-
PDF
- Split View
-
Views
-
Cite
Cite
Velislav N. Batchvarov, Marek Malik, A. John Camm, Incorrect electrode cable connection during electrocardiographic recording, EP Europace, Volume 9, Issue 11, November 2007, Pages 1081–1090, https://doi.org/10.1093/europace/eum198
- Share Icon Share
Abstract
Incorrect electrode cable connections during electrocardiographic (ECG) recording can simulate rhythm or conduction disturbance, myocardial ischaemia and infarction, as well as other clinically important abnormalities. When only precordial or only limb cables, excluding the neutral cable, have been interchanged the waveforms in the different leads are re-arranged, inverted, or unchanged, whereas the duration of intervals is not changed. The mistake can be recognized by the presence of unusual P–QRS patterns (e.g. negative P–QRS in lead I or II, positive in lead AVR, P–QRS complexes of opposite direction in leads I and V6, etc.), change in the P–QRS axis, or abnormal precordial QRS–T wave progression. Interchange of limb cables with the neutral cable distorts Wilson’s terminal and the morphology of all precordial and unipolar limb leads. The telltale sign of the mistake is the presence of (almost) a flat line in lead I, II or III. Interchange of even one of the limb cables, except for the neutral cable, with a precordial cable distorts the morphology of most leads and leaves not more than one lead (I, II, or III) unchanged. Computerized algorithms for detection of lead misplacement, such as those based on artificial neural networks, or on correlation between original and reconstructed leads, have been developed.
Introduction
It has been reported that 0.4–4% of all 12-lead electrocardiograms (ECG) acquired in various clinical settings have been recorded with incorrect connection of the electrode cables,1–3 with the highest figures reported for intensive care units.1 In absolute numbers this amounts to up to several hundred thousand out of the 40 million ECGs recorded annually in the United States.4 Cable misplacement can produce ECG abnormalities simulating ectopic rhythm, intraventricular conduction disturbance,5,6 chamber enlargement or ventricular pre-excitation,7 as well as simulating6,8–11 or concealing12 myocardial ischaemia or infarction. Although the most common cable reversals, such as interchange of the right arm (RA) and left arm (LA) cables are usually detected even by inexperienced ECG readers or standard computer programs for ECG interpretation, less frequent cable misplacements may be less obvious and often remain unnoticed both by man and computer.13 At times, the ECG with cable interchange may even look at a cursory glance ‘more normal’ than the correctly recorded one. With the exception of very few,14,15 most ECG textbooks briefly mention only the most obvious signs of lead misplacement, such as negative P–QRS complexes in lead I or II and positive complexes in augmented VR (AVR).
In order to find out which leads, if any, of the incorrectly recorded ECG are unaffected by the technical error and can still be used to interpret the ECG, it is necessary to decipher exactly which cables have been interchanged. This may sometimes be a challenging problem. Interchange of only precordial cables (e.g. V5 with V6) or only the RA, LA, or left leg (LL) cables (not involving the neutral electrode cable) does not affect the reference electrode for the unipolar* precordial leads (the so-called central or Wilson’s terminal*). In such cases, the waveforms in all leads are either unaffected but rearranged or only need to be re-labelled, whereas the duration of intervals and absolute amplitudes are not affected. On the other hand, when a peripheral cable has been interchanged with a precordial or with the right leg (neutral) cable, the shape and amplitude of the waveforms in most or all leads is affected and they cannot be used for precise morphological analysis or measurement of amplitude and intervals.
In the following text, we have tried to summarize the effect of the more common electrode cable misconnections on the normal 12-lead ECG and to present simple visual criteria for their detection. More sophisticated methods, such as those based on computer software algorithms are only briefly mentioned.
Our comments apply to standard ECG equipment that satisfies the common signal acquisition and processing criteria, namely that that resistor and amplifier setting of all three limb leads (i.e. RA, LA, and LL) are the same, and similarly that the resistor and amplifier setting of all precordial leads (i.e. leads V1–V6) are the same. This is not necessarily satisfied in some non-standard equipment and in such cases, lead interchanges may result in other technical abnormalities than those described in this text. We also assumed that the circuit setting of the grounding neutral electrode is such that the position of the electrode on the body is irrelevant.
Reversal of the left arm, right arm or left leg electrodes
Table 1 presents the changes resulting from the five possible interchanges of the lead cables not involving the neutral electrode cable.
