-
PDF
- Split View
-
Views
-
Cite
Cite
Yi Yi Yong, Michelle Wei Kim Ong, Gary Dykes, Wee Sim Choo, Betacyanin-inhibited biofilm formation of co-culture of Staphylococcus aureus and Pseudomonas aeruginosa on different polymer surfaces, FEMS Microbiology Letters, Volume 368, Issue 1, January 2021, fnaa214, https://doi.org/10.1093/femsle/fnaa214
- Share Icon Share
ABSTRACT
Staphylococcus aureus and Pseudomonas aeruginosa are bacteria that cause biofilm-associated infections. The aim of this study was to determine the activity of combined betacyanin fractions from Amaranthus dubius (red spinach) and Hylocereus polyrhizus (red pitahaya) against biofilms formed by co-culture of S. aureus and P. aeruginosa on different polymer surfaces. Various formulations containing different concentrations of the betacyanin fractions were investigated for biofilm-inhibiting activity on polystyrene surfaces using crystal violet assay and scanning electron microscopy. A combination of each betacyanin fraction (0.625 mg mL−1) reduced biofilm formation of five S. aureus strains and four P. aeruginosa strains from optical density values of 1.24–3.84 and 1.25–3.52 to 0.81–2.63 and 0.80–1.71, respectively. These combined fractions also significantly inhibited dual-species biofilms by 2.30 and reduced 1.0–1.3 log CFU cm−2 bacterial attachment on polymer surfaces such as polyvinyl chloride, polyethylene, polypropylene and silicone rubber. This study demonstrated an increase in biofilm-inhibiting activity against biofilms formed by two species using combined fractions than that by using single fractions. Betacyanins found in different plants could collectively be used to potentially decrease the risk of biofilm-associated infections caused by these bacteria on hydrophobic polymers.
INTRODUCTION
Bacteria evolved with a preference to live in biofilms—which are highly complex sessile communities—rather than planktonic cells, to protect themselves from harsh environments and damage from antimicrobials (Bjarnsholt et al. 2013). This feature is ubiquitous in nature and any surface that is exposed to naturally occurring fluids can be colonized by bacteria and a biofilm may form. Biofilm formation has been responsible for many persistent or chronic bacterial infections as they are resistant to removal by antimicrobials, host defense mechanisms and even physical cleaning processes. Several mechanisms that contribute to the resistance of biofilms to antimicrobial agents are different from those that contribute to the resistance of planktonic bacteria to the same agents. Some of these resistance mechanisms can contribute together, resulting in an increase in resistance of biofilms (Yong et al. 2019). The occurrence of biofilms on the surfaces of medical implants is frequent and a common way to solve the issue is the replacement of the medical implants and prolonged administration of antibiotics (Olivares et al. 2020).
Regardless of the specific variations in strain and niche that can influence the complex interactions that may occur within mono or multispecies biofilm, the initial steps allowing bacteria to attach and adhere to a surface appear to be universal (Klemm and Schembri 2004). Bacterial adhesion is influenced by parameters such as environmental conditions and cell surface properties of the bacteria (Katsikogianni and Missirlis 2004). A biofilm can be formed by single species of bacteria or more commonly by several different species of bacteria. Pseudomonas aeruginosa and Staphylococcus aureus can coexist in multiple infection settings, and both form biofilms that can be difficult to eradicate. They are often co-isolated from chronic wound infection and cystic fibrosis (Serra et al. 2015; Limoli and Hoffman 2019). They therefore should be co-cultured in vitro to better investigate the effect of potential therapeutics.
Synthetic polymers are widely used in the biomedical field to make into medical devices or implants because they are light in weight, show good biocompatibility and flexibility and have a low cost (Maitz 2015). Biofilms are commonly found in catheters (urinary and central venous), endotracheal tubes and implants (pacemakers, heart valves, prosthetic joints and orthopedic implants) that are made of synthetic polymers. Biofilms on these devices transmit bacteria and act as a source of infection. This can undermine the functionality and safety of the device and the health status of individuals (Muñoz-Bonilla and Fernández-García 2012). When a biomaterial is placed in vivo for some time a biofilm may start to form due to the presence of dead cells, conditioning layers of human proteins and the presence of persister cells after antibiotic treatment (Khatoon et al. 2018). Materials from different categories, namely, polypropylene, polyethylene, silicone rubber and polyvinyl chloride, all allow biofilm formation. These materials lack polar groups and tend to attract hydrophobic bacteria to different extents compared with hydrophilic material such as glass (Krasowska and Sigler 2014).
Betalains, specifically betacyanins, are red color pigments that are present in edible plants such as beetroot, cactus fruits and amaranth plants (Choo 2018). This family of compounds possess various biological properties such as antimicrobial, anti-lipidemic, antioxidant and anticancer (Gengatharan, Dykes and Choo 2015). Betanin from beetroot was recently found to enhance the survival of Caenorhabditis elegans infected with methicillin-resistant S. aureus (Choo, Wong and Nathan 2020). We have previously reported that a single betacyanin fraction from Amaranthus dubius and Hylocereus polyrhizus demonstrated biofilm-inhibiting activity against a single culture of S. aureus and P. aeruginosa biofilms on a polystyrene surface (Yong et al. 2019). The objective of this research was to determine the biofilm-inhibiting activity of combined betacyanin fractions from A. dubius and H. polyrhizus against co-culture of S. aureus and P. aeruginosa on different polymer surfaces.
