-
PDF
- Split View
-
Views
-
Cite
Cite
Tina Mygind, Svend Birkelund, Gunna Christiansen, Characterization of the variability of a 75-kDa membrane protein in Mycoplasma hominis, FEMS Microbiology Letters, Volume 190, Issue 1, September 2000, Pages 167–176, https://doi.org/10.1111/j.1574-6968.2000.tb09281.x
- Share Icon Share
Abstract
The gene p75 encoding a 75-kDa surface-exposed membrane protein P75 was cloned and sequenced from Mycoplasma hominis type strain PG21T. To investigate the intraspecies variability, sequences were obtained from an additional two isolates 7488 and 183, and the three sequences were compared. The nucleotide and amino acid differences were not confined to specific regions of the gene/protein, but when comparing the three sequences, differences were present as single site substitutions or small insertions or deletions of nucleotides/amino acids. The intraspecies variability was further investigated by restriction enzyme analysis with two restriction enzymes (AluI and MboII) of PCR products amplified from p75 from 28 M. hominis isolates. On the basis of band patterns produced by the two restriction enzymes, the isolates could be divided into five and six groups. These groups neither matched categories of the M. hominis vaa gene nor the M. hominis p120 gene classes, indicating that the three genes vary by different mechanisms and possibly indicating horizontal gene transfer.
1 Introduction
Mycoplasmas are among the smallest prokaryotes with a cell diameter of approximately 300 nm [1] and genome sizes, ranging from 580 kb in Mycoplasma genitalium[2] to 1300 kb in Mycoplasma iowae[3]. The mycoplasmas are distinct from other eubacteria in, for example, their lack of a cell wall, presence of cholesterol in their membrane, their tendency to form fried-egg colonies on solid medium and their often A-T rich genome.
Mycoplasma hominis is part of the normal genital flora of humans [4], but it has been associated with various infections. Isolation of M. hominis from the brain tissue and cerebrospinal fluid of a full-term newborn with meningitis have been reported [5], but more frequently it has been found that women colonized with M. hominis showing signs of bacterial vaginosis have an increased risk of preterm delivery and low birth weight infants [6]. So far, no specific pathogenicity factors have been discovered for M. hominis.
In the sequenced genomes of Mycoplasma pneumoniae and Mycoplasma genitalium[2,7] a substantial number of putative lipoproteins was present: 46 in M. pneumoniae (6.8% of the ORFs); and 21 in M. genitalium (4.5% of the ORFs). These are large proportions of the genomes and it is likely that these putative lipoproteins play an important role in the survival of the organisms [8]. The number of putative lipoproteins found in M. pneumoniae and M. genitalium suggests that there are probably still many surface proteins yet to be explored in M. hominis. Most of the M. hominis surface proteins investigated previously (Vaa, Lmp and P120) vary in size, sequence, antigenicity and expression [9–11]. The lmp genes encoding the Lmp proteins were shown to contain repeated elements, which is the basis for their variation in size [10]. The p120 gene and the resulting P120 protein has a hypervariable domain and two semivariable domains. Eighteen M. hominis isolates were divided into four classes on the basis of PCR-RFLP on the hypervariable domain. Four isolates were, by DNA sequencing, found to have pairwise identical hypervariable domains; 7488/DC63 and SC4/P2. They were speculated to have arisen by horizontal gene transfer [11]. The Vaa protein has a modular composition. Interchangeable cassettes cause differences between different isolates possibly by site-specific recombination with extrachromosomal DNA imported by the cells [9]. On the basis of these differences, isolates were divided into five categories.
In an earlier study by Christiansen et al. [12], a monoclonal antibody MAb 43.2 was produced against proteins from the M. hominis type strain PG21T. MAb 43.2 reacts with a protein which was calculated to have a size of approximately 70 kDa in the 26 M. hominis isolates investigated [12]. The protein has a hydrophobic part because it is present in the hydrophobic phase produced by Triton X-114 partitioning of whole cell PG21T. Furthermore, it is present at the surface of M. hominis as demonstrated by immunoelectron microscopy. The protein will be referred to as P75, and the corresponding gene will be referred to as p75.
Antibodies reacting with other M. hominis surface proteins investigated give variable reaction patterns (variation in band sizes, several bands or no bands) [12], so it seems likely that P75 is more conserved compared to other surface proteins investigated. Because of conservation of size and of the presence of P75 in all isolates investigated, it might have a function different from the surface antigens previously investigated in M. hominis. The objective of this study is to clone the p75 gene and to characterize the intraspecies variability of p75/P75.
2 Materials and methods
2.1 Selection and cultivation of M. hominis isolates and isolation of genomic DNA
The M. hominis isolates selected for this study are shown in Table 1. The isolates are obtained from a wide variety of geographical and different anatomical sites. The isolates comprising selection group I are isolates investigated previously [13]. These isolates are included to provide a basis for comparison of data. The isolates in selection group II in immunoblotting with MAb 43.2 react with proteins exhibiting small size variations [12]. Selection group III contains representatives of successive isolates from a patient with chronic septic arthritis [14]. Selection group IV contains isolates from the study by Thorsen et al. [15] and isolates from the the study by Boesen et al. [9], for a complete reference list for the isolates see [9].