ECG changes resulting from interchange of the ground cable and the other peripheral cables
Reversal . | Lead . | ||||||
---|---|---|---|---|---|---|---|
“I” . | “II” . | “III” . | “AVR” . | “AVL” . | “AVF” . | “V1–V6” . | |
LA/RA | −I | III | II | AVL | AVR | AVF | Unchanged |
LA/LL | II | I | −III | AVR | AVF | AVL | Unchanged |
RA/LL | −III | −II | −I | AVF | AVL | AVR | Unchanged |
Clockwise rotation | III | −I | −II | AVL | AVF | AVR | Unchanged |
Counter-clockwise rotation | −II | −III | I | AVF | AVR | AVL | Unchanged |
Reversal . | Lead . | ||||||
---|---|---|---|---|---|---|---|
“I” . | “II” . | “III” . | “AVR” . | “AVL” . | “AVF” . | “V1–V6” . | |
LA/RA | −I | III | II | AVL | AVR | AVF | Unchanged |
LA/LL | II | I | −III | AVR | AVF | AVL | Unchanged |
RA/LL | −III | −II | −I | AVF | AVL | AVR | Unchanged |
Clockwise rotation | III | −I | −II | AVL | AVF | AVR | Unchanged |
Counter-clockwise rotation | −II | −III | I | AVF | AVR | AVL | Unchanged |
RA—right arm; LA—left arm; LL—left leg; Clockwise rotation: RA → LA → LL → RA; counter-clockwise rotation: RA → LL → LA → RA. In quotation marks are cited the leads as they are labelled in the ECG recorded with interchanged cables. The (−) sign signifies that the respective lead is inverted upside down.
ECG changes resulting from interchange of the ground cable and the other peripheral cables
Reversal . | Lead . | ||||||
---|---|---|---|---|---|---|---|
“I” . | “II” . | “III” . | “AVR” . | “AVL” . | “AVF” . | “V1–V6” . | |
LA/RA | −I | III | II | AVL | AVR | AVF | Unchanged |
LA/LL | II | I | −III | AVR | AVF | AVL | Unchanged |
RA/LL | −III | −II | −I | AVF | AVL | AVR | Unchanged |
Clockwise rotation | III | −I | −II | AVL | AVF | AVR | Unchanged |
Counter-clockwise rotation | −II | −III | I | AVF | AVR | AVL | Unchanged |
Reversal . | Lead . | ||||||
---|---|---|---|---|---|---|---|
“I” . | “II” . | “III” . | “AVR” . | “AVL” . | “AVF” . | “V1–V6” . | |
LA/RA | −I | III | II | AVL | AVR | AVF | Unchanged |
LA/LL | II | I | −III | AVR | AVF | AVL | Unchanged |
RA/LL | −III | −II | −I | AVF | AVL | AVR | Unchanged |
Clockwise rotation | III | −I | −II | AVL | AVF | AVR | Unchanged |
Counter-clockwise rotation | −II | −III | I | AVF | AVR | AVL | Unchanged |
RA—right arm; LA—left arm; LL—left leg; Clockwise rotation: RA → LA → LL → RA; counter-clockwise rotation: RA → LL → LA → RA. In quotation marks are cited the leads as they are labelled in the ECG recorded with interchanged cables. The (−) sign signifies that the respective lead is inverted upside down.
Reversal of the RA and LA cables, which is the most frequent type of cable reversal, is easily recognizable during sinus rhythm by the presence of negative P and QRS waves in lead I, which never occurs in healthy hearts and is a very unusual finding in the presence of cardiac disease (usually chronic obstructive or other pulmonary disease, less frequently congenital heart disease14,19) (Figure 1). In the absence of P waves, a useful sign of this cable interchange is the negative QRS complex in lead I in the presence of mainly positive QRS in leads V5 and V6, which is physiologically very unlikely.14,20 When the RA and LA cables are interchanged, the morphology of the peripheral leads can resemble mirror-image dextrocardia; the cable interchange can be suspected by the unchanged precordial leads, whereas in mirror-image dextrocardia the QRS becomes smaller and exhibits mostly QS or rS shape in leads V3 through V6.14 Unlike RA/LA cable interchange, in congenitally corrected transposition, reversal of the precordial Q-wave pattern is observed (Q waves are present in the right precordial leads and are absent in the left21).
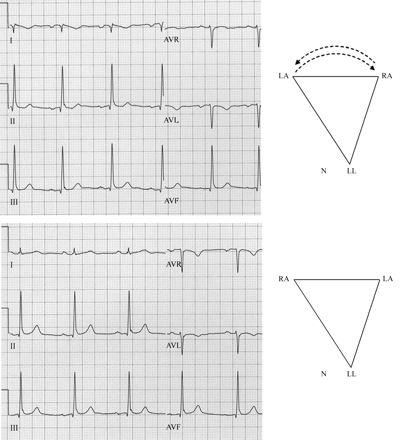
Peripheral leads of a 12-lead electrocardiogram recorded in a healthy young participant in a drug trial with right arm–left arm cable reversal (top panel) and with correct cable connections (bottom panel). In this one and in the subsequent figures, the positions of the peripheral electrodes and their effect on Einthoven’s triangle are schematically presented (in this figure to the right of each ECG). The cable reversal can be recognized by the negative P–QRS in lead I and positive P wave in lead AVR. Note, however, that the frontal QRS axis is very similar in the ECG and without cable reversal (96 and 86°, respectively). See the text for details.
Reversal of the RA and LL electrode cables can be easily spotted by the inverted P–QRS complex in lead II. This mistake can mimic inferior myocardial infarction as leads AVF and AVR are also interchanged. Unlike inferior infarction in sinus rhythm, however, the P wave in leads AVF and II is also inverted.
