-
PDF
- Split View
-
Views
-
Cite
Cite
Lena Ciric, Michael S. M. Brouwer, Peter Mullany, Adam P. Roberts, Minocycline resistance in an oral Streptococcus infantis isolate is encoded by tet(S) on a novel small, low copy number plasmid, FEMS Microbiology Letters, Volume 353, Issue 2, April 2014, Pages 106–115, https://doi.org/10.1111/1574-6968.12410
- Share Icon Share
Abstract
We have determined the genetic basis of minocycline resistance in a strain of Streptococcus infantis isolated from a healthy human oral cavity. We demonstrate that tet(S), identical to tet(S) found on the enterococcal conjugative transposon Tn6000, is responsible for the observed resistance. The gene is located on a small, low copy number plasmid and is flanked by IS1216 elements. The tet(S) gene is capable of excising from the plasmid together with one of the IS1216 elements. The plasmid contains a putative toxin/antitoxin system related to relBE. Deletion of the toxin, relE, did not result in plasmid instability but did increase the fitness of the mutant compared to the wild-type strain.
Introduction
Antibiotic resistance is one of the biggest challenges currently facing modern health care. Excessive and inappropriate use of antibiotics has resulted in a high selective pressure for bacteria to either evolve or acquire resistance genes (Andersson, 2003).
The oral cavity of healthy humans is a reservoir for antibiotic resistance genes (Seville et al., 2009; Roberts & Mullany, 2011), and resistance to antimicrobials such as tetracyclines (Lancaster et al., 2003; Villedieu et al., 2003), macrolides (Villedieu et al., 2004; Seville et al., 2009) and amoxicillin (Ready et al., 2004) has been found.
Tetracycline resistance is particularly common in the oral cavity microbial communities (Villedieu et al., 2003; Seville et al., 2009; Ciric et al., 2012), and the genes primarily responsible are those encoding ribosomal protection proteins (RPPs), which also confer resistance to minocycline (Seville et al., 2009). Of these RPP-encoding genes, tet(M) is the most common and is usually found within conjugative transposons of the Tn916 family (Seville et al., 2009; Roberts & Mullany, 2011; Ciric et al., 2012). Another RPP gene, tet(S), which is found much less often in the human oral cavity (Seville et al., 2009), shows 79% amino acid identity with tet(M) (Charpentier et al., 1993). The tet(S) gene was originally found in Listeria monocytogenes (Charpentier et al., 1993) and was recently discovered within a conjugative transposon of the Tn916 family (designated Tn6000) from Enterococcus casseliflavus (Roberts et al., 2006; Brouwer et al., 2010). Recently, tet(S) has been reported in a variety of Enterococcus spp., often on a derivative of Tn6000 and often associated with the insertion sequence IS1216 (Novais et al., 2012).
In this work, we have determined the molecular basis of tetracycline resistance in a Streptococcus infantis isolate from the oral cavity of a healthy human. We show tet(S) is present on a small, low copy number plasmid and is capable of excising from the plasmid with one of the two IS1216 elements which flank it and likely mediate its excision.
Materials and methods
Sampling
Saliva was obtained from 10 healthy volunteers who had not received antibiotics in the previous 3 months as previously described (Ciric et al., 2011). Ethical approval for the project was obtained from the University College London (UCL) Ethics Committee (Project No. 1364/001).
Bacterial strains and plasmids
All bacterial strains and plasmids used in this study are listed in Table 1. Streptococcus infantis C.MI.8 was cultured in 5% CO2 on Columbia blood agar (Oxoid) containing 4 mg L−1 minocycline at 37 °C. Streptococcus australis FRStet12 was cultured under the same conditions without antibiotics. Escherichia coli was grown on or in LB agar or broth containing 100 mg L−1 ampicillin when required.
Bacterial strains and plasmids used in this study
Bacterial strain name | Comments | Reference or source |
Streptococcus infantis C.MI.8 | Original isolate containing pSI01. MinR | This study |
Streptococcus australis FRStet12 | Recipient strain. TetR, MinS | Seville (2007) |
Streptococcus australis FRStet12::pSI01 | Recipient strain transformed with pSI01. MinR, TetR | This study |
Streptococcus australis FRStet12::pSI01ΔrelE | Recipient strain transformed with pSI01ΔrelE. MinR, TetR | This study |
Escherichia coli Alpha-Select Bronze | Used for general cloning | Bioline |
Plasmids | ||
pGEM T-easy | Vector for TA cloning. AmpR | Promega |
pSI01 | S. infantis C.MI.8 plasmid. MinR | This work |
pSI01ΔrelE | S. infantis C.MI.8 plasmid relE knockout. MinR | This work |
Bacterial strain name | Comments | Reference or source |
Streptococcus infantis C.MI.8 | Original isolate containing pSI01. MinR | This study |
Streptococcus australis FRStet12 | Recipient strain. TetR, MinS | Seville (2007) |
Streptococcus australis FRStet12::pSI01 | Recipient strain transformed with pSI01. MinR, TetR | This study |
Streptococcus australis FRStet12::pSI01ΔrelE | Recipient strain transformed with pSI01ΔrelE. MinR, TetR | This study |
Escherichia coli Alpha-Select Bronze | Used for general cloning | Bioline |
Plasmids | ||
pGEM T-easy | Vector for TA cloning. AmpR | Promega |
pSI01 | S. infantis C.MI.8 plasmid. MinR | This work |
pSI01ΔrelE | S. infantis C.MI.8 plasmid relE knockout. MinR | This work |
Min, minocycline; Tet, tetracycline; Rif, rifampicin; Fus, fusidic acid; Strep, streptomycin; R, resistant; S, susceptible; TA, toxin–antitoxin.
Bacterial strains and plasmids used in this study
Bacterial strain name | Comments | Reference or source |
Streptococcus infantis C.MI.8 | Original isolate containing pSI01. MinR | This study |
Streptococcus australis FRStet12 | Recipient strain. TetR, MinS | Seville (2007) |
Streptococcus australis FRStet12::pSI01 | Recipient strain transformed with pSI01. MinR, TetR | This study |
Streptococcus australis FRStet12::pSI01ΔrelE | Recipient strain transformed with pSI01ΔrelE. MinR, TetR | This study |
Escherichia coli Alpha-Select Bronze | Used for general cloning | Bioline |
Plasmids | ||
pGEM T-easy | Vector for TA cloning. AmpR | Promega |
pSI01 | S. infantis C.MI.8 plasmid. MinR | This work |
pSI01ΔrelE | S. infantis C.MI.8 plasmid relE knockout. MinR | This work |
Bacterial strain name | Comments | Reference or source |
Streptococcus infantis C.MI.8 | Original isolate containing pSI01. MinR | This study |
Streptococcus australis FRStet12 | Recipient strain. TetR, MinS | Seville (2007) |
Streptococcus australis FRStet12::pSI01 | Recipient strain transformed with pSI01. MinR, TetR | This study |
Streptococcus australis FRStet12::pSI01ΔrelE | Recipient strain transformed with pSI01ΔrelE. MinR, TetR | This study |
Escherichia coli Alpha-Select Bronze | Used for general cloning | Bioline |
Plasmids | ||
pGEM T-easy | Vector for TA cloning. AmpR | Promega |
pSI01 | S. infantis C.MI.8 plasmid. MinR | This work |
pSI01ΔrelE | S. infantis C.MI.8 plasmid relE knockout. MinR | This work |
Min, minocycline; Tet, tetracycline; Rif, rifampicin; Fus, fusidic acid; Strep, streptomycin; R, resistant; S, susceptible; TA, toxin–antitoxin.