MATERIALS AND METHODS
Preparation and characterization of betacyanin fractions
Red pitahaya or red dragon (H. polyrhizus) fruit and red spinach (A. dubius) leaves were bought from a local supermarket and wet market, respectively, in Selangor, Malaysia. Preparation of betacyanin fractions was conducted following the method of Yong et al. (2017). Extraction was carried out in a blender (Waring, Stamford, U.S.A.) with a substrate to solvent (chilled 70% methanol) ratio of 1:4. Next, centrifugation at 2000 × g at 4°C was carried out for 10 min. Filtration of the supernatant was conducted followed by rotary evaporation of the filtrates at 37°C. Lastly, freeze drying at −80°C was carried out. Sub-fractionation of the freeze-dried extract was carried out by dissolving the extract in water and the pH was adjusted to 2. Extraction was carried out with ethyl acetate (v/v: 1:1) three times in an extraction flask. The aqueous phase was collected then underwent rotary evaporation at 37°C followed by freeze-drying to produce the betacyanin fraction. The betacyanin fractions were stored at −80°C until use. High performance liquid chromatography (HPLC) analysis was used to determine the betacyanin composition and performed using an Agilent 1260 Infinity HPLC system from Agilent Technologies (Santa Clara, USA). The photodiode array detector was set at 538 nm. A LiChroCART® Purospher STAR C-18 column from Merck (Darmstadt, Germany) was used. The injection volume was 20 μL. Elution at a flow rate of 1 mL min−1 was conducted using 90% of 0.5% aqueous trifluoroacetic acid and 10% acetonitrile for 70 min.
Inhibition of biofilm formation by combined betacyanin fractions
Combinations of betacyanin fraction were tested in triplicate against five strains of S. aureus and four strains of P. aeruginosa from the American Type Culture Collection (Manassas, USA). The concentration of each betacyanin fraction in the combined fractions ranged from 0.313 mg mL−1 to 2.5 mg mL−1. The matrix of combinations used are presented in the x-axes of Figs 1 and 2. To evaluate the interaction of betacyanin fractions, the fractional inhibitory concentration (FIC) index of biofilm formation was calculated using the minimum biofilm inhibition concentration (MBIC). The FIC model was expressed as: ΣFIC = FICred pitahaya + FICred spinach, where FICred pitahaya = MBIC of betacyanin fraction formulation/MBIC of betacyanin fraction from red pitahaya and FICred spinach = MBIC of betacyanin fraction formulation/MBIC of betacyanin fraction from red spinach. Synergism is apparent if the value of ΣFIC ≤ 0.5 and antagonism for ΣFIC > 4. A ΣFIC > 0.5 while a ΣFIC ≤ 4 is considered as a no interaction (Odds 2003).
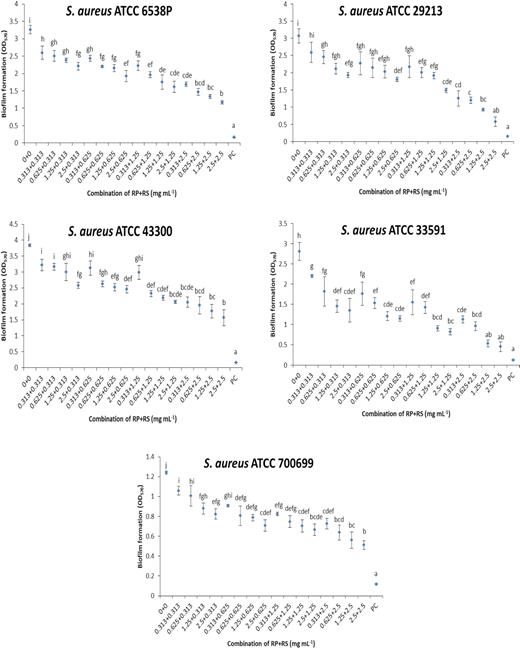
Effect of combination of betacyanin fractions from red pitahaya (RP) and red spinach (RS) at different concentrations against biofilm formation of S. aureus on a polystyrene surface. Different lowercase letters indicate significant difference in biofilm formation at P < 0.05. PC: positive control (sodium hypochlorite was used).
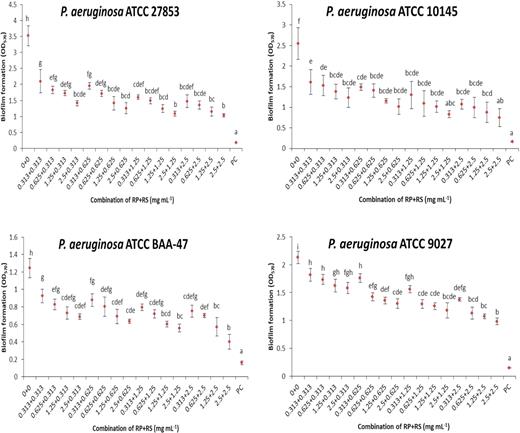
Effect of combination of betacyanin fractions from red pitahaya (RP) and red spinach (RS) at different concentrations against biofilm formation of P. aeruginosa on a polystyrene surface. Different lowercase letters indicate significant difference in biofilm formation at P < 0.05. PC: positive control (sodium hypochlorite was used).