Isolate | Anatomical site of isolation | Selection group | Geographical site of isolation |
PG21T | Lower genital | I | England |
7488 | Cervix | I | Aarhus, Denmark |
SC4 | Male urethra | I | England |
P2 | Upper urinary | I | Aarhus, Denmark |
183 | Vagina | I | USA |
10 | Vagina | I | USA |
DC63 | Oral cavity | I/II | England |
V2785 | Oral cavity | II | England |
5503 | Cervix | II | Aarhus, Denmark |
3105 | Cervix | II | Aarhus, Denmark |
132 | Vagina | II | USA |
1621 | Synovial fluid | III | USA |
1630 | Synovial fluid | III | USA |
2101 | Synovial fluid | III | USA |
2126 | Synovial fluid | III | USA |
P71 | Upper urinary | IV | Aarhus, Denmark |
M1449 | blood | IV | Denmark |
93 | Vagina | IV | USA |
P7 | Upper urinary | IV | Aarhus, Denmark |
W2 | Wound | IV | USA |
4712 | Cervix | IV | Aarhus, Denmark |
1893 | Cervix | IV | Aarhus, Denmark |
7808 | Cervix | IV | Aarhus, Denmark |
3849 | Cervix | IV | Aarhus, Denmark |
7357 | Cervix | IV | Aarhus, Denmark |
6188 | Cervix | IV | Aarhus, Denmark |
5941 | Cervix | IV | Aarhus, Denmark |
1935B | Cervix | IV | Aarhus, Denmark |
2347B | Cervix | IV | Aarhus, Denmark |
1572B | Cervix | IV | Aarhus, Denmark |
2032B | Cervix | IV | Aarhus, Denmark |
Isolate | Anatomical site of isolation | Selection group | Geographical site of isolation |
PG21T | Lower genital | I | England |
7488 | Cervix | I | Aarhus, Denmark |
SC4 | Male urethra | I | England |
P2 | Upper urinary | I | Aarhus, Denmark |
183 | Vagina | I | USA |
10 | Vagina | I | USA |
DC63 | Oral cavity | I/II | England |
V2785 | Oral cavity | II | England |
5503 | Cervix | II | Aarhus, Denmark |
3105 | Cervix | II | Aarhus, Denmark |
132 | Vagina | II | USA |
1621 | Synovial fluid | III | USA |
1630 | Synovial fluid | III | USA |
2101 | Synovial fluid | III | USA |
2126 | Synovial fluid | III | USA |
P71 | Upper urinary | IV | Aarhus, Denmark |
M1449 | blood | IV | Denmark |
93 | Vagina | IV | USA |
P7 | Upper urinary | IV | Aarhus, Denmark |
W2 | Wound | IV | USA |
4712 | Cervix | IV | Aarhus, Denmark |
1893 | Cervix | IV | Aarhus, Denmark |
7808 | Cervix | IV | Aarhus, Denmark |
3849 | Cervix | IV | Aarhus, Denmark |
7357 | Cervix | IV | Aarhus, Denmark |
6188 | Cervix | IV | Aarhus, Denmark |
5941 | Cervix | IV | Aarhus, Denmark |
1935B | Cervix | IV | Aarhus, Denmark |
2347B | Cervix | IV | Aarhus, Denmark |
1572B | Cervix | IV | Aarhus, Denmark |
2032B | Cervix | IV | Aarhus, Denmark |
Isolate | Anatomical site of isolation | Selection group | Geographical site of isolation |
PG21T | Lower genital | I | England |
7488 | Cervix | I | Aarhus, Denmark |
SC4 | Male urethra | I | England |
P2 | Upper urinary | I | Aarhus, Denmark |
183 | Vagina | I | USA |
10 | Vagina | I | USA |
DC63 | Oral cavity | I/II | England |
V2785 | Oral cavity | II | England |
5503 | Cervix | II | Aarhus, Denmark |
3105 | Cervix | II | Aarhus, Denmark |
132 | Vagina | II | USA |
1621 | Synovial fluid | III | USA |
1630 | Synovial fluid | III | USA |
2101 | Synovial fluid | III | USA |
2126 | Synovial fluid | III | USA |
P71 | Upper urinary | IV | Aarhus, Denmark |
M1449 | blood | IV | Denmark |
93 | Vagina | IV | USA |
P7 | Upper urinary | IV | Aarhus, Denmark |
W2 | Wound | IV | USA |
4712 | Cervix | IV | Aarhus, Denmark |
1893 | Cervix | IV | Aarhus, Denmark |
7808 | Cervix | IV | Aarhus, Denmark |
3849 | Cervix | IV | Aarhus, Denmark |
7357 | Cervix | IV | Aarhus, Denmark |
6188 | Cervix | IV | Aarhus, Denmark |
5941 | Cervix | IV | Aarhus, Denmark |
1935B | Cervix | IV | Aarhus, Denmark |
2347B | Cervix | IV | Aarhus, Denmark |
1572B | Cervix | IV | Aarhus, Denmark |
2032B | Cervix | IV | Aarhus, Denmark |
Isolate | Anatomical site of isolation | Selection group | Geographical site of isolation |
PG21T | Lower genital | I | England |
7488 | Cervix | I | Aarhus, Denmark |
SC4 | Male urethra | I | England |
P2 | Upper urinary | I | Aarhus, Denmark |
183 | Vagina | I | USA |
10 | Vagina | I | USA |
DC63 | Oral cavity | I/II | England |
V2785 | Oral cavity | II | England |
5503 | Cervix | II | Aarhus, Denmark |
3105 | Cervix | II | Aarhus, Denmark |
132 | Vagina | II | USA |
1621 | Synovial fluid | III | USA |
1630 | Synovial fluid | III | USA |
2101 | Synovial fluid | III | USA |
2126 | Synovial fluid | III | USA |
P71 | Upper urinary | IV | Aarhus, Denmark |
M1449 | blood | IV | Denmark |
93 | Vagina | IV | USA |
P7 | Upper urinary | IV | Aarhus, Denmark |
W2 | Wound | IV | USA |
4712 | Cervix | IV | Aarhus, Denmark |
1893 | Cervix | IV | Aarhus, Denmark |
7808 | Cervix | IV | Aarhus, Denmark |
3849 | Cervix | IV | Aarhus, Denmark |
7357 | Cervix | IV | Aarhus, Denmark |
6188 | Cervix | IV | Aarhus, Denmark |
5941 | Cervix | IV | Aarhus, Denmark |
1935B | Cervix | IV | Aarhus, Denmark |
2347B | Cervix | IV | Aarhus, Denmark |
1572B | Cervix | IV | Aarhus, Denmark |
2032B | Cervix | IV | Aarhus, Denmark |
The isolates were cultivated in BEA medium [16] modified to contain only 0.3% arginine and genomic DNA isolated according to the method by McClenaghan et al. [17].
2.2 SDS–PAGE and immunoblotting
M. hominis cultures were harvested in the late exponential growth phase and the pellets resuspended in SDS sample buffer (62.5 mM Tris–HCl pH 6.8, 10% (v/v) glycerol, 2.3% (w/v) SDS, 5% (v/v) β-mercaptoethanol and 0.05% (w/v) bromophenol blue), heated to 100°C for 5 min, separated by SDS–PAGE (4–20% gradient) and then electrotransferred to nitrocellulose membranes (Schleicher and Schüll, Dassel, Germany). Coomassie blue staining and immunoblottings were performed as described by Birkelund and Andersen [18]. MAb 43.2 prepared as hybridoma cell supernatant [12] was diluted 1:3 and used as primary antibody. The secondary antibody used was alkaline phosphatase (AP)-conjugated goat anti-mouse IgG (Bio-Rad, Richmond, CA, USA) (diluted 1:2000).