During sinus rhythm, reversal of the LA and LL electrode cables is frequently difficult or impossible to recognize, because when lead I becomes lead II, AVL and AVF are interchanged and lead III is inverted, the ECG may superficially look even ‘more normal’ than the correctly recorded one (Figure 2B). On the other hand, in the presence of typical atrial flutter, LA/LL cable interchange can easily be detected by the appearance of the sawtooth flutter waves in leads I, III, and AVL, but not in lead II.14 The observant ECG reader may even notice that the flutter waves in lead III are inverted (e.g. with the acute angles pointing upwards instead of downwards in case of typical (counter-clockwise, CCW) atrial flutter22). In such case, if the typical pattern was not observed previously, it would not be possible to exclude atypical flutter patterns. The inversion of lead III and interchange of leads AVF and AVL (in which the T wave can normally be inverted) can produce negative T waves in leads III and AVF, simulating inferior myocardial ischaemia.14 In a patient with inferior myocardial infarction, this cable reversal can cause the disappearance of the negative Q waves and T waves in the inferior leads.14
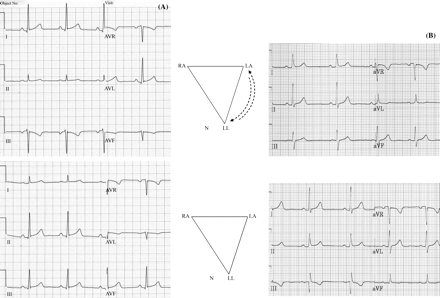
Changes in the morphology of the P wave can help to detect left arm–left leg cable reversal. The figure shows peripheral leads of 12-lead ECGs recorded in a 25-year old healthy participant in a drug trial (A) and in a 34-year-old man with mild arterial hypertension (B). The ECGs with left arm–left leg cable reversal are presented in the top panels, whereas those with correct cable connections are shown in the bottom panels. The positions of the peripheral electrodes with incorrect (top) and correct cable connections (bottom) are schematically presented in the middle panels.
Note that in both cases, the ECGs recorded with cable reversal look normal. In A, the clue to detect the mistake is the higher amplitude of the P wave in lead I than in lead II, which is abnormal,18 whereas in B the cable reversal can be suspected by the biphasic P wave with terminal positivity in lead III, which is also abnormal.18 See the text for details.
Abdollah and Milliken22 reported that in most cases, LA/LL cable reversal can be detected during sinus rhythm by careful analysis of the shape of the P wave, bearing in mind that normally the P wave is not higher in lead I than in lead II (which means that the frontal P axis should not be to the left of 30°) and should not have a terminal positive component in lead III. They found that the presence of either PI higher than PII (Figure 2A) or terminal positive PIII (Figure 2B) identified LA/LL cable reversal with 90% sensitivity. However, when these criteria were tested subsequently on more than 8000 ECGs with computationally generated LA/LL reversal (excluding ECGs without P waves), they yielded only 38% specificity at 90% level of sensitivity.23
Ho and Ho24 advanced this idea much further and developed an elaborate algorithm for detection of the five types of limb lead misplacement not involving the neutral electrode based on analysis of the P wave. Their method is based on the vectorcardiographic premise that the normal frontal plane P loop is (almost) invariably inscribed in the CCW rather than the CW direction. In ∼94% of all cases, the direction of P loop inscription (CCW or CW) may be discerned from the standard ECG by inspecting the P wave in simultaneously recorded three standard and three unipolar limb leads.24 For example, earlier occurrence of the P wave peak in lead III and AVF relative to lead I and AVL, respectively, signifies CCW inscription of the P loop, whereas biphasic negative–positive P wave in lead III reflects CW inscription, which normally occurs very rarely22 (Figure 2B). Each of the five limb lead misplacements changes the direction of inscription of the P loop and/or the frontal P axis. For example, RA/LA cable reversal ‘reflects’ the P loop across the vertical line passing through the LL apex of the Einthoven’s triangle. The resulting ‘mirror image’ of the P loop has obviously reversed direction of inscription (i.e. CW in most cases) and a frontal axis between 115° and 135° instead of the normal range of 45–65°. The authors showed that each of the six possible peripheral cable connections (1 correct + 5 incorrect) can be characterized by a unique combination of direction of P loop inscription (CW or CCW), frontal P axis and the unipolar limb lead in which the ‘real AVR’ appears (Table 2).
Changes in the P wave resulting from interchange of the peripheral cables excluding the ground cable20
Cable reversal . | Typical frontal P axis . | Direction of P loop inscription . |
---|---|---|
None | 45–65° | CCW |
RA/LA | 115–135° | CW |
RA/LL | −105° to −125° | CW |
LA/LL | −5° to −15° | CW |
CW rotationa | −55° to −75° | CCW |
CCW rotationb | 165° to −175° | CCW |
Cable reversal . | Typical frontal P axis . | Direction of P loop inscription . |
---|---|---|
None | 45–65° | CCW |
RA/LA | 115–135° | CW |
RA/LL | −105° to −125° | CW |
LA/LL | −5° to −15° | CW |
CW rotationa | −55° to −75° | CCW |
CCW rotationb | 165° to −175° | CCW |
Refer Table 1 for explanation of abbreviations.
aClockwise rotation here refers to RA → LA → LL → RA cable interchange.
bCounter-clockwise rotation here refers to RA → LL → LA → RA cable interchange.