Bacteria were first grown in brain heart infusion (BHI) broth (10 mL) at 37°C for 18 h. This bacterial suspension was then adjusted to a 0.5 McFarland standard with absorbance readings between 0.08 and 0.10 at 625 nm. This was equivalent to ~1 × 108 CFU mL−1. A 1:100 dilution was carried out with BHI broth plus 1% glucose to promote biofilm formation in vitro (Regassa, Novick and Betley 1992). Next, 180 μL of the bacterial suspensions were placed in a flat-bottomed 96-well polystyrene microtiter plate. Next, 20 μL of sterilized sample with a 0.20 μm polyethersulfone syringe filter was added. After 24 h at 37°C, the plate was inverted and the content in each well was rinsed three times with water to remove planktonic cells. Crystal violet assay was carried out according to the method of Stepanović et al. (2000). The plates were heat-fixed at 60°C for 1 h. The fixed biofilm was stained with 200 μL of 0.1% crystal violet stain for 15 min. Excess stain was washed three times with water then air-dried. Application of methanol was conducted to dissolve color to allow for absorbance measurement at 570 nm. Bacterial suspension plus distilled water only was the negative control whereas bacterial suspension plus sodium hypochlorite was the positive control.
The most effective betacyanin fraction formulation was tested on biofilm formed by co-culture of S. aureus (ATCC 6538P) and P. aeruginosa (ATCC 27853) on different polymers. Polymer tubes (1 m in length × 1 cm in diameter) of polyvinyl chloride, polypropylene, polyethylene and silicone rubber were purchased from a hardware shop (Selangor, Malaysia). Polymer tubes were cut to a length of 1 cm and cleaned with 70% ethanol followed by rinsing with water. Biofilm formation was achieved according to the method described above in universal bottles (10 mL) containing the sterilized tubes. After incubation for a duration of 24 h at 37°C, the tubes were removed from the respective universal bottles and rinsed gently with water prior to heat fixation. The tubes were then stained with crystal violet and the color was then dissolved in methanol with 2 min sonication. Bacterial cell surface hydrophobicity was determined following the method of Yong et al. (2019) using a bacterial adherence to hydrocarbon assay.
Attachment to different polymer surfaces
The ability of the betacyanin fraction formulation to affect the attachment of bacteria to a surface was compared with a negative control. Polymer coupons (10 cm × 10 cm) of polyvinyl chloride, polypropylene, polyethylene and silicone rubber were purchased from a local hardware shop. Both the bacterial suspension (optical density [OD]550 ∼1) and the betacyanin fraction were added to a six-well polystyrene microtiter plate in which a polymer coupon (2 cm × 2 cm) was inserted to allow cells to attach to its surface. After incubation at 37°C for 1 h without shaking, the coupon was then removed and gently rinsed three times with phosphate buffered saline (PBS) for the purpose of removing bacterial cells that were loosely attached to the coupon. The coupon was stained with 0.1% (w/v) crystal violet or 0.01% (w/v) acridine orange. The number of cells (log CFU cm−2) on the coupon was counted with 30 fields of view in total using light microscopy or fluorescence microscopy.
Scanning electron microscopy
The morphological effect of the combined fractions on biofilm formation on different polymer surfaces was determined using a S-3400N VP scanning electron microscope (Hitachi, Tokyo, Japan). Biofilm cell density and distribution were examined. Bacterial cell numbers were estimated by eye in triplicate on the coupon images and tabulated into log CFU cm−2 based on the morphological differences between S. aureus and P. aeruginosa. Staphylococcus aureus is cocci-shaped while P. aeruginosa is rod-shaped. Sterile polymer coupons (1 cm × 1 cm) were placed in 24-well plates containing standardized bacterial suspension and betacyanin fraction formulation. The plate was incubated at 37°C for a duration of 24 h. After incubation, the coupon was washed with PBS. Next, the preparation of the coupon for scanning electron microscopy (SEM) was conducted by soaking it in 2.5% (v/v) glutaraldehyde at room temperature for a duration of 2 h before washing with PBS. Dehydration was conducted using 50%, 60%, 70%, 80%, 90% and 100% ethanol sequentially for 10 min each time. This process was repeated three times. The coupons were then stored in a desiccator filled with silica gels. Before examining the coupons using SEM, the surface was gold-sputtered for 10 min using a sputter coater.
Statistical analysis
Independent triplicates of all experiments were carried out. Data were analyzed using one-way ANOVA followed by post-hoc Tukey's test. Significance at P < 0.05 was evaluated using IBM SPSS 20 software.