2.3 PCR amplification of probe used in hybridizations
The expression clones pMHX 1-15c [12] and M1, both known to express a fusion protein reacting with MAb 43.2, were sequenced for selection of primers P3 and P5 (Table 2). PCR was performed on purified genomic PG21T DNA with primers P3 and P5 (Table 2) (400 bp PCR product). PCR was performed as described previously [13] except that only 2.5 U AmpliTaq (R) DNA polymerase were used. The PCR product was purified as described previously [13], and the PCR fragments were radioactively labelled and used as probes in colony blot hybridization and Southern blotting.
Nameb | Isolate | Position in p75a | Sequence 5′ to 3′ |
pEX-F | – | pEX bp 3138–3156 | CGACGGTTTCCATATCCCC |
pEX-R | – | pEX bp 3249–3262 | CTAGAGCCGGATCGATCCGGTC |
P1 (F) | PG21T | bp 417–447 | GCTACTTTCATCAAATGAAGGTAAAAACATC |
P2 (F) | PG21T | bp 565–596 | CAAGAAATAGCAAATTTAGAAAAGGGCCC |
P3 (R) | PG21T | bp 657–688 | CAGCTCTTAAATCTTTATAAGCAAAGTCAGAG |
P4 (R) | PG21T | bp 1241–1273 | CCAAAACGCTATCTCTTAATGTTCTAGTAAAA |
P5 (F) | PG21T | bp 289–313 | GAAACTGCGCAAGATATAAATGCAC |
P6 (F) | PG21T | bp 860–889 | CTGAATTAGACGTTAAATTTTTAGCAGCGC |
P7 (F) | PG21T | bp 591–625 | CTTTTAATTTAGTAAATTCTTTTGATTTTGGGCCC |
P8 (F) | PG21T | bp 1090–1123 | GATCAAATTTATGAATTAAATCGAAACATAGAAC |
P9 (F) | PG21T | bp 1433–1453 | GAAGCGCACCAACATTTGACG |
P10 (F) | PG21T | bp 1828–1857 | GAAGCAAGAATGTTAAAACCTTGAACAGAT |
P11 (R) | PG21T | bp 2110–2137c | GCCCAATAAATTGCATATTCATAGCAAC |
P12 (R) | PG21T | bp 1825–1853 | GTTCAAGGTTTTAACATTCTTGCTTCTTC |
P13 (R) | PG21T | bp 1499–1530 | GTTAACATCTGTTGATTTTAGATCGCTAGATA |
P14 (R) | PG21T | bp 289–313 | GTGGATTTATATCTTGCGCAGTTTC |
P15 (F) | PG21T | bp −213-(−180)d | CATTTAAACATTTGTCTCTTTAATAATTAAGCC |
P16 (F) | PG21T | bp 3–32 | GAAAATGAGAAAGAAATTGATTGCTTTATC |
P17 (R) | PG21T | bp 1912–1936 | GTTAATTAGTTCCTTCAAAGATTTGCTTAATTC |
P18 (F) | 7488 | bp 1258–1285 | CTAGAATCGTTAAGAGATACCATTTCGG |
P19 (R) | 7488 | bp 1593–1622 | GAAGTAACAACCAAGTTGTAATCCTTTTCC |
P20 (R) | 7488 | bp 1258–1285 | CCGAAATGGTATCTCTTAACGATTCTAG |
P21 (R) | 7488 | bp 989–920 | GCTTGGCTTAATTCGTCATGCGC |
P22 (R) | 183 | bp 1512–1545 | GTTAACATCTGCTGATTTTAGATCAGTAGATTTT |
P23 (F) | 183 | bp 1102–1135 | GATCAAATTTATGAATTAAGTGGAGACATAGAAC |
P24 (R) | 183 | bp 289–320 | GAAATTTGTGCATTTATATCTTGTGTAGTTTC |
P25 (R) | 183 | bp 806–835 | CTAGTATTGACTTTTTGATGTTGGCAATTC |
P26 (F) | 183 | bp 1514–1545 | AATCTAGTGATCTAAAATCAGCAGATGTTAAC |
Nameb | Isolate | Position in p75a | Sequence 5′ to 3′ |
pEX-F | – | pEX bp 3138–3156 | CGACGGTTTCCATATCCCC |
pEX-R | – | pEX bp 3249–3262 | CTAGAGCCGGATCGATCCGGTC |
P1 (F) | PG21T | bp 417–447 | GCTACTTTCATCAAATGAAGGTAAAAACATC |
P2 (F) | PG21T | bp 565–596 | CAAGAAATAGCAAATTTAGAAAAGGGCCC |
P3 (R) | PG21T | bp 657–688 | CAGCTCTTAAATCTTTATAAGCAAAGTCAGAG |
P4 (R) | PG21T | bp 1241–1273 | CCAAAACGCTATCTCTTAATGTTCTAGTAAAA |
P5 (F) | PG21T | bp 289–313 | GAAACTGCGCAAGATATAAATGCAC |
P6 (F) | PG21T | bp 860–889 | CTGAATTAGACGTTAAATTTTTAGCAGCGC |
P7 (F) | PG21T | bp 591–625 | CTTTTAATTTAGTAAATTCTTTTGATTTTGGGCCC |
P8 (F) | PG21T | bp 1090–1123 | GATCAAATTTATGAATTAAATCGAAACATAGAAC |
P9 (F) | PG21T | bp 1433–1453 | GAAGCGCACCAACATTTGACG |
P10 (F) | PG21T | bp 1828–1857 | GAAGCAAGAATGTTAAAACCTTGAACAGAT |
P11 (R) | PG21T | bp 2110–2137c | GCCCAATAAATTGCATATTCATAGCAAC |
P12 (R) | PG21T | bp 1825–1853 | GTTCAAGGTTTTAACATTCTTGCTTCTTC |
P13 (R) | PG21T | bp 1499–1530 | GTTAACATCTGTTGATTTTAGATCGCTAGATA |
P14 (R) | PG21T | bp 289–313 | GTGGATTTATATCTTGCGCAGTTTC |
P15 (F) | PG21T | bp −213-(−180)d | CATTTAAACATTTGTCTCTTTAATAATTAAGCC |
P16 (F) | PG21T | bp 3–32 | GAAAATGAGAAAGAAATTGATTGCTTTATC |
P17 (R) | PG21T | bp 1912–1936 | GTTAATTAGTTCCTTCAAAGATTTGCTTAATTC |
P18 (F) | 7488 | bp 1258–1285 | CTAGAATCGTTAAGAGATACCATTTCGG |
P19 (R) | 7488 | bp 1593–1622 | GAAGTAACAACCAAGTTGTAATCCTTTTCC |
P20 (R) | 7488 | bp 1258–1285 | CCGAAATGGTATCTCTTAACGATTCTAG |
P21 (R) | 7488 | bp 989–920 | GCTTGGCTTAATTCGTCATGCGC |
P22 (R) | 183 | bp 1512–1545 | GTTAACATCTGCTGATTTTAGATCAGTAGATTTT |
P23 (F) | 183 | bp 1102–1135 | GATCAAATTTATGAATTAAGTGGAGACATAGAAC |
P24 (R) | 183 | bp 289–320 | GAAATTTGTGCATTTATATCTTGTGTAGTTTC |
P25 (R) | 183 | bp 806–835 | CTAGTATTGACTTTTTGATGTTGGCAATTC |
P26 (F) | 183 | bp 1514–1545 | AATCTAGTGATCTAAAATCAGCAGATGTTAAC |
aThe two first primers have positions given in the pEX vector.