DNA extraction
Total genomic DNA was extracted using the ArchivePure DNA Yeast and Gram Positive Bacteria Kit (5Prime) according to the manufacturer's instructions. Mutanolysin at a final concentration of 15 μg mL−1 and lysozyme at a final concentration of 3 μg mL−1 were added at the cell lysis step of the protocol. DNA was stored at −20 °C. Plasmid DNA extractions were performed using the QIAprep Spin Miniprep Kit (QIAGEN) according to the manufacturer's instructions with the following modification; mutanolysin and lysozyme were added to buffer P1 at the above concentrations and the cells were incubated for 1 h at 37 °C before proceeding with the remainder of the protocol. Plasmid DNA was eluted in sterile water heated to 70 °C.
PCR and sequencing
The sequences of all primers used in the study are shown in Table 2. Standard PCR was carried out using BioMix™ Red (Bioline) and long PCR was carried out with BIO-X-ACT™ Long Mix (Bioline) according to the manufacturer's instructions. RPP PCR using degenerate primers (RPPF and RPPR; Warburton et al., 2009) was used to identify RPP genes within S. infantis C.MI.8. The S. infantis C.MI.8 isolate was identified by sequencing of the superoxide dismutase gene, sod(A), as described by Poyart et al. (1998). Single-specific primer (ssp) PCR was performed in order to ascertain the sequence of the DNA flanking the S. infantis C.MI.8 RPP gene. All DNA sequencing was carried out at the UCL Wolfson Institute DNA sequencing facility. Quantitative PCR was used to estimate the copy number of the plasmid as described previously (Chen et al., 2011). Reactions were run using the Sensimix SYBR No-ROX kit (Bioline) on the Rotor-Gene 6500 system (QIAGEN). Primers were designed to amplify regions of c. 100 bp of the pSI01 rep gene and the S. infantis C.MI.8 sod(A) gene. The copy number was calculated as the mean threshold cycle (CT) of the amplicons of the sod(A) gene (single-copy chromosomal gene) compared to the amplicon of the plasmid rep gene. The formula , ΔCT being the difference between the threshold cycle number of the reference sod(A) gene and that of rep.
Primers used in this study
Name | Sequence (5′–3′) | Gene target | References |
RPP-F | CCIGGVCAYATGGAYTTYHTDGC | RPP genes | Warburton et al. (2009) |
RPP-R | CKRAARTCIGMIGGIGTRCTIACHGG | RPP genes | Warburton et al. (2009) |
d1 | CCITAYICITAYGAYGCIYTIGARCC | sod(A) | Poyart et al. (1998) |
d2 | ARRTARTAIGCRTGYTCCCAIACRTC | sod(A) | Poyart et al. (1998) |
Single-specific primer PCR and sequencing primers | |||
tet(S)-IS-5R-1 | CGTGAGTGCTCCAATCAGCTGG | This study | |
tet(S)-IS-5R-2 | GGATTGTAGTAGTCCGGCACG | This study | |
tet(S)-IS-3R-1 | GCCCAGGTGCGCCTTGTACG | This study | |
tet(S)-IS-3R-2 | AGCTACTCGTTGTGATAACC | This study | |
tet(S)-1F | GGAACGCTACATTTGCGAGACTC | This study | |
tet(S)-2F | GTCTGCTTTCCAACCACGTCGTCC | This study | |
tet(S)-3F | CCCTTACGGTATCTATGATTTAA | This study | |
tet(S)-IS-1F | GGTCGTTATACACGGGGCAT | This study | |
tet(S)-IS-2F | CCAAGCTTCTATTGTGAAGGC | This study | |
tet(S)-IS-3F | GAACACAAGTTTCCAAGGGC | This study | |
tet(S)-3R | AGGGCACAGTATCCACCTAGT | This study | |
tet(S)-1R | CGAGTTTGTGACTGTACTCCATC | This study | |
tet(S)-2R | CCTGCATCAACATGTGCTAAGATACCG | This study | |
tet(S)-IS-1R | CAGGTCAATAGGACAACTGTCC | This study | |
tet(S)-IS-2R | TTACGCACTGCCTCACCCACG | This study | |
tet(S)-IS-3R | GGGTTTTGCATCAACATATTTAG | This study | |
tet(S)-IS-4R | GAACGAGCGAGACGTAACCG | This study | |
tet(S)-IS-5R | GGGATAAAGACACTTCTGACC | This study | |
tet(S)-IS-6R | ACTTCTCTTACCAATAGCAATGAC | This study | |
pTETS-1 | CACCAGTATGTATTAAACCATAG | This study | |
PCR1-1F | GATACCTGAAGCACAAGATG | This study | |
PCR1-2F | GAGACGAGCGTTCTGACGC | This study | |
pTETS-2 | CAATGATCTGACGACCAATTTCG | This study | |
PCR2-1F | GGAAGTGTAGATAGCGGTAC | This study | |
pTETS-3 | GATAAGGCAGAGCCTGGTGAG | This study | |