Changes in the P wave resulting from interchange of the peripheral cables excluding the ground cable20
Cable reversal . | Typical frontal P axis . | Direction of P loop inscription . |
---|---|---|
None | 45–65° | CCW |
RA/LA | 115–135° | CW |
RA/LL | −105° to −125° | CW |
LA/LL | −5° to −15° | CW |
CW rotationa | −55° to −75° | CCW |
CCW rotationb | 165° to −175° | CCW |
Cable reversal . | Typical frontal P axis . | Direction of P loop inscription . |
---|---|---|
None | 45–65° | CCW |
RA/LA | 115–135° | CW |
RA/LL | −105° to −125° | CW |
LA/LL | −5° to −15° | CW |
CW rotationa | −55° to −75° | CCW |
CCW rotationb | 165° to −175° | CCW |
Refer Table 1 for explanation of abbreviations.
aClockwise rotation here refers to RA → LA → LL → RA cable interchange.
bCounter-clockwise rotation here refers to RA → LL → LA → RA cable interchange.
The method requires the presence of P waves and is obviously of limited applicability when P waves are small or abnormal, which renders timing comparisons and hence determination of the P loop direction of inscription difficult or impossible. As mentioned by the authors,24 the method is not applicable to the frontal QRS axis, because the QRS loop can normally be inscribed in both CW and CCW directions.
Interchange involving the neutral electrode cable
The neutral electrode, which is, by convention, placed on the right ankle, may be positioned anywhere on the body without affecting the ECG waveforms. On the other hand, interchange of its cable with the LA or RA cables affects the shape of all precordial leads (because the central terminal is changed) and of all unipolar limb leads, whereas only one of the bipolar limb leads is unaffected.14,25–27 However, the visible change in the precordial leads is small. The following features help to recognize the interchange of a limb lead and the neutral cable (Figure 3).
Lead I, II or III displays (almost) a straight line because it measures the potential difference between both legs, which is negligible.
The other two bipolar limb leads look either identical, or one of them is an inverted (upside down) image of the other.
Two of the unipolar limb leads also look identical, whereas the third looks like one of the bipolar limb leads or its inverted image (Table 3).
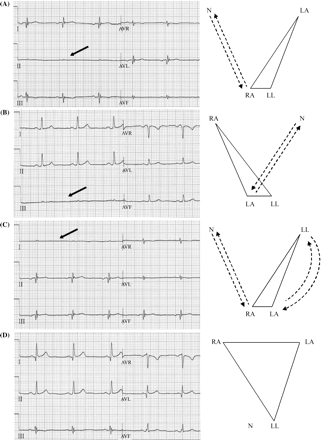
Peripheral leads of a 12-lead ECG recorded in a 34-year-old healthy man with intentional interchange of the right arm–right leg (A), left arm–right leg (B), both arms–corresponding leg electrode cables (C) and with correct cable connections (D). To the right of each ECG are schematically presented the positions of the peripheral electrodes. Note that unlike the cable interchanges presented in Figures 123, interchange of the right leg (ground) electrodes distorts Einthoven’s triangle and Wilson’s central terminal. Also note that the telltale sign of interchange of a peripheral and the ground electrode is the appearance of almost a flat line in one of the standard limb leads (see the arrows). See the text for further details.
ECG changes resulting from interchange of the ground cable and the other peripheral cables
Reversal . | Lead . | ||||||
---|---|---|---|---|---|---|---|
“I” . | “II” . | “III” . | “AVR” . | “AVL” . | “AVF” . | “V1–V6” . | |
RA/RLn | ≅−III | ≅0 | III | ≅AVF | ≅−III | ≅AVR | Distorted |
LA/RLn | ≅II | II | ≅0 | ≅−II | ≅AVF | ≅AVL | Distorted |
LL/RLn | I | ≅II | ≅III | ≅AVR | ≅AVL | ≅AVF | Visibly unchanged |
RA/RLn and LA/LL | ≅0 | −II | −I | ≅AVL | ≅AVR | ≅−III | Distorted |
Reversal . | Lead . | ||||||
---|---|---|---|---|---|---|---|
“I” . | “II” . | “III” . | “AVR” . | “AVL” . | “AVF” . | “V1–V6” . | |
RA/RLn | ≅−III | ≅0 | III | ≅AVF | ≅−III | ≅AVR | Distorted |
LA/RLn | ≅II | II | ≅0 | ≅−II | ≅AVF | ≅AVL | Distorted |
LL/RLn | I | ≅II | ≅III | ≅AVR | ≅AVL | ≅AVF | Visibly unchanged |
RA/RLn and LA/LL | ≅0 | −II | −I | ≅AVL | ≅AVR | ≅−III | Distorted |
RLn—righ leg (neutral) cable. For explanation of the other abbreviations refer to Table 1.
−: The respective lead is inverted upside down; ≅: almost equal to (visibly the same as); 0: the respective lead displays a flat line. As in Table 1, in quotation marks are cited the leads as they are labelled in the ECG recorded with interchanged cables.