bThe F's and R's in the brackets indicate that the primer is forward or reverse.
cThe primer is situated after the p75 stop codon.
dThe primer is situated upstream the p75 start codon.
Nameb | Isolate | Position in p75a | Sequence 5′ to 3′ |
pEX-F | – | pEX bp 3138–3156 | CGACGGTTTCCATATCCCC |
pEX-R | – | pEX bp 3249–3262 | CTAGAGCCGGATCGATCCGGTC |
P1 (F) | PG21T | bp 417–447 | GCTACTTTCATCAAATGAAGGTAAAAACATC |
P2 (F) | PG21T | bp 565–596 | CAAGAAATAGCAAATTTAGAAAAGGGCCC |
P3 (R) | PG21T | bp 657–688 | CAGCTCTTAAATCTTTATAAGCAAAGTCAGAG |
P4 (R) | PG21T | bp 1241–1273 | CCAAAACGCTATCTCTTAATGTTCTAGTAAAA |
P5 (F) | PG21T | bp 289–313 | GAAACTGCGCAAGATATAAATGCAC |
P6 (F) | PG21T | bp 860–889 | CTGAATTAGACGTTAAATTTTTAGCAGCGC |
P7 (F) | PG21T | bp 591–625 | CTTTTAATTTAGTAAATTCTTTTGATTTTGGGCCC |
P8 (F) | PG21T | bp 1090–1123 | GATCAAATTTATGAATTAAATCGAAACATAGAAC |
P9 (F) | PG21T | bp 1433–1453 | GAAGCGCACCAACATTTGACG |
P10 (F) | PG21T | bp 1828–1857 | GAAGCAAGAATGTTAAAACCTTGAACAGAT |
P11 (R) | PG21T | bp 2110–2137c | GCCCAATAAATTGCATATTCATAGCAAC |
P12 (R) | PG21T | bp 1825–1853 | GTTCAAGGTTTTAACATTCTTGCTTCTTC |
P13 (R) | PG21T | bp 1499–1530 | GTTAACATCTGTTGATTTTAGATCGCTAGATA |
P14 (R) | PG21T | bp 289–313 | GTGGATTTATATCTTGCGCAGTTTC |
P15 (F) | PG21T | bp −213-(−180)d | CATTTAAACATTTGTCTCTTTAATAATTAAGCC |
P16 (F) | PG21T | bp 3–32 | GAAAATGAGAAAGAAATTGATTGCTTTATC |
P17 (R) | PG21T | bp 1912–1936 | GTTAATTAGTTCCTTCAAAGATTTGCTTAATTC |
P18 (F) | 7488 | bp 1258–1285 | CTAGAATCGTTAAGAGATACCATTTCGG |
P19 (R) | 7488 | bp 1593–1622 | GAAGTAACAACCAAGTTGTAATCCTTTTCC |
P20 (R) | 7488 | bp 1258–1285 | CCGAAATGGTATCTCTTAACGATTCTAG |
P21 (R) | 7488 | bp 989–920 | GCTTGGCTTAATTCGTCATGCGC |
P22 (R) | 183 | bp 1512–1545 | GTTAACATCTGCTGATTTTAGATCAGTAGATTTT |
P23 (F) | 183 | bp 1102–1135 | GATCAAATTTATGAATTAAGTGGAGACATAGAAC |
P24 (R) | 183 | bp 289–320 | GAAATTTGTGCATTTATATCTTGTGTAGTTTC |
P25 (R) | 183 | bp 806–835 | CTAGTATTGACTTTTTGATGTTGGCAATTC |
P26 (F) | 183 | bp 1514–1545 | AATCTAGTGATCTAAAATCAGCAGATGTTAAC |
Nameb | Isolate | Position in p75a | Sequence 5′ to 3′ |
pEX-F | – | pEX bp 3138–3156 | CGACGGTTTCCATATCCCC |
pEX-R | – | pEX bp 3249–3262 | CTAGAGCCGGATCGATCCGGTC |
P1 (F) | PG21T | bp 417–447 | GCTACTTTCATCAAATGAAGGTAAAAACATC |
P2 (F) | PG21T | bp 565–596 | CAAGAAATAGCAAATTTAGAAAAGGGCCC |
P3 (R) | PG21T | bp 657–688 | CAGCTCTTAAATCTTTATAAGCAAAGTCAGAG |
P4 (R) | PG21T | bp 1241–1273 | CCAAAACGCTATCTCTTAATGTTCTAGTAAAA |
P5 (F) | PG21T | bp 289–313 | GAAACTGCGCAAGATATAAATGCAC |
P6 (F) | PG21T | bp 860–889 | CTGAATTAGACGTTAAATTTTTAGCAGCGC |
P7 (F) | PG21T | bp 591–625 | CTTTTAATTTAGTAAATTCTTTTGATTTTGGGCCC |
P8 (F) | PG21T | bp 1090–1123 | GATCAAATTTATGAATTAAATCGAAACATAGAAC |
P9 (F) | PG21T | bp 1433–1453 | GAAGCGCACCAACATTTGACG |
P10 (F) | PG21T | bp 1828–1857 | GAAGCAAGAATGTTAAAACCTTGAACAGAT |
P11 (R) | PG21T | bp 2110–2137c | GCCCAATAAATTGCATATTCATAGCAAC |
P12 (R) | PG21T | bp 1825–1853 | GTTCAAGGTTTTAACATTCTTGCTTCTTC |
P13 (R) | PG21T | bp 1499–1530 | GTTAACATCTGTTGATTTTAGATCGCTAGATA |
P14 (R) | PG21T | bp 289–313 | GTGGATTTATATCTTGCGCAGTTTC |
P15 (F) | PG21T | bp −213-(−180)d | CATTTAAACATTTGTCTCTTTAATAATTAAGCC |
P16 (F) | PG21T | bp 3–32 | GAAAATGAGAAAGAAATTGATTGCTTTATC |
P17 (R) | PG21T | bp 1912–1936 | GTTAATTAGTTCCTTCAAAGATTTGCTTAATTC |
P18 (F) | 7488 | bp 1258–1285 | CTAGAATCGTTAAGAGATACCATTTCGG |
P19 (R) | 7488 | bp 1593–1622 | GAAGTAACAACCAAGTTGTAATCCTTTTCC |
P20 (R) | 7488 | bp 1258–1285 | CCGAAATGGTATCTCTTAACGATTCTAG |
P21 (R) | 7488 | bp 989–920 | GCTTGGCTTAATTCGTCATGCGC |
P22 (R) | 183 | bp 1512–1545 | GTTAACATCTGCTGATTTTAGATCAGTAGATTTT |
P23 (F) | 183 | bp 1102–1135 | GATCAAATTTATGAATTAAGTGGAGACATAGAAC |
P24 (R) | 183 | bp 289–320 | GAAATTTGTGCATTTATATCTTGTGTAGTTTC |
P25 (R) | 183 | bp 806–835 | CTAGTATTGACTTTTTGATGTTGGCAATTC |
P26 (F) | 183 | bp 1514–1545 | AATCTAGTGATCTAAAATCAGCAGATGTTAAC |
aThe two first primers have positions given in the pEX vector.
