biology-course-and-exam-description.pdf. Accessed July 27, 2016.
Eagan, M. K., E. B. Stolzenberg, J. Berdan Lozano, M. C. Aragon, M. R. Suchard et al., 2014 Undergraduate teaching faculty: The 2013–2014 HERI Faculty Survey. Los Angeles Higher Education Research Institute, UCLA, Los Angeles.
Hollister, P. L., 1939 Development of the teaching of introductory biology in American colleges. Ph.D. Thesis, George Peabody College for Teachers, Nashville, TN.