ECG changes resulting from interchange of the ground cable and the other peripheral cables
Reversal . | Lead . | ||||||
---|---|---|---|---|---|---|---|
“I” . | “II” . | “III” . | “AVR” . | “AVL” . | “AVF” . | “V1–V6” . | |
RA/RLn | ≅−III | ≅0 | III | ≅AVF | ≅−III | ≅AVR | Distorted |
LA/RLn | ≅II | II | ≅0 | ≅−II | ≅AVF | ≅AVL | Distorted |
LL/RLn | I | ≅II | ≅III | ≅AVR | ≅AVL | ≅AVF | Visibly unchanged |
RA/RLn and LA/LL | ≅0 | −II | −I | ≅AVL | ≅AVR | ≅−III | Distorted |
Reversal . | Lead . | ||||||
---|---|---|---|---|---|---|---|
“I” . | “II” . | “III” . | “AVR” . | “AVL” . | “AVF” . | “V1–V6” . | |
RA/RLn | ≅−III | ≅0 | III | ≅AVF | ≅−III | ≅AVR | Distorted |
LA/RLn | ≅II | II | ≅0 | ≅−II | ≅AVF | ≅AVL | Distorted |
LL/RLn | I | ≅II | ≅III | ≅AVR | ≅AVL | ≅AVF | Visibly unchanged |
RA/RLn and LA/LL | ≅0 | −II | −I | ≅AVL | ≅AVR | ≅−III | Distorted |
RLn—righ leg (neutral) cable. For explanation of the other abbreviations refer to Table 1.
−: The respective lead is inverted upside down; ≅: almost equal to (visibly the same as); 0: the respective lead displays a flat line. As in Table 1, in quotation marks are cited the leads as they are labelled in the ECG recorded with interchanged cables.
With RA/ground cable interchange, lead II displays a flat line, i.e. II ≈ 0 (Figure 3A). Since I + III = II and hence I + III ≈ 0, lead I (in this case potential difference between RL and LA) visibly looks like an inverted image of lead III (−III), whereas lead III is the only 1 unchanged of all 12 leads. Lead AVL, which in this case measures LA – (RL + LL)/2 also looks like –III, whereas AVR [RL – (LA + LL)/2] and AVF [LL – (LA + RL)/2] look identical.
When the LA cable is interchanged with the ground cable, lead III (RL – LL) is a flat line, whereas lead I (RA – RL) is practically the same as lead II (RA – LL, the only unaffected lead) (Figure 3B). Lead AVR, which in this case measures RA – (RL + LL)/2 looks like inverted lead II, whereas AVL [RL – (RA + LL)/2] and AVF [LL – (RA + RL)/2] look identical.
When the 2 arm and the respective 2 leg cables are interchanged, lead I displays a flat line, as it records the voltage difference between the two legs instead of the two arms; lead II (RL – LA) is practically the same as lead III (LL–LA), whereas lead III is inverted because its two electrodes are reversed. Lead AVR [RL – (LA + LL)/2] looks identical to AVL [LL – (RL + LA)/2], whereas AVF [LA – (RL + LL)/2] looks like lead III. Unlike the previous 2 cases of cable interchange, in this case none of the 12 leads is unaffected (Figure 3C).
Interchange of the left leg and the ground (i.e. right leg) cables does not visibly affect the ECG, because the potentials of the legs are almost equal.
When a precordial cable is interchanged with the neutral cable, the respective precordial lead displays essentially the ‘VF’ lead (AVF without augmentation*), because the potential of the right leg (which is approximately equal to that of the left leg) is referenced against the Wilson’s terminal instead of the average of the RA and LA potentials. The peripheral and the rest of the precordial leads are unchanged (Figure 4).
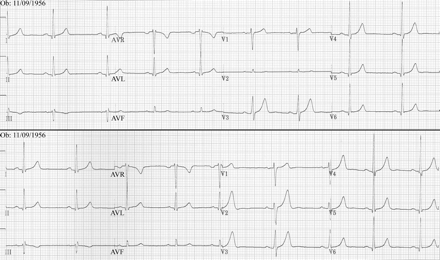
Two resting 12-lead ECGs recorded ∼15 min apart in a 40-year-old man with mild arterial hypertension with correct cable connections (bottom panel) and with intentional interchange of the right leg (ground) cable end the V2 electrode cable (top panel). Note that with this cable interchange, all leads are unchanged except lead V2, look similar to lead AVF but with reduced amplitude. This is practically lead VF (i.e. lead AVF without augmentation). See the text for details.
All the above considerations about misplacement of the ground electrode cable refer to the cases when the leg electrodes are placed on their standard places, i.e. the ankles. In clinical practice, however, the peripheral electrodes are often placed on the torso in accordance with the so-called ‘Mason–Likar system’28 used in exercise and ambulatory electrocardiography. It must be stressed that ECGs recorded with these electrode positions are not ‘essentially identical with the standard 12-lead electrocardiograms’,16,29 as advocated by the authors of this lead system. Moving the peripheral electrodes to the torso changes the potential of central terminal and usually causes rightward shift of the QRS axis and alteration in the R-wave amplitude of the precordial leads.29 Moreover, when the Mason–Likar system is used, the leg electrodes are often placed arbitrarily anywhere in the left and right abdominal region, probably because of the advice from Mason and Likar that the position of the left leg electrode ‘can be varied a few centimetres in any direction’.28Figure 5 clearly demonstrates that while the potential difference between the two ankles is practically zero, this difference is substantially greater between electrodes positioned just above the iliac crests (approximately corresponding to the position of the left leg electrode according to the Mason–Likar system) and further increases between electrodes in the subcostal regions. Thus, when the right arm and right leg cables, for example, are interchanged, lead II will display a flat line only if the leg electrodes have been placed on the lower limbs, but not if they have been positioned on the abdomen (Figure 5). For the same reason, interchange of the 2 leg electrodes positioned on the lower abdomen does visibly change at least the limb ECG leads.
