-
PDF
- Split View
-
Views
-
Cite
Cite
Luigi P. Badano, Oscar Gaddi, Carlo Peraldo, Gabriele Lupi, Marta Sitges, Frangisko Parthenakis, Santo Molteni, Maria Rosaria Pagliuca, Biagio Sassone, Paola Di Stefano, Tiziana De Santo, Carlo Menozzi, Michele Brignole, Left ventricular electromechanical delay in patients with heart failure and normal QRS duration and in patients with right and left bundle branch block, EP Europace, Volume 9, Issue 1, January 2007, Pages 41–47, https://doi.org/10.1093/europace/eul144
- Share Icon Share
Abstract
Aims We sought to define the reference values of intra-left ventricular (LV) electromechanical delay (EMD), and to assess the prevalence (and pattern) of intra-LV dyssynchrony in patients with heart failure (HF) and normal QRS and in patients with right and left bundle branch block.
Methods and results We used tissue Doppler imaging echocardiography and a six-LV wall model to study LV EMD in 103 patients [41 with HF and normal QRS, 22 with right bundle branch block (RBBB), and 40 with left bundle branch block (LBBB)], and in 59 controls. In controls, the median intra-LV EMD was 17 ms, (inter-quartile range 13–30); 95% of controls had a value ≤41 ms. Patients showed a longer intra-LV EMD than controls: 33 ms (20–57) in patients with normal QRS, 32 ms (23–50) in RBBB patients, and 50 ms (30–94) in LBBB patients. Intra-LV dyssynchrony (defined as intra-LV EMD >41 ms) was present in 39, 36, and 60% of the patients, respectively. On average, HF patients showed the same pattern of activation as controls, from the septum to the posterior wall, but activation times were significantly prolonged. In RBBB patients the activation sequence was directed from inferior to anterior and in LBBB from anterior to inferior wall.
Conclusions Left ventricular dyssynchrony was present in several patients with HF and normal QRS, and in patients with RBBB; conversely, 40% of LBBB patients showed values of LV EMD within the normal range. Left ventricular activation sequence was different between groups. Assessment of LV synchronicity by means of imaging techniques may be more important than QRS duration or morphology in selecting patients for cardiac resynchronization treatment.
Introduction
Cardiac resynchronization therapy (CRT) has been proved to be effective in patients with drug-refractory heart failure (HF) and QRS duration >120 ms. Several studies have demonstrated both the acute haemodynamic and sustained clinical benefit of CRT in selected patients, as shown by reduced mortality and improvement in HF symptoms, exercise capacity, quality of life, and left ventricular (LV) systolic function.1–8
Despite careful patient selection, however, CRT trials based on QRS complex duration have shown that a considerable subset of patients do not respond to ventricular pacing, and their LV function and clinical status do not improve.9 On the other hand, recent studies have documented the pivotal role of assessing the presence of systolic dyssynchrony10–14 and of identifying the most delayed LV wall15 by echocardiography in predicting improvement of systolic function or LV reverse remodelling after CRT. Most of these studies have focused on patients with left bundle branch block (LBBB). Less is known about patients with normal QRS and those with right bundle branch block (RBBB); moreover, comparative studies are not available.
The aims of this prospective observational study were to define the reference values of a method of measuring intra-LV electromechanical delay (EMD) by means of tissue Doppler imaging (TDI), and to assess the prevalence and pattern of intra-LV EMD in HF patients with normal QRS and in patients with RBBB and LBBB.
Methods
This pilot echocardiographic trial was a multi-centre, international, prospective observational study. Both normal subjects and patients were enrolled at nine European centres from September 2004 to February 2005. This study is part of the ablate and pace in atrial fibrillation (APAF) trial.16 The APAF trial was approved by the local ethics committee of each participating institution.
Patient selection
Eligible patients were randomly selected among those referred for an elective echocardiographic study. Patients with an inadequate echocardiographic study, those who had primary valvular heart disease or previous valve replacement or reconstruction, and those who had suffered a major cardiovascular event in the previous 6 weeks were excluded. Among eligible patients, each participating institution selected HF patients with LV ejection fraction <40% and normal QRS complex (defined as QRS duration ≤100 ms) (HF patients); patients with RBBB and patients with LBBB, irrespective of clinical status and cardiac rhythm. The proportion of patients that each participating institution was requested to provide was 40, 20, and 40% for HF, RBBB, and LBBB, respectively. In addition, each institution was requested to provide data from echocardiographic studies of normal subjects, defined as healthy subjects older than 50 years, with no history of cardiovascular or systemic disease, and normal physical examination, electrocardiogram and echocardiographic findings. For each institution, normal subjects were enlisted in a 1:2 ratio with patients, and constituted the control group for the determination of the reference values of intra-LV and inter-ventricular EMD, and sequence of LV activation.