pTETS-4 | GTAGAAGGGGATCACTATCGG | This study | |
pTETS-5 | TTCCCTTACCGAGTCAGCTTG | This study | |
pTETS-6 | GATGCTGAGATAGTCATTCTG | This study | |
IS1216 element excision | |||
tet(S)IS-1 | GCGCAGCTGAAATTATCAGC | This study | |
tet(S)IS-2 | TCAGGTCAATAGGACAACTG | This study | |
tet(S)IS-3 | GATGAAGTACAGAGTTACTCG | This study | |
tet(S)IS-4 | AAGCCTCACTTTCAACACG | This study | |
Probe amplification for Southern blot | |||
ISF | GATAGCAGGAGGAATGACGATG | Ciric et al. (2011) | |
ISR | CCCTTACGGCATCTATGA | Ciric et al. (2011) | |
PCR2-1F | As above | ||
pTETS-4 | As above | ||
qPCR primers for copy number | |||
rep-F | CTGGATCATACGTGCCAACGAC | This study | |
rep-R | CGATAGGGCGGTCATCACTCCG | This study | |
C. MI.8 sodA-F | GCTTGCTGATGTAGAATCTATCC | This study | |
C. MI.8 sodA-R | GTTGAAGTCACTTCAAGCTTACC | This study | |
relE-knockout primers | |||
relE check F | GATCAGAATGACTATCTCAGCATCA | This study | |
relE check R | GAGCGTTCAAATCAGCGTTA | This study | |
mut relE F | AACTGGCTATTGCTTGTTTCAATTATGT | This study | |
mut relE R | AACTCAATCCCAACCATCCTCTACATTT | This study |
Name | Sequence (5′–3′) | Gene target | References |
RPP-F | CCIGGVCAYATGGAYTTYHTDGC | RPP genes | Warburton et al. (2009) |
RPP-R | CKRAARTCIGMIGGIGTRCTIACHGG | RPP genes | Warburton et al. (2009) |
d1 | CCITAYICITAYGAYGCIYTIGARCC | sod(A) | Poyart et al. (1998) |
d2 | ARRTARTAIGCRTGYTCCCAIACRTC | sod(A) | Poyart et al. (1998) |
Single-specific primer PCR and sequencing primers | |||
tet(S)-IS-5R-1 | CGTGAGTGCTCCAATCAGCTGG | This study | |
tet(S)-IS-5R-2 | GGATTGTAGTAGTCCGGCACG | This study | |
tet(S)-IS-3R-1 | GCCCAGGTGCGCCTTGTACG | This study | |
tet(S)-IS-3R-2 | AGCTACTCGTTGTGATAACC | This study | |
tet(S)-1F | GGAACGCTACATTTGCGAGACTC | This study | |
tet(S)-2F | GTCTGCTTTCCAACCACGTCGTCC | This study | |
tet(S)-3F | CCCTTACGGTATCTATGATTTAA | This study | |
tet(S)-IS-1F | GGTCGTTATACACGGGGCAT | This study | |
tet(S)-IS-2F | CCAAGCTTCTATTGTGAAGGC | This study | |
tet(S)-IS-3F | GAACACAAGTTTCCAAGGGC | This study | |
tet(S)-3R | AGGGCACAGTATCCACCTAGT | This study | |
tet(S)-1R | CGAGTTTGTGACTGTACTCCATC | This study | |
tet(S)-2R | CCTGCATCAACATGTGCTAAGATACCG | This study | |
tet(S)-IS-1R | CAGGTCAATAGGACAACTGTCC | This study | |
tet(S)-IS-2R | TTACGCACTGCCTCACCCACG | This study | |
tet(S)-IS-3R | GGGTTTTGCATCAACATATTTAG | This study | |
tet(S)-IS-4R | GAACGAGCGAGACGTAACCG | This study | |
tet(S)-IS-5R | GGGATAAAGACACTTCTGACC | This study | |
tet(S)-IS-6R | ACTTCTCTTACCAATAGCAATGAC | This study | |
pTETS-1 | CACCAGTATGTATTAAACCATAG | This study | |
PCR1-1F | GATACCTGAAGCACAAGATG | This study | |
PCR1-2F | GAGACGAGCGTTCTGACGC | This study | |
pTETS-2 | CAATGATCTGACGACCAATTTCG | This study | |
PCR2-1F | GGAAGTGTAGATAGCGGTAC | This study | |
pTETS-3 | GATAAGGCAGAGCCTGGTGAG | This study | |
pTETS-4 | GTAGAAGGGGATCACTATCGG | This study | |
pTETS-5 | TTCCCTTACCGAGTCAGCTTG | This study | |
pTETS-6 | GATGCTGAGATAGTCATTCTG | This study | |
IS1216 element excision | |||
tet(S)IS-1 | GCGCAGCTGAAATTATCAGC | This study | |
tet(S)IS-2 | TCAGGTCAATAGGACAACTG | This study | |
tet(S)IS-3 | GATGAAGTACAGAGTTACTCG | This study | |
tet(S)IS-4 | AAGCCTCACTTTCAACACG | This study | |
Probe amplification for Southern blot | |||
ISF | GATAGCAGGAGGAATGACGATG | Ciric et al. (2011) | |
ISR | CCCTTACGGCATCTATGA | Ciric et al. (2011) | |
PCR2-1F | As above | ||
pTETS-4 | As above | ||
qPCR primers for copy number | |||
rep-F | CTGGATCATACGTGCCAACGAC | This study | |
rep-R | CGATAGGGCGGTCATCACTCCG | This study | |
C. MI.8 sodA-F | GCTTGCTGATGTAGAATCTATCC | This study | |
C. MI.8 sodA-R | GTTGAAGTCACTTCAAGCTTACC | This study | |
relE-knockout primers | |||
relE check F | GATCAGAATGACTATCTCAGCATCA | This study | |
relE check R | GAGCGTTCAAATCAGCGTTA | This study | |
mut relE F | AACTGGCTATTGCTTGTTTCAATTATGT | This study | |
mut relE R | AACTCAATCCCAACCATCCTCTACATTT | This study |
RPP, ribosomal protection protein.