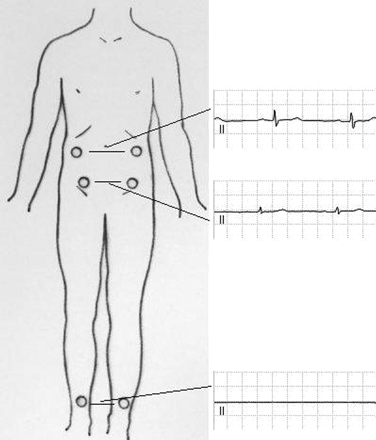
Schematic presentation of the leg electrodes positioned at the level of the ankles, cristae iliacae and the subcostal regions. To the right of the human figure are presented lead II of 3 standard 12 lead ECGs consecutively recorded in one subject with the leg electrodes attached to places marked on the human figure. The rest of the electrodes have been attached to the standard places in all ECGs. In each ECG, the right arm and right leg electrodes have been deliberately interchanged.
It is clear that the voltage difference between the leg electrodes is practically zero only when they are placed on the ankles; in such cases, interchange of the RA and RL cables produces almost a flat line in lead II (Figure 4, bottom electrocardiogram strip). The voltage difference between the leg electrodes positioned at the level of the cristae iliacae is by far not zero (middle electrocardiogram strip) and further increases when the electrodes are moved up to the subcostal region (top electrocardiogram strip).
Ho et al.30 developed an algorithm for detection of all possible interchanges of the peripheral cables including the ground electrode cable based on their effect on the frontal QRS axis (with the peripheral electrodes positioned on the limbs). They demonstrated that all 23 possible incorrect cable connections to the four extremities change the frontal QRS axis in 11 different ways (since reversal of the leg electrodes practically does not affect the axis), which can be expressed by simple formulae (Table 4). They tested their algorithm on 15 individuals (5 with normal axis, 5 with right-axis deviation and 5 with left-axis deviation) and reported a mean difference between actual QRS axes calculated from ECGs recorded with lead misplacement and QRS axes predicted by their formulae of 5 ± 8° (r = 1.0, P < 0.0001, linear regression analysis). The method obviously requires reference QRS axis from previous recordings to be available for comparison. It is not clear to what extent it is also applicable to ECGs recorded with the Mason–Likar electrode positions.
Changes in the frontal QRS axis resulting from interchange of the four peripheral cables26
Cable reversal . | Frontal QRS axis . |
---|---|
RA/LA | 180° − n |
RA/LL | 300° − n |
LA/LL | 60° − n |
CW rotation* | n − 120° |
CCW rotation** | n + 120° |
RA/RLn | −30° or 150° |
LA/RLn | 30° or 210° |
RA/RLn and LA/LL | 90° or 270° |
Cable reversal . | Frontal QRS axis . |
---|---|
RA/LA | 180° − n |
RA/LL | 300° − n |
LA/LL | 60° − n |
CW rotation* | n − 120° |
CCW rotation** | n + 120° |
RA/RLn | −30° or 150° |
LA/RLn | 30° or 210° |
RA/RLn and LA/LL | 90° or 270° |
n—frontal QRS axis of the ECG with correct cable connections. The other abbreviations as in Table 1.
*Clockwise rotation here refers to RA → LA → LL → RA cable interchange.
**Counter-clockwise rotation here refers to RA → LL → LA → RA cable interchange.
Changes in the frontal QRS axis resulting from interchange of the four peripheral cables26
Cable reversal . | Frontal QRS axis . |
---|---|
RA/LA | 180° − n |
RA/LL | 300° − n |
LA/LL | 60° − n |
CW rotation* | n − 120° |
CCW rotation** | n + 120° |
RA/RLn | −30° or 150° |
LA/RLn | 30° or 210° |
RA/RLn and LA/LL | 90° or 270° |
Cable reversal . | Frontal QRS axis . |
---|---|
RA/LA | 180° − n |
RA/LL | 300° − n |
LA/LL | 60° − n |
CW rotation* | n − 120° |
CCW rotation** | n + 120° |
RA/RLn | −30° or 150° |
LA/RLn | 30° or 210° |
RA/RLn and LA/LL | 90° or 270° |
n—frontal QRS axis of the ECG with correct cable connections. The other abbreviations as in Table 1.
*Clockwise rotation here refers to RA → LA → LL → RA cable interchange.
**Counter-clockwise rotation here refers to RA → LL → LA → RA cable interchange.
From a practical point of view, it is important to remember that whilst in most cases, limb lead misplacement shifts the frontal QRS axis significantly (and may simulate a fascicular block), it may sometimes change the frontal QRS axis only negligibly as, for example, RA/LA reversal in a patient with vertical QRS axis (Figure 1) or LA/LL reversal in a patient with QRS axis of 30°.