RESULTS
Determination of the most effective betacyanin fraction formulation
A range of combinations of betacyanin fractions were tested against biofilms of S. aureus strains (Fig. 1) and P. aeruginosa strains (Fig. 2). These concentrations were selected as it was shown in the previous study by Yong et al. (2019) that the single betacyanin fractions at these concentrations did not affect the bacterial cell viability during a 24-h growth assay. The strains of S. aureus tested typically produce biofilm ranging from low density (S. aureus ATCC 700699 and ATCC 29213) to high density (S. aureus ATCC 6538P and ATCC 43300). Methicillin-resistant bacteria (S. aureus ATCC 43300, ATCC 700699 and ATCC 33591) were also tested. The strains of P. aeruginosa tested were moderate biofilm formers (ATCC 27853 and ATCC 9027) to weak biofilm formers (ATCC 10145 and ATCC BAA-47). According to Darabpour et al. (2017), bacteria are classified as strong biofilm producer when the OD at 570 nm > 4. Moderate and weak biofilm producers show 2 ≤ OD570 ≤ 4 and OD570 < 2, respectively. Using crystal violet assay, the formulation that combined betacyanin fractions (0.625 mg mL−1 each) reduced 0.44‒0.93 of biofilm formation by S. aureus strains (ATCC 700699, ATCC 29213) and 1.1‒1.3 for S. aureus ATCC 6538P, ATCC 43300 and ATCC 33591 (Fig. 1). There was no significant trend in the inhibitory activity of betacyanin fraction formulations against S. aureus in terms of methicillin sensitivity and ability to produce biofilms. Combined betacyanin fractions (0.625 mg mL−1 each) reduced 0.44‒1.14 of biofilm formation by P. aeruginosa strains (ATCC 9027, ATCC 10145 and ATCC BAA-47) and 1.8 of biofilm formation by P. aeruginosa ATCC 27853 (Fig. 2). These biofilm inhibitory activities showed a strain-specific effect. The combination of the lowest concentration of the betacyanin fraction (0.313 mg mL−1) significantly reduced biofilm formation (P < 0.05) for all strains except S. aureus ATCC 29213 (Figs 1 and 2). The anti-biofilm effect of the combined betacyanin fraction increased with increasing concentrations. The combination of 0.625 mg mL−1 of each betacyanin fraction significantly reduced biofilm formation (P < 0.05) of all strains of S. aureus and P. aeruginosa (Figs 1 and 2).
Effect of betacyanin formulation on a dual-species biofilm
A dual-species biofilm was formed on the polystyrene surface by culturing S. aureus (ATCC 6538P) and P. aeruginosa (ATCC 27853) together (Fig. 3). The strains were established in initial studies not to inhibit each other (Supplementary Table 1). The co-culture was stable as there was no cell death measured by the viable cell count (Wijesinghe et al. 2019). When grown together, these strains formed more biofilm, as indicated by the higher absorbance reading in crystal violet assay compared with when grown individually. Using crystal violet assay, the betacyanin fraction formulation reduced biofilm formation of co-culture of S. aureus and P. aeruginosa by 2.30 compared with that of the single betacyanin fractions (1.25 mg mL−1), which reduced 1.75‒1.86 of biofilm formation (Table 1). Our previous study showed that the composition of the single betacyanin fraction from red pitahaya was 45% phyllocactin, 39% betanin, 11% hylocerenin and 5% isobetanin whereas the composition of the single betacyanin fraction from red spinach was 79% amaranthine, 19% decarboxy-amaranthine and 2% betanin (Yong et al. 2017). HPLC analysis of the betacyanin formulation consisting of the combination of 0.625 mg mL−1 of each betacyanin fraction contained 23.6 ± 0.1% betanin, 6.4 ± 0.1% isobetanin, 25.5 ± 0.4% phyllocactin, 6.7 ± 0.4% hylocerenin, 29.6 ± 0.6% amaranthine and 8.4 ± 0.2% decarboxy-amaranthine. As a result of the treatment with this betacyanin formulation, the microbial hydrophobicity was significantly reduced (P < 0.05) by 37.5% from 67.0% to 29.5%, whereas the attachment to polystyrene was significantly reduced (P < 0.05) by 0.6 log CFU cm−2 (Supplementary Table 2). The cell morphology of the S. aureus and P. aeruginosa was, however, not affected by the betacyanin fraction formulation (Fig. 3).
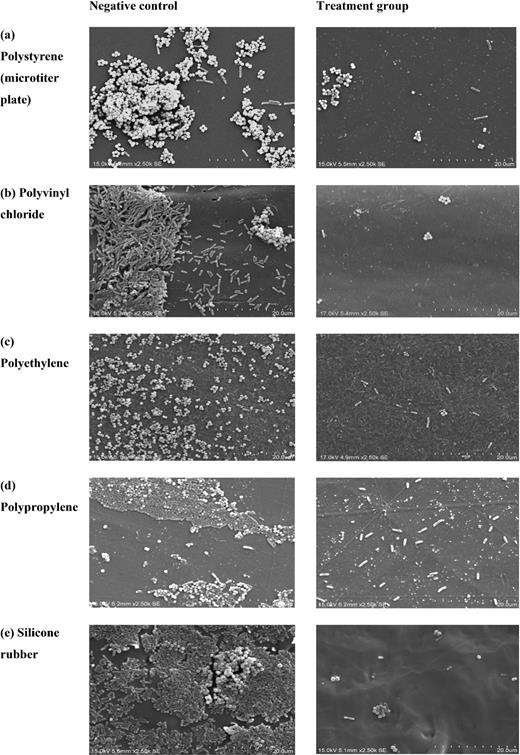
SEM images of dual-species biofilm of S. aureus ATCC 6538P and P. aeruginosa ATCC 27853 on various polymer surfaces (a‒e): negative control (left); treatment with betacyanin fraction formulation (0.625 mg/mL RP + 0.625 mg/mL RS) (right). Scale bars = 20 μm.
Effect of betacyanin fraction formulation on biofilm formation of single or dual-species of S. aureus and P. aeruginosa on a polystyrene surface.