bThe F's and R's in the brackets indicate that the primer is forward or reverse.
cThe primer is situated after the p75 stop codon.
dThe primer is situated upstream the p75 start codon.
2.4 Cloning of M. hominis DNA
Southern blotting was performed by standard methods [19]. M. hominis genomic DNA (2.8 μg for each cleavage) was cleaved with 60 U BglII, 60 U HindIII or 30 U ClaI plus 30 U HindIII. pBluescript SK+ vector DNA (Stratagene, La Jolla, CA, USA) (1.2 μg for each cleavage) was cleaved with 20 U BamHI (compatible ends with BglII), 20 U HindIII or 10 U ClaI plus 10 U HindIII. The remaining cloning procedure was essentially performed by standard procedures [19]. Enzymes and buffers were purchased from Roche, Mannheim, Germany. The plasmids were introduced to E. coli XL1-Blue competent cells (Stratagene) by electroporation [19]. E. coli XL1-Blue were cultivated in Luria–Bertani broth or Terrific broth [19]. The electrotransformed E. coli XL1-Blue colonies were subjected to colony blot hybridization as described by Sambrook et al. [19]. Specific p75 probes used were amplified by PCR as described below and labelled radioactively with α-32P-dATP by nick translation [19]. Plasmid DNA was prepared by the standard alkaline lysis method except that phenol/chloroform extraction was omitted [19].
The pEX clone M1 containing p75 DNA was obtained by screening of a pEX expression library with MAb 43.2 to search for E. coli clones containing Sau3AI cleaved PG21T DNA fragments with p75 gene sequence as described by Christiansen et al. [12].
2.5 High fidelity PCR to amplify the p75 gene
High fidelity PCR was performed on M. hominis genomic DNA from the selected isolates with the Expand™ High Fidelity PCR system from Roche. Primers P11 and P16 (see Table 2) (2135-bp PCR product) were used to amplify the p75 gene. High Fidelity PCR was performed in a total volume of 50 μl with 0.75 μl enzyme mix (3.5×103 U ml−1), 0.2 mM of each of dATP, dTTP, dGTP and dCTP, 15 pmol of each primer, 5 μl Expand HF buffer with 15 mM MgCl2, 0.1 μg purified genomic DNA and ddH2O up to 50 μl. The following cycling conditions were used: denaturation, 94°C for 5 min, 10 cycles of 92°C for 10 s, 55°C for 30 s, 68°C for 10 min, 20 cycles of 92°C for 10 s, 55°C for 30 s, 68°C for 10 min with 20 s added per cycle, and last 68°C for 20 min.
For sequencing, PCR products were purified as described previously [13]. PCR products and purifications were examined by electrophoresis in 0.7% agarose gels.
2.6 Restriction endonuclease analysis
The PCR products of p75 from the selected isolates were cleaved with AluI (Roche) and MboII (New England Biolabs, Herts, UK). The digests were analysed by agarose gel eletrophoresis in 2% gels and the resulting fragment patterns were used to divide the isolates into groups.
2.7 Pulsed-field gel electrophoresis (PFGE)
The p75 gene was mapped to existing genome maps of five M. hominis strains; PG21T, 132, 4195, 93 and 7488. This was done by PFGE of restriction enzyme digested genomic M. hominis DNA and Southern hybridization as described by Ladefoged et al. [20]. The probe was amplified by PCR as described above.
2.8 DNA sequencing and sequence analysis
DNA sequencing was performed as described previously [13] with the primers in Table 2 (the primers were synthesized at DNA Technology, Aarhus, Denmark). The sequences were assembled and analysed with software from the Wisconsin Package Version 9.1-unix from the Genetics Computer Group (GCG), Madison, WI, USA [21]. For comparison of DNA and protein sequences the programs GAP (with the option -RAN) and PILEUP (multiple alignment) were used. To create a hydrophobicity plot the programs PEPTIDESTRUCTURE and PLOTSTRUCTURE were used. For similarity searches in GenBank and Swissprot, the programs FASTA and BLAST were used. To predict secondary protein structure, the GORIV secondary structure prediction was used [22] (Website: http://pbil.ibcp.fr/). The sequences have been deposited in the EMBL database (http://www.embl-heidelberg.de/) under the accession numbers: PG21T, AJ243901; 7488, AJ243902; and 183, AJ243903.
3 Results and discussion
3.1 Cloning and sequencing of p75 for type strain PG21T
The DNA sequence of the p75 gene from PG21T (see Fig. 1) consists of 1947 nucleotides with 23% G+C, which translates into 649 amino acids.