Primers used in this study
Name | Sequence (5′–3′) | Gene target | References |
RPP-F | CCIGGVCAYATGGAYTTYHTDGC | RPP genes | Warburton et al. (2009) |
RPP-R | CKRAARTCIGMIGGIGTRCTIACHGG | RPP genes | Warburton et al. (2009) |
d1 | CCITAYICITAYGAYGCIYTIGARCC | sod(A) | Poyart et al. (1998) |
d2 | ARRTARTAIGCRTGYTCCCAIACRTC | sod(A) | Poyart et al. (1998) |
Single-specific primer PCR and sequencing primers | |||
tet(S)-IS-5R-1 | CGTGAGTGCTCCAATCAGCTGG | This study | |
tet(S)-IS-5R-2 | GGATTGTAGTAGTCCGGCACG | This study | |
tet(S)-IS-3R-1 | GCCCAGGTGCGCCTTGTACG | This study | |
tet(S)-IS-3R-2 | AGCTACTCGTTGTGATAACC | This study | |
tet(S)-1F | GGAACGCTACATTTGCGAGACTC | This study | |
tet(S)-2F | GTCTGCTTTCCAACCACGTCGTCC | This study | |
tet(S)-3F | CCCTTACGGTATCTATGATTTAA | This study | |
tet(S)-IS-1F | GGTCGTTATACACGGGGCAT | This study | |
tet(S)-IS-2F | CCAAGCTTCTATTGTGAAGGC | This study | |
tet(S)-IS-3F | GAACACAAGTTTCCAAGGGC | This study | |
tet(S)-3R | AGGGCACAGTATCCACCTAGT | This study | |
tet(S)-1R | CGAGTTTGTGACTGTACTCCATC | This study | |
tet(S)-2R | CCTGCATCAACATGTGCTAAGATACCG | This study | |
tet(S)-IS-1R | CAGGTCAATAGGACAACTGTCC | This study | |
tet(S)-IS-2R | TTACGCACTGCCTCACCCACG | This study | |
tet(S)-IS-3R | GGGTTTTGCATCAACATATTTAG | This study | |
tet(S)-IS-4R | GAACGAGCGAGACGTAACCG | This study | |
tet(S)-IS-5R | GGGATAAAGACACTTCTGACC | This study | |
tet(S)-IS-6R | ACTTCTCTTACCAATAGCAATGAC | This study | |
pTETS-1 | CACCAGTATGTATTAAACCATAG | This study | |
PCR1-1F | GATACCTGAAGCACAAGATG | This study | |
PCR1-2F | GAGACGAGCGTTCTGACGC | This study | |
pTETS-2 | CAATGATCTGACGACCAATTTCG | This study | |
PCR2-1F | GGAAGTGTAGATAGCGGTAC | This study | |
pTETS-3 | GATAAGGCAGAGCCTGGTGAG | This study | |
pTETS-4 | GTAGAAGGGGATCACTATCGG | This study | |
pTETS-5 | TTCCCTTACCGAGTCAGCTTG | This study | |
pTETS-6 | GATGCTGAGATAGTCATTCTG | This study | |
IS1216 element excision | |||
tet(S)IS-1 | GCGCAGCTGAAATTATCAGC | This study | |
tet(S)IS-2 | TCAGGTCAATAGGACAACTG | This study | |
tet(S)IS-3 | GATGAAGTACAGAGTTACTCG | This study | |
tet(S)IS-4 | AAGCCTCACTTTCAACACG | This study | |
Probe amplification for Southern blot | |||
ISF | GATAGCAGGAGGAATGACGATG | Ciric et al. (2011) | |
ISR | CCCTTACGGCATCTATGA | Ciric et al. (2011) | |
PCR2-1F | As above | ||
pTETS-4 | As above | ||
qPCR primers for copy number | |||
rep-F | CTGGATCATACGTGCCAACGAC | This study | |
rep-R | CGATAGGGCGGTCATCACTCCG | This study | |
C. MI.8 sodA-F | GCTTGCTGATGTAGAATCTATCC | This study | |
C. MI.8 sodA-R | GTTGAAGTCACTTCAAGCTTACC | This study | |
relE-knockout primers | |||
relE check F | GATCAGAATGACTATCTCAGCATCA | This study | |
relE check R | GAGCGTTCAAATCAGCGTTA | This study | |
mut relE F | AACTGGCTATTGCTTGTTTCAATTATGT | This study | |
mut relE R | AACTCAATCCCAACCATCCTCTACATTT | This study |
Name | Sequence (5′–3′) | Gene target | References |
RPP-F | CCIGGVCAYATGGAYTTYHTDGC | RPP genes | Warburton et al. (2009) |
RPP-R | CKRAARTCIGMIGGIGTRCTIACHGG | RPP genes | Warburton et al. (2009) |
d1 | CCITAYICITAYGAYGCIYTIGARCC | sod(A) | Poyart et al. (1998) |
d2 | ARRTARTAIGCRTGYTCCCAIACRTC | sod(A) | Poyart et al. (1998) |
Single-specific primer PCR and sequencing primers | |||
tet(S)-IS-5R-1 | CGTGAGTGCTCCAATCAGCTGG | This study | |
tet(S)-IS-5R-2 | GGATTGTAGTAGTCCGGCACG | This study | |
tet(S)-IS-3R-1 | GCCCAGGTGCGCCTTGTACG | This study | |
tet(S)-IS-3R-2 | AGCTACTCGTTGTGATAACC | This study | |
tet(S)-1F | GGAACGCTACATTTGCGAGACTC | This study | |
tet(S)-2F | GTCTGCTTTCCAACCACGTCGTCC | This study | |
tet(S)-3F | CCCTTACGGTATCTATGATTTAA | This study | |
tet(S)-IS-1F | GGTCGTTATACACGGGGCAT | This study | |
tet(S)-IS-2F | CCAAGCTTCTATTGTGAAGGC | This study | |
tet(S)-IS-3F | GAACACAAGTTTCCAAGGGC | This study | |
tet(S)-3R | AGGGCACAGTATCCACCTAGT | This study | |
tet(S)-1R | CGAGTTTGTGACTGTACTCCATC | This study | |
tet(S)-2R | CCTGCATCAACATGTGCTAAGATACCG | This study | |
tet(S)-IS-1R | CAGGTCAATAGGACAACTGTCC | This study | |
tet(S)-IS-2R | TTACGCACTGCCTCACCCACG | This study | |
tet(S)-IS-3R | GGGTTTTGCATCAACATATTTAG | This study | |
tet(S)-IS-4R | GAACGAGCGAGACGTAACCG | This study | |
tet(S)-IS-5R | GGGATAAAGACACTTCTGACC | This study | |
tet(S)-IS-6R | ACTTCTCTTACCAATAGCAATGAC | This study | |
pTETS-1 | CACCAGTATGTATTAAACCATAG | This study | |
PCR1-1F | GATACCTGAAGCACAAGATG | This study | |
PCR1-2F | GAGACGAGCGTTCTGACGC | This study | |
pTETS-2 | CAATGATCTGACGACCAATTTCG | This study | |
PCR2-1F | GGAAGTGTAGATAGCGGTAC | This study | |
pTETS-3 | GATAAGGCAGAGCCTGGTGAG | This study | |
pTETS-4 | GTAGAAGGGGATCACTATCGG | This study | |
pTETS-5 | TTCCCTTACCGAGTCAGCTTG | This study | |
pTETS-6 | GATGCTGAGATAGTCATTCTG | This study | |
IS1216 element excision | |||
tet(S)IS-1 | GCGCAGCTGAAATTATCAGC | This study | |
tet(S)IS-2 | TCAGGTCAATAGGACAACTG | This study | |
tet(S)IS-3 | GATGAAGTACAGAGTTACTCG | This study | |
tet(S)IS-4 | AAGCCTCACTTTCAACACG | This study | |
Probe amplification for Southern blot | |||
ISF | GATAGCAGGAGGAATGACGATG | Ciric et al. (2011) | |
ISR | CCCTTACGGCATCTATGA | Ciric et al. (2011) | |
PCR2-1F | As above | ||
pTETS-4 | As above | ||
qPCR primers for copy number | |||
rep-F | CTGGATCATACGTGCCAACGAC | This study | |
rep-R | CGATAGGGCGGTCATCACTCCG | This study | |
C. MI.8 sodA-F | GCTTGCTGATGTAGAATCTATCC | This study | |
C. MI.8 sodA-R | GTTGAAGTCACTTCAAGCTTACC | This study | |
relE-knockout primers | |||
relE check F | GATCAGAATGACTATCTCAGCATCA | This study | |
relE check R | GAGCGTTCAAATCAGCGTTA | This study | |
mut relE F | AACTGGCTATTGCTTGTTTCAATTATGT | This study | |
mut relE R | AACTCAATCCCAACCATCCTCTACATTT | This study |
RPP, ribosomal protection protein.