. | Biofilm formation (OD570) . | ||
---|---|---|---|
Sample (mg mL−1) . | S. aureus ATCC 6538P . | P. aeruginosa ATCC 27853 . | S. aureus ATCC 6538P and P. aeruginosa ATCC 27853 . |
Negative control | 3.76 ± 0.45b | 1.21 ± 0.30c | 5.76 ± 0.30c |
0.625 (RP) + 0.625 (RS) | 2.54 ± 0.05a | 0.59 ± 0.03a | 3.47 ± 0.16a |
1.25 (RP) | 2.49 ± 0.29a | 0.81 ± 0.12b | 4.01 ± 0.24ab |
1.25 (RS) | 2.42 ± 0.24a | 0.94 ± 0.13b | 3.91 ± 0.18bc |
. | Biofilm formation (OD570) . | ||
---|---|---|---|
Sample (mg mL−1) . | S. aureus ATCC 6538P . | P. aeruginosa ATCC 27853 . | S. aureus ATCC 6538P and P. aeruginosa ATCC 27853 . |
Negative control | 3.76 ± 0.45b | 1.21 ± 0.30c | 5.76 ± 0.30c |
0.625 (RP) + 0.625 (RS) | 2.54 ± 0.05a | 0.59 ± 0.03a | 3.47 ± 0.16a |
1.25 (RP) | 2.49 ± 0.29a | 0.81 ± 0.12b | 4.01 ± 0.24ab |
1.25 (RS) | 2.42 ± 0.24a | 0.94 ± 0.13b | 3.91 ± 0.18bc |
Different lowercase letters within the same column indicate significant difference in biofilm formation at P < 0.05. RP: red pitahaya; RS: red spinach.
Effect of betacyanin fraction formulation on biofilm formation of single or dual-species of S. aureus and P. aeruginosa on a polystyrene surface.
. | Biofilm formation (OD570) . | ||
---|---|---|---|
Sample (mg mL−1) . | S. aureus ATCC 6538P . | P. aeruginosa ATCC 27853 . | S. aureus ATCC 6538P and P. aeruginosa ATCC 27853 . |
Negative control | 3.76 ± 0.45b | 1.21 ± 0.30c | 5.76 ± 0.30c |
0.625 (RP) + 0.625 (RS) | 2.54 ± 0.05a | 0.59 ± 0.03a | 3.47 ± 0.16a |
1.25 (RP) | 2.49 ± 0.29a | 0.81 ± 0.12b | 4.01 ± 0.24ab |
1.25 (RS) | 2.42 ± 0.24a | 0.94 ± 0.13b | 3.91 ± 0.18bc |
. | Biofilm formation (OD570) . | ||
---|---|---|---|
Sample (mg mL−1) . | S. aureus ATCC 6538P . | P. aeruginosa ATCC 27853 . | S. aureus ATCC 6538P and P. aeruginosa ATCC 27853 . |
Negative control | 3.76 ± 0.45b | 1.21 ± 0.30c | 5.76 ± 0.30c |
0.625 (RP) + 0.625 (RS) | 2.54 ± 0.05a | 0.59 ± 0.03a | 3.47 ± 0.16a |
1.25 (RP) | 2.49 ± 0.29a | 0.81 ± 0.12b | 4.01 ± 0.24ab |
1.25 (RS) | 2.42 ± 0.24a | 0.94 ± 0.13b | 3.91 ± 0.18bc |
Different lowercase letters within the same column indicate significant difference in biofilm formation at P < 0.05. RP: red pitahaya; RS: red spinach.
Effect of betacyanin fraction formulation on biofilm formation and bacterial attachment to different polymers
Using crystal violet assay, the betacyanin fraction formulation significantly reduced (P < 0.05) dual-species biofilm formation by 0.95–1.78 when tested on different polymer surfaces (Table 2). A water contact angle analysis was conducted on the polymers and it was established that the contact angle for polyvinyl chloride (95°) < polyethylene (102°) < polypropylene (107°) < silicone rubber (122°). Silicone rubber with the highest water contact angle is most hydrophobic due to the presence of silicones. Biofilm inhibition and reduction in cell attachment on the silicone rubber surface was the highest, a result which is also supported by the estimated cell count (Table 3) based on SEM images. The number of S. aureus and P. aeruginosa were significantly reduced (P < 0.05) for all polymers after treatment with the betacyanin fraction formulations except for P. aeruginosa on polyvinyl chloride surfaces (Table 3).
Effect of betacyanin fraction formulation on biofilm formation and cell attachment of dual-species of S. aureus and P. aeruginosa on different types of polymer.
. | Biofilm formation (OD570) . | Cell attachment (log CFU cm−2) . | ||
---|---|---|---|---|
Type of polymer . | Negative control . | 0.625 mg mL−1 RP + 0.625 mg mL−1 RS . | Negative control . | 0.625 mg mL−1 RP + 0.625 mg mL−1 RS . |
Polyvinyl chloride | 1.52 ± 0.22b | 0.56 ± 0.18a | 7.75 ± 0.05B | 6.62 ± 0.05A |
Polyethylene | 1.71 ± 0.18b | 0.61 ± 0.10a | 7.71 ± 0.06B | 6.73 ± 0.07A |
Polypropylene | 1.75 ± 0.20b | 0.46 ± 0.08a | 7.73 ± 0.02B | 6.66 ± 0.08A |
Silicone rubber | 2.28 ± 0.21b | 0.50 ± 0.04a | 7.87 ± 0.02B | 6.57 ± 0.04A |
. | Biofilm formation (OD570) . | Cell attachment (log CFU cm−2) . | ||
---|---|---|---|---|
Type of polymer . | Negative control . | 0.625 mg mL−1 RP + 0.625 mg mL−1 RS . | Negative control . | 0.625 mg mL−1 RP + 0.625 mg mL−1 RS . |
Polyvinyl chloride | 1.52 ± 0.22b | 0.56 ± 0.18a | 7.75 ± 0.05B | 6.62 ± 0.05A |
Polyethylene | 1.71 ± 0.18b | 0.61 ± 0.10a | 7.71 ± 0.06B | 6.73 ± 0.07A |
Polypropylene | 1.75 ± 0.20b | 0.46 ± 0.08a | 7.73 ± 0.02B | 6.66 ± 0.08A |
Silicone rubber | 2.28 ± 0.21b | 0.50 ± 0.04a | 7.87 ± 0.02B | 6.57 ± 0.04A |
Different letters within the row indicate significant difference at P < 0.05 in biofilm formation.