Interchange of precordial electrodes
Interchange of precordial electrode cables is a much less common technical mistake than incorrect placement of the precordial electrodes31 (too high or too low, V5 and V6 too close to V4, etc.). It is usually easy to detect or suspect by careful inspection of the transition across the chest of the morphology and amplitude of the P waves, QRS–complexes and T waves.20,32 Absence of the normal precordial progression of the R wave (increase in amplitude from V1 to V4 or V5) or S wave (increase from V1 to V2, then progressive decrease from V3 to V6)33 should always alert the reader about the possibility of precordial cable interchange, although there are also other causes, such as myocardial infarction and ventricular hypertrophy. For example, lower R and deeper S in V3 than V1, or P wave with biphasic plus–minus contour in V3 but only positive in V1, or isolated negative T wave only in V3, all point to V1/V3 cable reversal. R in V6 higher than in V5, which may be seen with left ventricular hypertrophy,34 can obviously be due to interchange of the cables to the two leads. However, the amplitude of the R and S wave of the precordial leads (most notably in lead V232) often show artificial variability between successive recordings of the same patient due to variable placement of the electrodes.
Other methods for detection of incorrect cable connections
Hedén et al.35 applied artificial neural networks (ANN) to detect RA/LA cable reversal. The authors took the detection criteria incorporated in two popular computer programs for ECG interpretation (based on QRS and P wave axis) and used them to train the ANNs. Following that, they compared the performance of the ANNs with the two programs. The study was based on 11 009 ECG without lead misplacement and an equal number of computer-derived ECGs with RA/LA cable interchange, divided into a training set and a test set. In the presence of P wave, ANN further increased the high sensitivity of the two programs up to an average of 99.11%. In the absence of P waves, the low sensitivity of the two programs (average of 31 and 39%, respectively) was increased by the ANNs to 63 and 95%, respectively. Both computer programs and ANNs showed very high specificity (close to 100%), both in the presence as well as absence of P waves.
In a second study,3 the authors applied the same method to the same database to test the ability of the ANNs to detect LA/LL and five cable reversal of adjacent precordial cables (V1/V2, V2/V3,…, V5/V6). The sensitivities of the networks ranged between 45 and 83% and were higher than that of the conventional programmes, which were between 0.1 and 10%.
Kors and van Herpen2 reported an ingenious method for automatic detection of lead misplacement in digital 12-lead ECGs. It is based on the fact that the 8 independent leads of the 12-lead ECG (i.e. any 2 peripheral and the 6 precordial leads) contain both independent as well as redundant information and therefore each of them (and hence each of the 12 leads) can be reconstructed from the 7 others in reasonable approximation.36 For a given ECG, each of the 12-leads can be reconstructed from the rest and the correlation coefficient between each original and the corresponding reconstructed lead may be calculated. If the ECG has been recorded with correct cable connections the correlations between each original and the respective reconstructed lead will be high. However, if the ECG has been recorded with some cable interchange, these correlations will generally be lower.† In order to detect cable interchange, each digital ECG is subjected to a series of computationally simulated cable interchanges, and the correlation coefficients between each recorded and the corresponding reconstructed lead are calculated before as well as after each simulated cable interchange.2 If an ECG has originally been recorded with correct cable connections, a computational simulation of a cable interchange will reduce the correlation coefficients. On the other hand, if the same ECG has originally been recorded with a particular cable interchange, the computational simulation of the same interchange will increase the correlation coefficients (because it will remove the cable interchange). The method was tested on a set of more than 3000 ECGs (QRS complex analysis only) and demonstrated specificities of ≥99.5% with sensitivities of ≥93% for detection of 14 common cable interchanges, except LA/LL cable interchange (sensitivity of 17.9%).2
Interchange of precordial and peripheral cables and other cable interchanges
It is impossible to review all 3 628 800 (=1 × 2 ×…× 10) possible interchanges of the 10 ECG cables, some of which are extremely unlikely. Interchange of even one peripheral with a precordial cable will distort the Wilson’s terminal and hence the morphology of the precordial leads may augment the amplitude of some limb leads and will leave not more than 12 (lead I, II, or III) of all 12 leads unchanged. Such bizarre looking ECGs occasionally occur in clinical practice6 (Figure 6) and can be misinterpreted even by experienced electrocardiographers.11
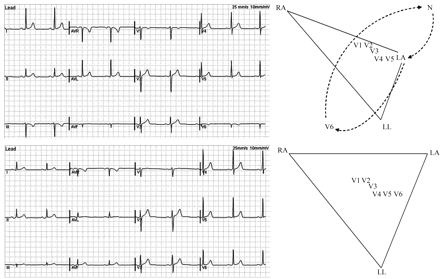
Standard 12-lead electrocardiograms recorded in a healthy young male subject during a drug trial. Top panel: The left arm cable has been connected to the V6 electrode, the V6 cable has been attached to the right leg, and the ground electrode has been connected to the left arm. The participants in the trial have been left to walk freely between recording sessions with the electrodes and cable connected to them and most probably, this subject has decided to re-connect himself inadvertently disconnected cables. Bottom panel: Electrocardiogram in the same subject with correct cable connections.