Southern blot hybridisation
Southern blot hybridisation was performed on HindIII-digested genomic DNA of S. infantis C.MI.8, S. australis FRStet12 and S. australis FRStet12::pSI01 using a probe specific to the tet(S) gene (JX275965, 7148–8174 bp; primers PCR2-1F and pTETS-4 in Table 2) and the IS1216 sequence (JX275965, 5719–6437 and 9535–10 253 bp; primers ISF and ISR in Table 2) from pSI01. Probes were labelled and the blots detected with the DIG-High Prime DNA Labelling and Detection Kit (Roche, UK).
Transformation studies
Streptococcus infantis C.MI.8 pSI01 plasmid DNA (20 ng) was added to minocycline-susceptible S. australis FRStet12 (Table 1) while competent during the exponential phase of growth (Warburton et al., 2013). Transformants were selected on media containing 4 mg L−1 minocycline.
Minimal inhibitory concentrations
Minimum inhibitory concentrations (MICs) were determined by agar dilution according to the British Society for Antimicrobial Chemotherapy guidelines (http://www.bsac.org.uk/) using minocycline. The concentrations tested ranged from 0.125 to 64 mg L−1. These were carried out using S. infantis C.MI.8, S. australis FRStet12 (used as a control strain as the MIC to minocycline had been previously determined; Ciric et al., 2011) and S. australis FRStet12::pSI01. Iso-sensitest agar containing 5% blood was used and the plates were incubated at 37 °C for 48 h.
Plasmid stability and mutation of relBE
To assess the contribution of the putative RelBE toxin–antitoxin (TA) system to the stability of pSI01, a deletion was made in the relE toxin component using the Phusion Site-Directed Mutagenesis Kit (Thermo Scientific). The mutant plasmid pSI01∆relE was transformed into S. australis FRStet12. PCR across the deletion was performed (primer pair: relEcheck F + R), and the products of appropriate length were sequenced to confirm the deletion of relE.
To assess the stability of pSI01, S. infantis C.MI.8, S. australis FRStet12::pSI01 and FRStet12::pSI01∆relE were cultured without selection for minocycline resistance in BHI broth for 24 h. Dilutions of the cultures were spread onto BHI and 5% defibrinated horse blood without minocycline. After 24 h, 100 single colonies were picked from nonselective plates onto plates containing minocycline (4 mg L−1). The minocycline plates were cultured for 48 h, and the proportion of resistant colonies was determined. Colony PCR was performed to establish whether both tet(S) and the plasmid backbone were present.
In subsequent experiments, FRStet12::pSI01 and FRStet12::pSI01∆relE were serially cultured in antibiotic-free BHI broth for 5 and 10 days. A 1 : 10 dilution of the culture was made with fresh broth every 24 h. Stability of the plasmids in these experiments was determined as above.
Competitive fitness experiments
Overnight cultures of S. australis FRStet12::pSI01 and FRStet12::pSI01∆relE were diluted to OD600 of 0.1, mixed and grown in competition for 5 h. Dilutions of the cultures were then spread onto BHI and 5% defibrinated horse blood without minocycline, and after 24 h, 100 colonies were picked from nonselective plates onto plates containing minocycline (4 mg L−1). After 24 h, the ratio of wild-type and RelE mutant colonies was determined by PCR across the relE region of the plasmid. The size difference in the PCR product identified whether either the wild-type or mutant relE containing plasmid was present.
Results
Isolation and genotypic characterisation of pSI01
A minocycline-resistant (MIC ≥ 32 mg L−1) Streptococcus strain designated C.MI.8 was isolated from pooled saliva collected from healthy volunteers. The strain was identified as S. infantis by the sequence of the superoxide dismutase gene, sod(A) (ID 98.39%, JN181310).
To determine whether an RPP gene was responsible for minocycline resistance, PCR using degenerate primers designed to detect RPP genes was performed. The resulting amplicon was sequenced and shown to be identical to tet(S) from the conjugative transposon Tn6000 (FN555436; Roberts et al., 2006; Brouwer et al., 2010).
The DNA sequence flanking tet(S) was determined and this demonstrated that the gene was contained on a plasmid which we designated pSI01 (Fig. 1 and Table 3. Accession number JX275965). The plasmid had an overall GC content of 34.73%, which was consistent throughout the ORFs; it possesses a predicted TA addiction system with homology to the relBE module found in the Streptococcus parasanguinis plasmid pFW213 (EU685104, 99.27%). The antitoxin relB differs from that of pFW213 by two SNPs and the toxin relE by one SNP. The SNPs result in one amino acid sequence change in RelB (S52F) and no amino acid changes in RelE. Orf3 is predicted to encode a hypothetical protein that has 91.41% amino acid similarity to a hypothetical protein found in S. infantis ATCC 700779 (ZP_08062518). rep encodes a predicted replication initiation protein belonging to the Rep3 superfamily, which is related (89.04% aa sequence identity) to one found in S. parasanguinis ATCC 903 (ZP_08064587) and to (68% aa sequence identity) a proven Rep of Streptococcus bovis plasmid pSB01 (BAA35196; Nakamura et al., 2001). Orf5 encodes another hypothetical protein with identity (48.28% aa sequence) to a hypothetical protein found in Streptococcus suis R61 (EHC01600). The plasmid has two IS1216 sequences that flank the tet(S) region. The two insertion sequences are not identical (ID 99.63%), differing by three SNPs. The closest homologue of the IS1216 element which follows orf5 is found in Enterococcus faecalis strain EF-01 plasmid pEF-01 (ID 99.42%, CP002208). The IS1216 element closest to orf10 is highly similar to one found in the Lactococcus lactis subsp. lactis bv. diacetylactis plasmid pVF22 (ID 99.71%, JN17912). The region encoding tet(S) flanked by the two IS1216 elements is almost identical to the E. casseliflavus conjugative transposon Tn6000 (ID 99.93%, FN555436). This region includes the tet(S) gene and a Tn916-like orf6. Finally, orf10 is predicted to encode a hypothetical protein similar to one found in Streptococcus ratti FA-1 (aa ID 77.56%, ZP_10793128).