Different letters within the row indicate significant difference at P < 0.05 in cell attachment. RP: red pitahaya; RS: red spinach.
Effect of betacyanin fraction formulation on biofilm formation and cell attachment of dual-species of S. aureus and P. aeruginosa on different types of polymer.
. | Biofilm formation (OD570) . | Cell attachment (log CFU cm−2) . | ||
---|---|---|---|---|
Type of polymer . | Negative control . | 0.625 mg mL−1 RP + 0.625 mg mL−1 RS . | Negative control . | 0.625 mg mL−1 RP + 0.625 mg mL−1 RS . |
Polyvinyl chloride | 1.52 ± 0.22b | 0.56 ± 0.18a | 7.75 ± 0.05B | 6.62 ± 0.05A |
Polyethylene | 1.71 ± 0.18b | 0.61 ± 0.10a | 7.71 ± 0.06B | 6.73 ± 0.07A |
Polypropylene | 1.75 ± 0.20b | 0.46 ± 0.08a | 7.73 ± 0.02B | 6.66 ± 0.08A |
Silicone rubber | 2.28 ± 0.21b | 0.50 ± 0.04a | 7.87 ± 0.02B | 6.57 ± 0.04A |
. | Biofilm formation (OD570) . | Cell attachment (log CFU cm−2) . | ||
---|---|---|---|---|
Type of polymer . | Negative control . | 0.625 mg mL−1 RP + 0.625 mg mL−1 RS . | Negative control . | 0.625 mg mL−1 RP + 0.625 mg mL−1 RS . |
Polyvinyl chloride | 1.52 ± 0.22b | 0.56 ± 0.18a | 7.75 ± 0.05B | 6.62 ± 0.05A |
Polyethylene | 1.71 ± 0.18b | 0.61 ± 0.10a | 7.71 ± 0.06B | 6.73 ± 0.07A |
Polypropylene | 1.75 ± 0.20b | 0.46 ± 0.08a | 7.73 ± 0.02B | 6.66 ± 0.08A |
Silicone rubber | 2.28 ± 0.21b | 0.50 ± 0.04a | 7.87 ± 0.02B | 6.57 ± 0.04A |
Different letters within the row indicate significant difference at P < 0.05 in biofilm formation.
Different letters within the row indicate significant difference at P < 0.05 in cell attachment. RP: red pitahaya; RS: red spinach.
Effect of betacyanin fraction formulation on the number of bacterial cells on SEM images of different types of polymer.
. | . | log CFU cm−2 . | . | . | . |
---|---|---|---|---|---|
Polymer . | Species . | Negative control . | Ratio SA/PA . | Treatment . | Ratio SA/PA . |
Polystyrene | S. aureus | 7.82 ± 0.10b | 1.12 | 6.59 ± 0.09a | 1.11 |
P. aeruginosa | 6.97 ± 0.07b | 5.92 ± 0.34a | |||
Polyvinyl chloride | S. aureus | 7.44 ± 0.23b | 0.99 | 6.24 ± 0.20a | 1.17 |
P. aeruginosa | 7.52 ± 0.50a | 5.33 ± 0.15a | |||
Polyethylene | S.aureus | 7.88 ± 0.11b | 1.21 | 6.03 ± 0.16a | 1.02 |
P.aeruginosa | 6.49 ± 0.11b | 5.89 ± 0.09a | |||
Polypropylene | S. aureus | 7.91 ± 0.07b | 1.21 | 6.09 ± 0.08a | 1.01 |
P. aeruginosa | 6.54 ± 0.14b | 6.02 ± 0.29a | |||
Silicone rubber | S. aureus | 7.21 ± 0.23b | 0.91 | 6.05 ± 0.37a | 1.01 |
P. aeruginosa | 7.87 ± 0.14b | 5.98 ± 0.15a |
. | . | log CFU cm−2 . | . | . | . |
---|---|---|---|---|---|
Polymer . | Species . | Negative control . | Ratio SA/PA . | Treatment . | Ratio SA/PA . |
Polystyrene | S. aureus | 7.82 ± 0.10b | 1.12 | 6.59 ± 0.09a | 1.11 |
P. aeruginosa | 6.97 ± 0.07b | 5.92 ± 0.34a | |||
Polyvinyl chloride | S. aureus | 7.44 ± 0.23b | 0.99 | 6.24 ± 0.20a | 1.17 |
P. aeruginosa | 7.52 ± 0.50a | 5.33 ± 0.15a | |||
Polyethylene | S.aureus | 7.88 ± 0.11b | 1.21 | 6.03 ± 0.16a | 1.02 |
P.aeruginosa | 6.49 ± 0.11b | 5.89 ± 0.09a | |||
Polypropylene | S. aureus | 7.91 ± 0.07b | 1.21 | 6.09 ± 0.08a | 1.01 |
P. aeruginosa | 6.54 ± 0.14b | 6.02 ± 0.29a | |||
Silicone rubber | S. aureus | 7.21 ± 0.23b | 0.91 | 6.05 ± 0.37a | 1.01 |
P. aeruginosa | 7.87 ± 0.14b | 5.98 ± 0.15a |
Different letters within the row indicate significant difference at P < 0.05. SA: S. aureus; PA: P. aeruginosa.