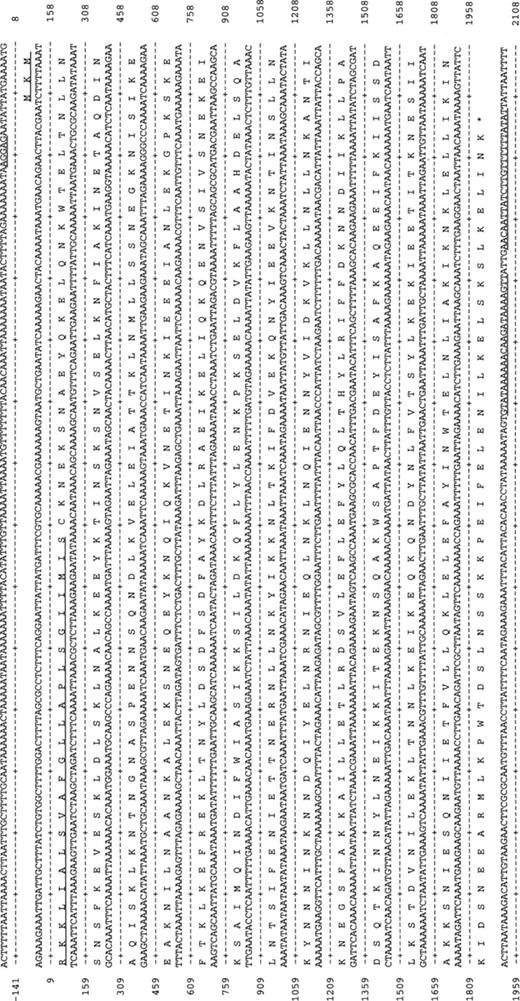
The DNA and protein sequence for p75 from PG21T. The putative Shine–Dalgarno sequence is underlined. The putative lipoprotein signal peptide is underlined. Downstream of the stop codon, a putative stem-loop structure is indicated by underlining.
A putative Shine–Dalgarno sequence was located 6 bp upstream the start codon and 18 bp downstream the TAA stop codon a putative terminator stem-loop structure was found (ΔG=−20.3 kcal mol−1) [23]. The protein sequence of P75 contains a high number of the amino acids isoleucine (10.5%) and leucine (12.5%), some of which may be part of a leucine zipper structure.
P75 contains a prokaryotic lipid attachment site [24], which indicates that it is cleaved by Signal Peptidase II and transported to the membrane. A hydrophobicity plot (Kyte–Doolittle algorithm) [25] showed that P75 contains no internal potential membrane-spanning hydrophobic regions, the only hydrophobic region was the signal peptide. These findings confirm those found by Christiansen et al. [12], as they determined the protein to be hydrophobic by Triton X-114 phase separation [12]. The molecular mass of P75 calculated from the sequence is 72.4 kDa (excluding the signal peptide), which correlates well with the molecular size determined by immunoblotting with MAb 43.2: 75.7 kDa.
In a similarity search on the P75 protein sequence using the program FASTA, the protein with highest similarity score was P72 from Mycoplasma mycoides subsp. mycoides[26]. It showed 25.2% identity to P75. The program GAP was run with the randomization option (100 randomizations). The quality of the normal alignment was calculated by GAP to be 49. The average quality for the 100 randomized alignments was 45.6±12.3 with a randomized P72 sequence and 40.1±12.1 with a randomized P75 sequence. The qualities for the randomized alignments were similar to or higher than the quality for the normal alignment; therefore, the homology found is probably not significant, but might be due to similar amino acid composition of the two proteins.
3.2 Intraspecies variation of p75/P75 investigated by DNA sequencing
Two additional M. hominis isolates were selected for sequencing of p75; 183 and 7488. The p75 genes from the two isolates were amplified by High Fidelity PCR, and the PCR products were sequenced bidirectionally. The three sequences were aligned with the program PILEUP. PG21T numbering is used for nucleotide and amino acid positions in the following text. The isolates 183 and 7488 have an insert of 12 bp after nucleotide no. 375 and an insert of 3 bp after nucleotide no. 1499. There were 104 polymorphic sites out of 1950 comparable nucleotides (the length of the shortest sequence), this makes 5.3 nucleotide substitutions per 100 bp. There were three sites at which the three sequences each had a different nucleotide (bp nos. 1083, 1439 and 1579). For the rest of the DNA sequence, the differences seemed to be spread evenly and there was no obvious clustering of the polymorphic sites.
Alignment of the protein sequences from PG21T, 183 and 7488 is shown in Fig. 2. Here it is seen that 7488 and 183 have an insert of four amino acids after aa no. 125 and an insert of one amino acid after aa no. 500, so the insertions at DNA level are in-frame. Furthermore, there are 69 sites with amino acid substitutions. With a comparable length of 649 amino acids, it makes 11 substitutions per 100 amino acids. It is notable that at six of the polymorphic sites, all the isolates have different amino acids (aa nos. 23, 99, 363, 480, 527 and 611), these may represent particularly variable sites. The differences in amino acids are also distributed over the entire sequence.
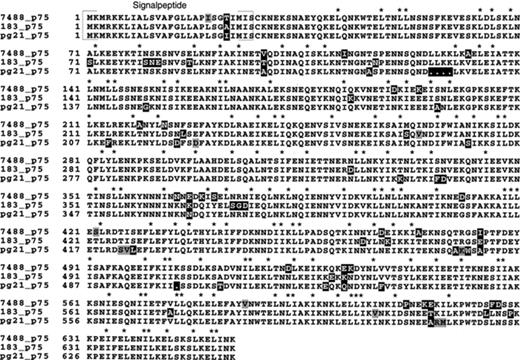
Multiple alignment of the P75 protein sequences from isolates 7488, 183 and PG21T showing the scattered polymorphic sites. The alignment was performed with the program PILEUP. Conserved isoleucines and leucines are indicated with asterisks. Gray boxes indicate amino acids with similar properties; black boxes indicate amino acids with different properties.
Eighty nucleotide differences out of a total of 104 nucleotide substitutions (77%) were non-synonymous. For the gap gene (a housekeeping gene that encodes the enzyme glyceraldehyde 3-phosphate dehydrogenase, which participates in glycolysis) when comparing sequences from eight isolates, 20% of the nucleotide substitutions were non-synonymous [27]. This probably reflects that the p75 gene is under a greater selective pressure or possibly that more variation is allowed in P75, because it could be an advantage to the cell. This is also reflected by the low G+C content of the p75 gene (23% G+C) compared to the gap gene (32% G+C) [27].