Details of predicted ORFs on pSI01
Coding region | Position on pSI01 (bp) | Size (bp) | Strand | Closest homologue (BlastN) | Closest homologue (BlastX) | Percentage identity and coverage (%) | Accession numbers |
relB | 432–680 | 249 | + | RelB – addiction module antitoxin | – | 98.80, 100 | EU685104 |
relE | 671–976 | 306 | + | RelE – addiction module toxin | – | 99.67, 100 | EU685104 |
orf3 | 1155–1550 | 396 | − | – | Hypothetical protein | 91.41, 96 | ZP_08062518 |
rep | 1914–2795 | 882 | − | – | Replication initiation protein – Rep3 superfamily | 89.04, 98 | ZP_08064587 |
orf5 | 3501–4433 | 933 | − | – | Hypothetical protein | 48.28, 92 | EHC01600 |
IS1216 tnp | 5738–6424 | 687 | − | IS1216 transposase | – | 99.42, 100 | CP002208 |
tet(S) | 7034–8974 | 1941 | + | Tet(S) – tetracycline resistance protein | – | 100, 100 | FN555436 |
orf6 | 9098–9229 | 132 | + | Tn916-like Orf6 – conjugative transposon protein | – | 100, 100 | FN555436 |
IS1216 tnp | 9554–10 240 | 687 | − | IS1216 transposase | – | 99.71, 100 | JN172912 |
orf10 | 10 410–11 027 | 618 | − | – | Hypothetical protein | 77.56, 99 | ZP_10793128 |
Coding region | Position on pSI01 (bp) | Size (bp) | Strand | Closest homologue (BlastN) | Closest homologue (BlastX) | Percentage identity and coverage (%) | Accession numbers |
relB | 432–680 | 249 | + | RelB – addiction module antitoxin | – | 98.80, 100 | EU685104 |
relE | 671–976 | 306 | + | RelE – addiction module toxin | – | 99.67, 100 | EU685104 |
orf3 | 1155–1550 | 396 | − | – | Hypothetical protein | 91.41, 96 | ZP_08062518 |
rep | 1914–2795 | 882 | − | – | Replication initiation protein – Rep3 superfamily | 89.04, 98 | ZP_08064587 |
orf5 | 3501–4433 | 933 | − | – | Hypothetical protein | 48.28, 92 | EHC01600 |
IS1216 tnp | 5738–6424 | 687 | − | IS1216 transposase | – | 99.42, 100 | CP002208 |
tet(S) | 7034–8974 | 1941 | + | Tet(S) – tetracycline resistance protein | – | 100, 100 | FN555436 |
orf6 | 9098–9229 | 132 | + | Tn916-like Orf6 – conjugative transposon protein | – | 100, 100 | FN555436 |
IS1216 tnp | 9554–10 240 | 687 | − | IS1216 transposase | – | 99.71, 100 | JN172912 |
orf10 | 10 410–11 027 | 618 | − | – | Hypothetical protein | 77.56, 99 | ZP_10793128 |
Details of predicted ORFs on pSI01
Coding region | Position on pSI01 (bp) | Size (bp) | Strand | Closest homologue (BlastN) | Closest homologue (BlastX) | Percentage identity and coverage (%) | Accession numbers |
relB | 432–680 | 249 | + | RelB – addiction module antitoxin | – | 98.80, 100 | EU685104 |
relE | 671–976 | 306 | + | RelE – addiction module toxin | – | 99.67, 100 | EU685104 |
orf3 | 1155–1550 | 396 | − | – | Hypothetical protein | 91.41, 96 | ZP_08062518 |
rep | 1914–2795 | 882 | − | – | Replication initiation protein – Rep3 superfamily | 89.04, 98 | ZP_08064587 |
orf5 | 3501–4433 | 933 | − | – | Hypothetical protein | 48.28, 92 | EHC01600 |
IS1216 tnp | 5738–6424 | 687 | − | IS1216 transposase | – | 99.42, 100 | CP002208 |
tet(S) | 7034–8974 | 1941 | + | Tet(S) – tetracycline resistance protein | – | 100, 100 | FN555436 |
orf6 | 9098–9229 | 132 | + | Tn916-like Orf6 – conjugative transposon protein | – | 100, 100 | FN555436 |
IS1216 tnp | 9554–10 240 | 687 | − | IS1216 transposase | – | 99.71, 100 | JN172912 |
orf10 | 10 410–11 027 | 618 | − | – | Hypothetical protein | 77.56, 99 | ZP_10793128 |
Coding region | Position on pSI01 (bp) | Size (bp) | Strand | Closest homologue (BlastN) | Closest homologue (BlastX) | Percentage identity and coverage (%) | Accession numbers |
relB | 432–680 | 249 | + | RelB – addiction module antitoxin | – | 98.80, 100 | EU685104 |
relE | 671–976 | 306 | + | RelE – addiction module toxin | – | 99.67, 100 | EU685104 |
orf3 | 1155–1550 | 396 | − | – | Hypothetical protein | 91.41, 96 | ZP_08062518 |
rep | 1914–2795 | 882 | − | – | Replication initiation protein – Rep3 superfamily | 89.04, 98 | ZP_08064587 |
orf5 | 3501–4433 | 933 | − | – | Hypothetical protein | 48.28, 92 | EHC01600 |
IS1216 tnp | 5738–6424 | 687 | − | IS1216 transposase | – | 99.42, 100 | CP002208 |
tet(S) | 7034–8974 | 1941 | + | Tet(S) – tetracycline resistance protein | – | 100, 100 | FN555436 |
orf6 | 9098–9229 | 132 | + | Tn916-like Orf6 – conjugative transposon protein | – | 100, 100 | FN555436 |
IS1216 tnp | 9554–10 240 | 687 | − | IS1216 transposase | – | 99.71, 100 | JN172912 |
orf10 | 10 410–11 027 | 618 | − | – | Hypothetical protein | 77.56, 99 | ZP_10793128 |
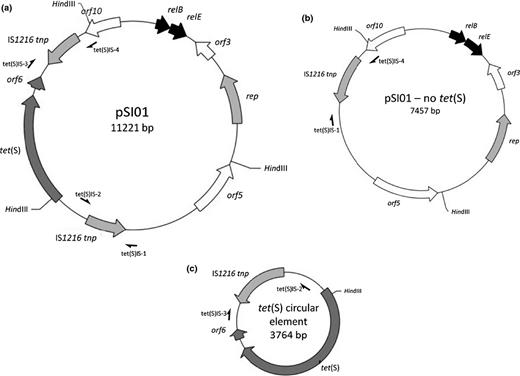
(a) Schematic of pSI01. (b, c) The recombinant forms of the plasmid detected by PCR.
qPCR was performed in order to estimate the copy number of pSI01 in S. infantis C.MI.8. As the efficiency of both plasmid and chromosomal assays was near identical (rep: M = −3.434, R2 = 0.999; sod(A): M = −3.401, R2 = 0.997), the copy number was estimated at two to three copies per cell using the method (Pfaffl, 2001).