Effect of betacyanin fraction formulation on the number of bacterial cells on SEM images of different types of polymer.
. | . | log CFU cm−2 . | . | . | . |
---|---|---|---|---|---|
Polymer . | Species . | Negative control . | Ratio SA/PA . | Treatment . | Ratio SA/PA . |
Polystyrene | S. aureus | 7.82 ± 0.10b | 1.12 | 6.59 ± 0.09a | 1.11 |
P. aeruginosa | 6.97 ± 0.07b | 5.92 ± 0.34a | |||
Polyvinyl chloride | S. aureus | 7.44 ± 0.23b | 0.99 | 6.24 ± 0.20a | 1.17 |
P. aeruginosa | 7.52 ± 0.50a | 5.33 ± 0.15a | |||
Polyethylene | S.aureus | 7.88 ± 0.11b | 1.21 | 6.03 ± 0.16a | 1.02 |
P.aeruginosa | 6.49 ± 0.11b | 5.89 ± 0.09a | |||
Polypropylene | S. aureus | 7.91 ± 0.07b | 1.21 | 6.09 ± 0.08a | 1.01 |
P. aeruginosa | 6.54 ± 0.14b | 6.02 ± 0.29a | |||
Silicone rubber | S. aureus | 7.21 ± 0.23b | 0.91 | 6.05 ± 0.37a | 1.01 |
P. aeruginosa | 7.87 ± 0.14b | 5.98 ± 0.15a |
. | . | log CFU cm−2 . | . | . | . |
---|---|---|---|---|---|
Polymer . | Species . | Negative control . | Ratio SA/PA . | Treatment . | Ratio SA/PA . |
Polystyrene | S. aureus | 7.82 ± 0.10b | 1.12 | 6.59 ± 0.09a | 1.11 |
P. aeruginosa | 6.97 ± 0.07b | 5.92 ± 0.34a | |||
Polyvinyl chloride | S. aureus | 7.44 ± 0.23b | 0.99 | 6.24 ± 0.20a | 1.17 |
P. aeruginosa | 7.52 ± 0.50a | 5.33 ± 0.15a | |||
Polyethylene | S.aureus | 7.88 ± 0.11b | 1.21 | 6.03 ± 0.16a | 1.02 |
P.aeruginosa | 6.49 ± 0.11b | 5.89 ± 0.09a | |||
Polypropylene | S. aureus | 7.91 ± 0.07b | 1.21 | 6.09 ± 0.08a | 1.01 |
P. aeruginosa | 6.54 ± 0.14b | 6.02 ± 0.29a | |||
Silicone rubber | S. aureus | 7.21 ± 0.23b | 0.91 | 6.05 ± 0.37a | 1.01 |
P. aeruginosa | 7.87 ± 0.14b | 5.98 ± 0.15a |
Different letters within the row indicate significant difference at P < 0.05. SA: S. aureus; PA: P. aeruginosa.
The decrease in biofilm formation for the treated groups compared with the negative control was supported by the SEM images, which show less cell density and less compacted aggregates of cells on the surfaces (Fig. 3). The ratio of S. aureus/P. aeruginosa for the negative control for both polyvinyl chloride and silicone rubber was less than 1, suggesting these polymers were selective for P. aeruginosa (Table 3). Polyvinyl chloride and silicone rubber consist of other elements such as chloride, oxygen and silicone besides hydrocarbons . The ratio of less than 1 seen on polyvinyl chloride and silicone rubber became normalized to a ratio of more than 1 due to the presence of a betacyanin fraction that affected the cell hydrophobicity (Table 3). There was not a substantial variation in the number of bacteria attached to these surfaces. The betacyanin fraction formulation was able to reduce 1.0‒1.3 log CFU cm−2 of bacteria attached to these polymer surfaces (Table 2). When S. aureus and P. aeruginosa were co-cultured on polystyrene (Table 3), the estimated cell numbers in the biofilm for S. aureus were similar to those in its mono species biofilm (7.85 ± 0.06 log CFU cm−2). The estimated cell numbers in the biofilm for P. aeruginosa, on the other hand, were significantly lower (P < 0.05) compared with its mono species biofilm (7.63 ± 0.04 log CFU cm−2).
DISCUSSION
When combining 2.5 mg mL−1 of each betacyanin fraction from red pitahaya and red spinach, more than 50% of biofilm was inhibited for S. aureus and P. aeruginosa. Other studies used higher concentrations of ginger and garlic extracts (more than 5 mg mL−1) to inhibit 50‒80% biofilm of S. aureus clinical isolates and P. aeruginosa isolates (Kim and Park 2013; Mohsenipour and Hassanshahian 2015). Inhibition of biofilm by Amomum tsaoko extract at 4 mg mL−1 was less than 50% against S. aureus and P. aeruginosa individual biofilm (Rahman et al. 2017). Our screening result shows that a combination of 0.625 mg mL−1 of the betacyanin fraction from red pitahaya and red spinach was able to inhibit biofilm formation of both S. aureus and P. aeruginosa. The limitation of using an agent that interferes with bacterial cell viability is the development of bacterial resistance to the agent. We, therefore, focused on changing the properties of biofilm formation without affecting microbial cell viability. This formulation was chosen for the subsequent experiment to characterize its effect on dual-species biofilm on different polymer surfaces. Our previous study showed that betacyanin from red pitahaya was not cytotoxic at the concentrations of 0.39–3.13 mg mL−1 against human embryonic kidney cells (HEK-293) and human monocytes (THP-1) (Yong et al. 2018).