Conclusions
Some simple yet frequently overlooked rules can help detecting electrode cable reversal in the majority of cases. When a previously recorded tracing is available for comparison, the ECG reader should be aware of any inexplicable change in the axis, polarity or morphology of the P–QRS complex, while bearing in mind that sometime peripheral cable reversal may change only slightly (or ‘normalize’) the axis of the QRS complex or P wave, the latter may be too small for the tell-tale signs of lead misplacement to be seen, and the changes in the precordial leads may be due to variable (i.e. incorrect) placement of the precordial electrodes.
The experienced electrocardiographer should immediately notice unusual (in the normal heart during sinus rhythm) ECG patterns (e.g. negative P–QRS complexes in lead I or II or positive in lead AVR, abnormal precordial R, S, or T wave progression) or physiologically improbable patterns (e.g. an almost flat line in a peripheral lead, opposite polarity of P–QRS in lead I and lead V6, incompatibility between the frontal and horizontal P–QRS–T axis,11 etc.).
Vectorial analysis of the P wave from simultaneously recorded and properly displayed peripheral leads, as described by Ho and Ho,24 can be very helpful for detection of peripheral cable reversal. Computerized or computer-based ECG recorders, which allow the ECG to be displayed in various formats and amplitude gain, or directly display the vectorcardiographic loops, can obviously facilitate such analysis. Generally speaking, the study of temporal relations between corresponding parts of the P wave or QRS complex in different simultaneously recorded leads can render very valuable information not only for detection of lead misplacement.37,38
All electrode cable interchanges, except those involving only peripheral cables (excluding the ground electrode cable) or only precordial cables, affect the reference electrodes for the unipolar limb leads and the precordial leads (Wilson’s central terminal). As a result, in such cases the morphology of all leads (expect, possibly, not more than one of leads I, II, or III) is affected and they should not be used for morphological analysis or precise measurement of intervals (e.g. the QT interval). However, the degree to which different cable interchanges affect the duration of the QT and other intervals has never been systematically investigated.
Computer algorithms capable of reliable recognition of all possible cable interchanges (and, eventually, of reconstruction of the ECG with correct cable connections from the one recorded with lead misplacement) will eventually be introduced. Nevertheless, these should not cause the clinician to forget about the possible clinical errors due to poorly recorded ECGs.
Acknowledgements
We thank Prof. Ivaylo Christov from the Centre of Biomedical Engineering at the Bulgarian Academy of Sciences, for the valuable advice, and Mrs Slaveia Petrova, MSc, from St. George’s University of London, for the important help with the preparation of the figures.
Conflict of interest: none declared.
The term ‘unipolar lead’ is imprecise.16 All 12 leads of the standard ECG are ‘bipolar’ in the sense that they measure the difference between the electrical potentials of two electrodes. In 1934, Wilson et al.17 proposed a new lead designed to measure the potential variations only at a single point. They reasoned that if the potential of one of the electrodes of such a lead did not vary, the lead would record only the potential variations of the other (‘exploring’) electrode (hence the term ‘unipolar lead’). To devise an electrode with a constant potential, they connected with a special circuitry the electrodes of the RA, LA, and LL, reasoning that the sum (or average) of their potentials did not vary throughout the cardiac cycle. Thus was designed the so-called ‘central terminal’ (or Wilson’s terminal), whose potential is relatively constant during the cardiac cycle. Each of the ‘unipolar’ precordial leads measures the difference between the respective ‘exploring’ electrode (V1, V2,…,V6, etc.) and the central terminal (in other words, the central terminal is their ‘reference electrode’). As in the electrocardiographic literature, in this publication, we use the terms ‘central terminal’ and ‘Wilson’s terminal’ interchangeably.
Initially, the ‘unipolar’ limb leads were obtained by pairing the central terminal with the electrode of the right arm (lead VR), left arm (lead VL) and left leg (lead VF), respectively. In 1942, Goldberger18 found a way to augment their voltage. When recording lead VR, he disconnected the RA electrode from the central terminal. Thus was obtained the ‘AVR’ lead which measures the potential difference between the RA and the average of the LA and LL electrodes. In the same way, lead AVL (difference between LA and the average of RA and LL electrodes) and AVF (difference between LL and the average of RA and LA) were modified.
As an example, consider an ECG recorded with RA/LA cable reversal (i.e. with inverted lead I and interchanged leads II and III). Lead I of this ECG will be reconstructed from ‘lead II’ (which is actually lead III) and leads V1–V6 (unchanged) using a universal set of coefficients developed for reconstruction of lead I from lead II and leads V1–V6. The error resulting from using in the reconstruction procedure lead III instead of lead II will be different with different ECGs (e.g. it will be small if lead II and lead III are similar—for example, when the frontal QRS axis is 90°). The resemblance (correlation) between the reconstructed and original (i.e. inverted) lead I will vary with different ECGs, but generally it will be smaller than the correlation between a reconstructed and original (not inverted) lead I of the same ECG recorded without RA/LA cable reversal.