As there are two near-identical IS1216 elements flanking tet(S), we hypothesised that this could lead to genetic instability. In order to test this, PCRs were carried out to determine whether any genetic rearrangement occurred (see Fig. 1b and c for position of primers and Table 2 for primer sequences). Four PCRs were performed using the following primer combinations: tet(S)IS-1 and tet(S)IS2; tet(S)IS-3 and tet(S)IS-4; tet(S)IS-1 and tet(S)IS-4; and tet(S)IS-2 and tet(S)IS-3. The first two assays yielded the expected products which included the IS1216 elements and the flanking regions. However, the PCR which was expected to amplify the entire region including the two IS1216 elements and the tet(S) region (using primers tet(S)IS-1 and tet(S)IS-4) resulted in an amplicon of c. 1 kb. Subsequent sequencing showed that tet(S), orf6 and one IS1216 element had excised, leaving the IS1216 element closest to orf10 within the replicon (see Fig. 1b). Furthermore, the assay designed to amplify a circular molecule using the primers tet(S)IS-2 and tet(S)IS-3 also resulted in an amplicon of c. 1 kb. Sequencing of the amplicon showed that the IS1216 element closest to orf5 had excised from pSI01 along with the tet(S) region (see Fig. 1c).
Southern blot hybridisation was performed using S. infantis C.MI.8 genomic DNA digested with HindIII. Both the tet(S) and IS1216 tnp genes were used as probes to determine whether copies of either of the genes were found anywhere other than pSI01. One copy of the tet(S) gene was found on a 3.4-kb fragment predicted by in silico digestion of pSI01 (Fig. 2a). Similarly, two copies of the IS1216 tnp gene were found on two fragments 3.7 and 3.4 kb in size, as predicted by in silico digestion (Fig. 2b). Therefore, the recombinant forms of the plasmid that were detected by PCR (shown in Fig. 1b and c) were not detectable in the genomic DNA extracted from the original S. infantis C.MI.8 isolate, suggesting that these molecules are rare.
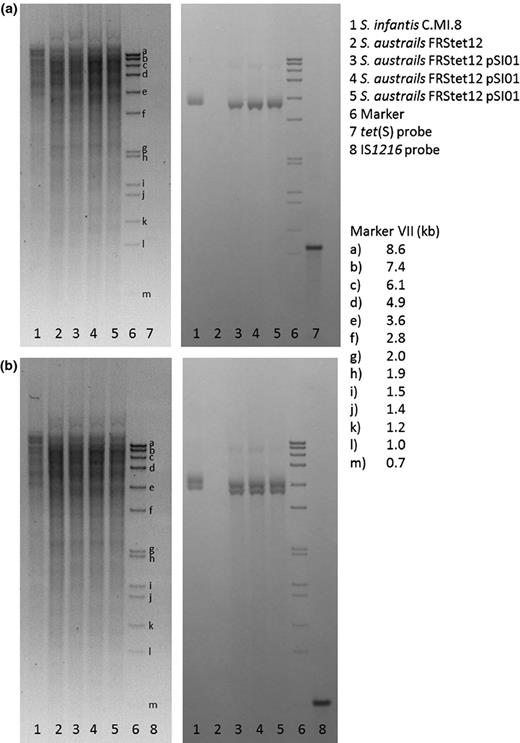
Southern blot analysis of the genomic DNA of Streptococcus infantis and Streptococcus australis transformants. We did not detect the recombinant forms shown in Fig. 1b and c in the genomic DNA of the original S. infantis isolate and the S. australis transformants. Hybridisation with (a) the tet(S) gene-derived probe and (b) the IS1216-derived probe.
pSI01 can transfer by transformation
pSI01 was transferred by transformation using plasmid DNA and the minocycline-susceptible recipient S. australis FRStet12. The transformation rate was c. 8.9 × 10−5 transformants per microgram of plasmid DNA. Four minocycline-resistant transformants were picked and screened for the presence of pSI01 by PCR amplification of tet(S). The sod(A) sequences of the four transformants were found to be identical to that of the recipient and different from the donor.
Streptococcus infantis C.MI.8 had an MIC of 32 mg L−1. The transfer of pSI01 into S. australis FRStet12 increased its minocycline MIC from 0.25 to 32 mg L−1, a 128-fold increase.
Southern blot hybridisation of S. australis FRStet12::pSI01 genomic DNA digested with HindIII using both the tet(S) and IS1216 tnp genes as probes showed one 3.4-kb fragment hybridising to tet(S) probe and two hybridising fragments of 3.7 and 3.4 kb to IS1216 tnp probe.
pSI01 stability is not affected by relE deletion but the mutant has a growth advantage
The stability of pSI01 was tested in the original host S. infantis C.MI.8. After 24-h growth in antibiotic-free medium, all 108 colonies tested were resistant to minocycline, showing that the plasmid was 100% stable after 24-h growth (with a detection limit of 0.93%).
Using PCR-based mutagenesis, we constructed pSI01∆relE (which lacks most of the sequence of the toxin component relE; bases 683–1029 of pSI01) to study the effect of the toxin on pSI01 stability. pSI01∆relE was transformed into S. australis FRStet12 and PCR across the joint of the deleted relE and subsequent sequencing of the product confirmed the deletion.
The stability of pSI01 and pSI01∆relE in S. australis FRStet12 was tested. After 24-h growth in antibiotic-free medium, cells were plated out onto antibiotic-free agar plates. The following day, 100 colonies were subcultured onto agar containing minocycline. 100% of the colonies containing pSI01 grew up on minocycline plates compared to 99% of colonies containing pSI01∆relE (average of four experiments, detection limit is 1%). The experiment was repeated with cells that were grown in antibiotic-free medium for 5 and 10 days. Again, 100 colonies were subcultured from antibiotic-free agar plates onto agar containing minocycline. When the cells were grown in antibiotic-free medium for 5 days, 100% of the colonies, containing either the wild-type or mutant plasmid, grew on minocycline (experiment performed once, detection limit 1%). When the cells were grown in antibiotic-free medium for 10 days, an average of 98% of colonies grew on minocycline regardless of which plasmid was present (average of three experiments, detection limit 1%).
To test whether all colonies that grew on minocycline plates actually contained the plasmid, PCR was conducted using primers to amplify relE or the point where relE was deleted, respectively, for pSI01 and pSI01∆relE (primer pair relE check F + R) and to amplify tet(S) (RPP-F + R). Both for the cells containing pSI01 and pSI01∆relE, the PCR for tet(S) was positive in all colonies. The PCR for relE in the cells containing pSI01 was positive in all colonies; however, the PCR across the joint where relE was deleted in pSI01∆relE was negative in c. 1% of the colonies that contained pSI01∆relE.