The secondary structure was predicted for the P75 sequences from 7488 and 183 with the GORIV secondary structure prediction [22]. The predicted P75 structures for the three isolates were mostly α-helical and virtually identical though small differences could be seen (results not shown). This in addition to the fact that the isoleucines/leucines are largely conserved among the three isolates (see Fig. 2) supports the idea that the protein is able to form leucine zippers.
3.3 Intraspecies variation of p75 investigated by PCR and RFLP
Primers P11 and P16 (Table 2) were used to amplify the p75 gene from 31 M. hominis isolates (Table 1) by High Fidelity PCR and PCR products of approximately 2 kb were visualized in an agarose gel containing ethidium bromide (results not shown). Three isolates, 10, 7357 and V2785, showed only very low amounts of PCR products by amplification with the two primers, and as a consequence, they were excluded from the next step. This indicates that some variation might be present at the primer sites.
The PCR products from the remaining 28 isolates were cleaved with the restriction enzymes AluI and MboII and the fragments visualized on 2% agarose gels (Fig. 3). AluI and MboII were chosen because four or more of these restriction enzyme sites were present in the PG21T sequence. Furthermore, MboII was chosen to give larger resolution as it cleaves more times than AluI. For each of the restriction enzyme analyses (REAs) the isolates were divided into groups according to their band pattern. In Table 3A, the RFLP groups are shown. Group 1 isolates exhibited individual band patterns. Isolates categorized to the remaining RFLP groups have identical band patterns. The two sets of RFLP groups for the PCR products do not correspond entirely, nevertheless some isolates seem to be grouping together with both restriction enzymes. The isolates 1621, 1630, 2101, 2126 and 2032B are in the same group with both restriction enzymes, so are SC4, P2, P7 and P71; DC63, 5503 and 132; and M1449 and 1572B. The rest of the isolates varied individually. The isolates 1621, 1630, 2101 and 2126 were all consecutive isolates from the knee of one patient [14], so they were expected to be closely related [14,28], but their apparent relation to 2032B was unexpected, as 2032B was isolated from a different person and on a different continent [15]. The fact that the two sets of RFLP groups do not correspond entirely is probably partly due to the fact that MboII cleaves more times than AluI and thereby a larger resolution is obtained. Furthermore, it should be noted that the PCR-RFLP not necessarily reflects the total variation when sequences are compared, because of the less resolution of this method. Therefore, the isolate groupings obtained by PCR-RFLP might not give a complete differentiation.
![(A) The PCR product from p75 cleaved with AluI. (B) The PCR product from p75 cleaved with MboII. Both are 2% agarose gels stained with ethidium bromide. (C) Genomic map of the type strain PG21T showing the position of known genes. The map has been redrawn from [27].](https://oup.silverchair-cdn.com/oup/backfile/Content_public/Journal/femsle/190/1/10.1111_j.1574-6968.2000.tb09281.x/1/m_FML_167_f3.jpeg?Expires=1750842101&Signature=px7sjqsA2bvosGVwiti~6~jGC6ydaKST8JA2-TeWBOl~qw1aVbxDlR~QiOU0PW97nE0o0QbgWQQ-LEn5thRuqtOosWL1Ov4wSPekNzb4nLoqI8uOk7W7m99i2Lcewn5e3OS-DjU0qlA7IU6fC1kc43X5y~PMcN3CvKg2FVlQCe~1cxUGKZWJVPIKZu95rT9bzmleitwMLgh~vf0j8~gaTk710g241rOesgPaj~RK1Lwarjv4j4ZGc8kT0jPzP2qvfltGFM-ugKakWRtQItq9q5xc7phNZPKipKGgo75uSU14wagxUzBlI8LhmiKAdlVzWButIaj64LYQPqBiBWiNDA__&Key-Pair-Id=APKAIE5G5CRDK6RD3PGA)
(A) The PCR product from p75 cleaved with AluI. (B) The PCR product from p75 cleaved with MboII. Both are 2% agarose gels stained with ethidium bromide. (C) Genomic map of the type strain PG21T showing the position of known genes. The map has been redrawn from [27].
RFLP groups of the 28 PCR products cleaved with two different restriction enzymes (A) and vaa categories for the 28 isolates [9] (B)
p75 RFLP group/vaa category [9] | Isolates |
(A) | |
AluI group 1 | W2, 7808, 3849, 5941, PG21T, 93, 4712, 1893, 2347B, 183 |
MboII group 1 | W2, 7808, 3849, 5941, 7488 |
AluI group 2 | 1621, 1630, 2101, 2126, 2032B, 7488 |
MboII group 2 | 1621, 1630, 2101, 2126, 2032B, PG21T, 183, 3105, 93, 6188 |
AluI group 3 | SC4, P2, P7, P71 |
MboII group 3 | SC4, P2, P7, P71, 1893 |
AluI group 4 | DC63, 5503, 132, 3105 |
MboII group 4 | DC63, 5503, 132, 1935B |
AluI group 5 | M1449, 1572B, 1935B, 6188 |
MboII group 5 | M1449, 1572B |
MboII group 6 | 4712, 2347B |
(B) | |
vaa category no. 1 | PG21T, P71, M1449, 93, 4712, 5503, 1893, 3105, 7488, 7808, 3849, 1935B, 2347B |
vaa category no. 2 | P7, 183, SC4, DC63, P2, W2, 132, 6188 |
vaa category no. 3 | 1572B, 2032B |
vaa category no. 4 | 1621, 1630, 2102, 2126 |
vaa category no. 5 | 5941 |
AluI group 1 and MboII group 1 contain isolates with individual band patterns. |
p75 RFLP group/vaa category [9] | Isolates |
(A) | |
AluI group 1 | W2, 7808, 3849, 5941, PG21T, 93, 4712, 1893, 2347B, 183 |
MboII group 1 | W2, 7808, 3849, 5941, 7488 |
AluI group 2 | 1621, 1630, 2101, 2126, 2032B, 7488 |
MboII group 2 | 1621, 1630, 2101, 2126, 2032B, PG21T, 183, 3105, 93, 6188 |
AluI group 3 | SC4, P2, P7, P71 |
MboII group 3 | SC4, P2, P7, P71, 1893 |
AluI group 4 | DC63, 5503, 132, 3105 |
MboII group 4 | DC63, 5503, 132, 1935B |
AluI group 5 | M1449, 1572B, 1935B, 6188 |
MboII group 5 | M1449, 1572B |
MboII group 6 | 4712, 2347B |
(B) | |
vaa category no. 1 | PG21T, P71, M1449, 93, 4712, 5503, 1893, 3105, 7488, 7808, 3849, 1935B, 2347B |