Staphylococcus aureus (ATCC 6538P) and P. aeruginosa (ATCC 27853) were selected to co-culture for biofilm formation because both of them are good biofilm formers and the anti-biofilm effect of the betacyanin fraction formulation against them was also prominent. However, a FIC index of 1.0 indicates that there was no interaction between the red pitahaya betacyanin fraction and red spinach betacyanin fraction. It is possible that betacyanins act differently against each bacteria due to different characteristics such as cell wall structure and mobility. It has been reported that β-type proanthocyanidin can inhibit adhesion of S. aureus (Trentin et al. 2015), whereas A-type proanthocyanidin can disrupt P. aeruginosa biofilm production by reducing their swarming motility (Ulrey et al. 2014).
When S. aureus and P. aeruginosa were co-cultured to form a biofilm, the density of the biofilm produced was more intense and difficult to control due to both competitive and mutually beneficial interactions between S. aureus and P. aeruginosa during early biofilm establishment (Alves et al. 2018). A study revealed that eDNA of S. aureus might interact with type lV pili of P. aeruginosa when forming mixed species microcolonies (Yang et al. 2011). Both S. aureus and P. aeruginosa have commensal-like interaction in cystic fibrosis infections, where enhanced growth of P. aeruginosa was observed in the presence of S. aureus (Michelsen et al. 2014). This consequently caused an increase in resistance to antibiotic treatment (Beaudoin et al. 2017).
Betacyanin fractions from red pitahaya showed biofilm-inhibiting activity on P. aeruginosa via the activation of the expression of genes for synthesis of pili and flagella of P. aeruginosa thereby reducing adherence (Whiteley et al. 2001). The reasoning for the positive interactions of betacyanins with bacteria are still not established and this requires further investigation. Increasing anti-biofilm spectrum of an agent can improve the effectiveness of therapeutic strategies. To the authors’ best knowledge, the inhibitory activity of plant extracts against dual-species biofilm of Gram-positive and Gram-negative bacteria has not been reported.
In this research, the biofilm formation of the co-cultured species is suggested to be more dependent on the water contact angle rather than surface properties. This phenomenon is not seen on individual P. aeruginosa, which indicates the presence of an interaction between S. aureus and P. aeruginosa in adhering to a surface in forming a biofilm. Pseudomonas aeruginosa was able to inhibit the biofilm formation of Staphylococcus epidermidis and S. aureus (Filkins et al. 2015; Pihl et al. 2010). This research did not show a similar trend as the presence of P. aeruginosa did not reduce the cell density of S. aureus adhered to the surfaces (Fig. 3). This can be due to strain variation. Silicone rubber, which has different roughness, porosity and surface functional groups, can result in greater P. aeruginosa adhesion to the surfaces compared with other less hydrophobic surfaces. Saphylococcus aureus has a competitive advantage over P. aeruginosa in terms of attachment to polyvinyl chloride, polyethylene and polypropylene, but S. aureus promotes increased aggregation of P. aeruginosa resulting in more attachment seen on highly hydrophobic silicone rubber surfaces. Compared with other polymers, silicone rubber has been a material of choice for making tubing, feeding tubes, catheters, drains, implants for short-term or long-term use, as well as seals and gaskets, in the field of medicine. Silicone materials have desirable properties such as great flexibility and reduced immunological reactivity, but are is not impervious to bacterial colonization (Burroughs et al. 2020). The interaction of bacteria from different species can also affect the ability of bacteria to attach to a surface compared with a single species. This is because the factors involved in cell surface hydrophobicity and adhesion are different: lipoteichoic acid for Staphylococcus and outer membrane protein for Pseudomonas (Krasowska and Sigler 2014).
The adherence step is irreversible after several hours of incubation, so the betacyanin fraction formulation might help in interfering with the interaction of bacteria with a surface. Saphylococcus aureus and P. aeruginosa can exhibit cell-to-cell communication, induce the expression of biofilm-forming factors (Pastar et al. 2013) and act synergistically within common multiple species to survive harsh environmental conditions (Stoodley et al. 2002). Betacyanin fraction formulation in this research was able to reduce ~1 log of bacteria attached to the polymer surfaces. Gibson et al. (1999) reported that effective cleaning in an industry should achieve at least 1 log order of microorganisms from surfaces. The betacyanin fraction formulation likely inhibited biofilm formation by reducing bacterial attachment as one of the mechanisms of action when tested on polymers with a range of hydrophobicity indexes. The reduction of attachment was greatest on silicone rubber and this is in agreement with the result of biofilm inhibition.
In summary, combined betacyanin fractions exhibited improved anti-biofilm activity when they were applied together to act against dual-species biofilm of S. aureus and P. aeruginosa compared with when they were applied individually. This formulation can potentially be used as an active ingredient to prevent biofilm-associated infection.
ACKNOWLEDGEMENTS
Funding was received from the School of Science of Monash University Malaysia.
Conflict of interest
None declared.