To test whether all colonies that grew on minocycline plates actually contained the plasmid, PCR was conducted using primers to amplify part of the plasmid backbone (primer pair relE check F + R). A total of 500 colonies were tested containing either the wild-type or mutant plasmid. These colonies were acquired from the 24-h, 5-day and 10-day experiments in antibiotic-free medium. Three colonies that were minocycline resistant and were derived from cells containing the mutant plasmid were negative for the PCR of the plasmid backbone. PCR for tet(S) (primers RPP-F + R) was positive on each of these, confirming that minocycline resistance did not occur through spontaneous mutation.
As no clear difference in stability of pSI01 was found, a competition assay was set up using FRStet12 pSI01 and FRStet12 pSI01∆relE. Overnight cultures of both strains were mixed and the mixed culture was incubated for 5 h after which the cells were spread onto antibiotic-free plates. One hundred colonies were subcultured onto plates containing minocycline and colony PCR was performed for relE in pSI01 or the junction from where relE was deleted in the recombinant plasmid (primer pair relEcheck F + R). The size of the PCR products, c. 0.9 kb for pSI01∆relE and 1.2 kb for pSI01, was used to determine which of the plasmids was present, see Table 4. On average, 80% of the colonies contained pSI01∆relE and 20% contained pSI01, based on three independent experiments.
Results of competition assays between pSI01 and pSI01∆relE in Streptococcus australis FRStet12. PCR was performed on 100 minocycline-resistant colonies in three independent experiments
Experiment 1 | Experiment 2 | Experiment 3 | Average (%) | |
pSI01 | 21 | 19 | 20 | 20 |
pSI01∆relE | 79 | 81 | 80 | 80 |
Experiment 1 | Experiment 2 | Experiment 3 | Average (%) | |
pSI01 | 21 | 19 | 20 | 20 |
pSI01∆relE | 79 | 81 | 80 | 80 |
Results of competition assays between pSI01 and pSI01∆relE in Streptococcus australis FRStet12. PCR was performed on 100 minocycline-resistant colonies in three independent experiments
Experiment 1 | Experiment 2 | Experiment 3 | Average (%) | |
pSI01 | 21 | 19 | 20 | 20 |
pSI01∆relE | 79 | 81 | 80 | 80 |
Experiment 1 | Experiment 2 | Experiment 3 | Average (%) | |
pSI01 | 21 | 19 | 20 | 20 |
pSI01∆relE | 79 | 81 | 80 | 80 |
Discussion
We have shown that tet(S) is carried on a novel, small, low copy number plasmid in S. infantis C.MI.8 isolated from the human oral cavity. The rep of the plasmid is related to the Rep3 superfamily and the tet(S) itself is flanked by directly repeated IS1216 copies. The plasmid is predicted to have a copy number of 2–3 per cell and confers high-level minocycline resistance upon its host.
In the original host strain S. infantis C.MI.8, an infrequent recombination event can occur that allows the region of the plasmid containing tet(S), orf6 and one copy of IS1216 to excise from the plasmid and form a small circular molecule, whereas the remainder of pSI01 circularises but no longer contains a copy of tet(S) (Fig. 1b and c). This has important implications for the dissemination of tet(S); many different genetic platforms containing tet(S) have been found, for example in Enterococcus sp. (Novais et al., 2012), and it is likely that tet(S) has transferred from the conjugative transposon Tn6000, or a derivative, to other DNA molecules catalysed by recombination reactions involving IS1216 which are very often found associated with it. Additionally, the observation of circular molecules containing resistance genes and single copies of IS elements (or other repeat sequences which flank resistance genes) has been made previously. The tetracycline resistance gene tet(W), for example, has been detected on circular molecules in both Butyrivibrio fibrisolvens (Kazimierczak et al., 2006) and Rothia sp. (Villedieu et al., 2007). Various IS elements, including IS1216, have been shown to be responsible for the formation of circular molecules containing resistance genes, for example the multidrug resistance gene cfr in Enterococcus spp. (Liu et al., 2012a, 2013) and S. suis (Wang et al., 2013) and the antiseptic resistance gene qrg in Streptococcus oralis (Ciric et al., 2011). Our observation of the circular molecule containing tet(S) and IS1216 extends the number of resistance genes shown to exist as nonreplicative circular forms and supports the view that these ‘unconventional circularisable structures’ (Palmieri et al., 2013) may be an evolutionarily important mechanism for the dissemination of resistance genes between different replicons.
pSI01 encodes a TA addiction module with high similarity to the relBE module found in the plasmid pFW213 of S. parasanguinis. TA addiction modules function to prevent the loss of the element on which they are encoded. After constructing a mutant pSI01 that does not contain the relE toxin component, stability was tested in S. australis FRStet12. We could not find any difference in the stability of pSI01∆relE compared to pSI01 over a 10-day period of continuous subculturing.
During the stability assays of pSI01 and pSI01∆relE in S. australis FRStet12, it was observed occasionally that PCRs on colonies were positive for tet(S), whereas they were negative for the plasmid backbone. It is hypothesised that after recombination the circular element containing tet(S) has integrated into the chromosome in these colonies. These pSI01 backbone negative colonies were only found in the cultures that originally contained pSI01∆relE, which may indicate that plasmid stability is mildly affected or the recombination events take place somewhat more often for the mutant plasmid.
A competition assay was set up between S. australis FRStet12 pSI01 and FRStet12 pSI01∆relE. The starter culture for the assay contained equal numbers of both cell types but after 5 h 80% of the mixed cell culture consisted of FRStet12 pSI01∆relE. These results indicate that the toxin mutant strain had a growth advantage over the strain containing the wild-type pSI01, which indicates that the production of the toxin itself may have a considerable cost to the cell or that the effects of the toxin, despite the presence of the antitoxin, slow down the growth rate of the host cells.
In conclusion, we have determined the genetic basis of minocycline resistance in a human-derived oral S. infantis isolate. We have shown that it is due to tet(S) present on a novel low copy number plasmid flanked by IS1216 elements. It is capable of excising, together with orf6 and one of the IS1216 into a free, presumably nonreplicative circular form. We suspect that this type of recombination between IS1216 elements associated with tet(S) is aiding the dissemination of tet(S) amongst different DNA molecules.
Acknowledgements
This research was funded by the Commission of the European Communities, specifically the Infectious Diseases research domain of the Health theme of the 7th Framework Programme, contract 241446, ‘The effects of antibiotic administration on the emergence and persistence of antibiotic-resistant bacteria in humans and on the composition of the indigenous microbiotas at various body sites’. The authors have no conflict of interest to declare.
References
Author notes
Present addresses: Lena Ciric, Department of Civil, Environmental and Geomatic Engineering, University College London, Gower Street, London WC1E 6BT, UK Michael S. M. Brouwer, Central Veterinary Institute of Wageningen UR, PO Box 65, 8200 AB, Lelystad, The Netherlands
Editor: Stefan Schwarz