vaa category no. 2 | P7, 183, SC4, DC63, P2, W2, 132, 6188 |
vaa category no. 3 | 1572B, 2032B |
vaa category no. 4 | 1621, 1630, 2102, 2126 |
vaa category no. 5 | 5941 |
AluI group 1 and MboII group 1 contain isolates with individual band patterns. |
RFLP groups of the 28 PCR products cleaved with two different restriction enzymes (A) and vaa categories for the 28 isolates [9] (B)
p75 RFLP group/vaa category [9] | Isolates |
(A) | |
AluI group 1 | W2, 7808, 3849, 5941, PG21T, 93, 4712, 1893, 2347B, 183 |
MboII group 1 | W2, 7808, 3849, 5941, 7488 |
AluI group 2 | 1621, 1630, 2101, 2126, 2032B, 7488 |
MboII group 2 | 1621, 1630, 2101, 2126, 2032B, PG21T, 183, 3105, 93, 6188 |
AluI group 3 | SC4, P2, P7, P71 |
MboII group 3 | SC4, P2, P7, P71, 1893 |
AluI group 4 | DC63, 5503, 132, 3105 |
MboII group 4 | DC63, 5503, 132, 1935B |
AluI group 5 | M1449, 1572B, 1935B, 6188 |
MboII group 5 | M1449, 1572B |
MboII group 6 | 4712, 2347B |
(B) | |
vaa category no. 1 | PG21T, P71, M1449, 93, 4712, 5503, 1893, 3105, 7488, 7808, 3849, 1935B, 2347B |
vaa category no. 2 | P7, 183, SC4, DC63, P2, W2, 132, 6188 |
vaa category no. 3 | 1572B, 2032B |
vaa category no. 4 | 1621, 1630, 2102, 2126 |
vaa category no. 5 | 5941 |
AluI group 1 and MboII group 1 contain isolates with individual band patterns. |
p75 RFLP group/vaa category [9] | Isolates |
(A) | |
AluI group 1 | W2, 7808, 3849, 5941, PG21T, 93, 4712, 1893, 2347B, 183 |
MboII group 1 | W2, 7808, 3849, 5941, 7488 |
AluI group 2 | 1621, 1630, 2101, 2126, 2032B, 7488 |
MboII group 2 | 1621, 1630, 2101, 2126, 2032B, PG21T, 183, 3105, 93, 6188 |
AluI group 3 | SC4, P2, P7, P71 |
MboII group 3 | SC4, P2, P7, P71, 1893 |
AluI group 4 | DC63, 5503, 132, 3105 |
MboII group 4 | DC63, 5503, 132, 1935B |
AluI group 5 | M1449, 1572B, 1935B, 6188 |
MboII group 5 | M1449, 1572B |
MboII group 6 | 4712, 2347B |
(B) | |
vaa category no. 1 | PG21T, P71, M1449, 93, 4712, 5503, 1893, 3105, 7488, 7808, 3849, 1935B, 2347B |
vaa category no. 2 | P7, 183, SC4, DC63, P2, W2, 132, 6188 |
vaa category no. 3 | 1572B, 2032B |
vaa category no. 4 | 1621, 1630, 2102, 2126 |
vaa category no. 5 | 5941 |
AluI group 1 and MboII group 1 contain isolates with individual band patterns. |
The RFLP groups for the PCR product from p75 do not correspond to the vaa categories (Table 3B). This could be due to the horizontal gene transfer of vaa cassettes, suggested by Boesen et al. [9].
The p75 RFLP groups do not correspond to the p120 hypervariable domain classes either. The isolates DC63/7488 were shown to have identical hypervariable domains in p120[11]. These isolates were, in the present study, shown to be in different p75 RFLP groups. The identity in the p120 hypervariable domain is speculated to originate from horizontal gene transfer [11]. This hypothesis is supported by the fact that these two isolates differ in p75 RFLP groups, because the p75 gene is less variable than p120.
Regarding recombination and horizontal gene transfer, a phylogenetic analysis of p75, p120 and vaa sequences from many isolates could probably help to elucidate these issues. So far, no experimental approaches have proven that recombination and horizontal gene transfer occur between M. hominis isolates.
A further difference between p75 RFLP groups and vaa categories or p120 classes is that in the p75 RFLP groups, many isolates shows an individual pattern. It should be kept in mind though, that the vaa categories are based on PCR results and partial DNA sequencing of the genes and not RFLP results, therefore there actually are differences among isolates within vaa categories as homologous vaa modules show from 82–100% amino acid identity [9]. The p120 classes contain subclasses, therefore some of the isolates are individual here too [11]. Consequently, both vaa and p120 show major variation in the gene structure in addition to point mutations. It illustrates that vaa and p120 probably vary by both sequence variation, recombination and horizontal gene transfer, but p75 probably displays only sequence variation by point mutation. This means that there are clear differences in modes of variation between the three genes, but they all vary by point mutation presumably caused by the normal genetic drift in M. hominis. It probably reflects that the three genes are under different selective pressures.
3.4 Mapping of the p75 gene by PFGE and Southern hybridization
The p75 gene was mapped to the existing genome maps [20] of five M. hominis isolates by PFGE of restriction enzyme digested genomic DNA followed by Southern hybridization with a p75 specific probe. The p75 gene was mapped to the A fragment [20] with all restriction enzymes in all isolates. Consequently, the p75 gene was found in an 80-kb region in type strain PG21T, in which the p120, gyrB, rpoA[20] and vaa (Fig. 3C) genes are also present. In the four other isolates, p75 was also present near vaa and p120, gyrB and rpoA. As discussed above, the p75, p120 and vaa genes are very different; nevertheless, they are situated relatively close to each other on the genome. Consequently, their difference in mode of variation is probably not caused by differential development of distant genome regions.
Acknowledgments
This work was supported by the Danish Health Research Council (Grants 12-0850-1, 12-1620-1 and 9702071), Aarhus University Foundation, Novo Foundation and Fonden til Lægevidenskabens Fremme. We thank Karin S. Sørensen and Inger Andersen for excellent technical assistance and Lisbet Wellejus Pedersen for linguistic assistance.
References