Echocardiography
Echocardiography was performed by means of commercially available machines (Sonos 5500, Philips, Andover, MA, USA; Vivid 7, GE Healthcare, Horten, Norway; Acuson Sequoia, Siemens, Erlangen, Germany). All images were recorded digitally to allow off-line quantitative analysis and assessment of inter-laboratory reproducibility of measurements. Each measurement was averaged over three consecutive beats during sinus rhythm, and five consecutive beats during atrial fibrillation. M-mode and two-dimensional echocardiograms were obtained in accordance with the American Society of Echocardiography guidelines. Global LV function was assessed both from M-mode tracings by measuring LV end-diastolic and end-systolic diameters and from two-dimensional apical views by measuring LV ejection fraction by means of the modified biplane Simpson's algorithm. Functional mitral regurgitation was estimated from apical views by means of the jet area method. Left ventricular filling time was assessed by pulsed-wave Doppler transmitral flow as the time between the onset of the E-wave and the end of the A-wave. To determine the intra-LV EMD, TDI was performed in the pulsed-wave Doppler mode from three standard apical views (four-chamber, two-chamber, and long-axis). The sample volume was placed in the middle of the basal segments of the six LV walls (namely the inferoseptal, lateral, anteroseptal, posterior, anterior, and inferior walls); gain and filters were adjusted, as needed, in order to eliminate background noise and to obtain clear tissue signals, which were recorded at a sweep speed of 100 mm/s. Time to onset of systolic velocity was measured for all segments from the onset of the QRS complex to the onset of the positive component of the regional systolic velocity (S2), and a six-basal segmental model of LV activation was obtained. Pulsed-wave TDI had a time resolution of 5 ms. Intra-LV EMD was defined as the time difference between the longest and the shortest interval among the six LV walls. The inter-observer correlation for intra-LV EMD was 0.93 (P = 0.0001, Spearman correlation test) in a sample size of 15 patients with HF with and without LBBB.
Finally, inter-ventricular EMD was defined as the difference between the aortic and pulmonary pre-ejection delays, and was determined by pulsed-wave Doppler imaging as the time from the onset of the QRS complex to the beginning of each respective systolic ejection.17
Data analysis
Continuous data were expressed as mean ± SD or median (25th–75th percentile), while categorical variables were expressed as absolute number and percentage. Differences between categorical variables were evaluated by means of the χ2 test or Fisher's exact test, when appropriate. Statistical comparisons of continuous variables between groups and centres were performed by Student's t-test or non-parametric test (Mann–Whitney U test or Wilcoxon) for normal and non-normal distributions, respectively. Statistical comparisons among groups were carried out by one-way analysis of variance (ANOVA). Linear regression analysis was used to identify clinical predictors of dyssynchrony. The statistical significance, defined as P-value < 0.05, was evaluated by means of the Bonferroni correction for normal distributions, or the Kruskal–Wallis non-parametric test. Analyses were performed by means of SPSS 12.0 (SPSS Inc., Chicago, IL, USA) software for Windows.
Results
Overall, 59 controls and 103 patients (41 HF, 22 RBBB, and 40 LBBB) were recruited. Controls were younger than patients (59 ± 8 vs. 68 ± 11, P <0.0001), while the prevalence of male gender was comparable between the two groups (63 vs. 71%, P = NS). Table 1 shows the clinical characteristics and baseline echocardiographic parameters of the different groups. There was no difference in QRS duration between HF patients and controls [85 (80–90) ms vs. 80 (80–88) ms, P = 0.070] (Table 1).
Clinical and echocardiographic characteristics of patients with HF and normal QRS complex, and patients with wide QRS
Parameters . | HF, normal QRS(n = 41) . | RBBB(n = 22) . | LBBB(n = 40) . | P value . |
---|---|---|---|---|
Male gender (%) | 31 (76) | 15 (68) | 26 (65) | 0.645 |
Age (years) | 66 ± 11 | 70 ± 11 | 69 ± 10 | 0.358 |
Sinus rhythm (%) | 24 (58) | 15 (96) | 35 (88) | <0.0001 |
HF (%) | 41 (100) | 8 (36) | 27 (68) | <0.0001 |
Coronary artery disease (%) | 3 (8) | 8 (36) | 8 (20) | 0.029 |
Previous myocardial infarction (%) | 18 (43) | 2 (9) | 6 (15) | 0.005 |
Dilated cardiomyopathy (%) | 13 (32) | 2 (9) | 15 (38) | 0.031 |
Other/unknown heart disease (%) | 7 (17) | 1 (5) | 2 (5) | 0.121 |
NYHA class | 2 (2–3) | 3 (2–3) | 2 (2–3) | 0.443 |
Baseline QRS duration (ms) | 85 (80–90) | 140 (120–161) | 156 (140–179) | <0.0001 |
Standard echocardiography | ||||
LV End-diastolic diameter (cm) | 6.2 (5.6–6.7) | 5.8 (5.0–6.1) | 6.5 (5.5–7.5) | 0.006 |
LV End-Systolic diameter (cm) | 4.5 (4.0–5.5) | 3.5 (2.8–4.5) | 5.2 (3.7–6.2) | 0.001 |
LV Ejection fraction (%) | 35 (27–40) | 50 (35–62) | 32 (24–53) | 0.001 |
TEI index | 0.6 (0.4–0.9) | 1.0 (0.7–2.0) | 1.0(0.7–2.0) | 0.198 |
Mitral regurgitant area (cm2) | 2.5 (0.1–6.0) | 2.2 (0.4–4.5) | 6.6 (1.8–12.5) | 0.047 |
Diastolic filling time (ms) | 396 (274–509) | 465 (386–630) | 370 (300–472) | 0.645 |
EMD | 0.358 | |||
Inter-ventricular delay (ms) | 10 (6–25) | 37 (6–52) | 35 (18–55) | <0.0001 |
Intra-LV delay (ms) | 33 (20–57) | 32 (23–50) | 50 (30–94) | <0.0001 |
Parameters . | HF, normal QRS(n = 41) . | RBBB(n = 22) . | LBBB(n = 40) . | P value . |
---|---|---|---|---|
Male gender (%) | 31 (76) | 15 (68) | 26 (65) | 0.645 |
Age (years) | 66 ± 11 | 70 ± 11 | 69 ± 10 | 0.358 |
Sinus rhythm (%) | 24 (58) | 15 (96) | 35 (88) | <0.0001 |
HF (%) | 41 (100) | 8 (36) | 27 (68) | <0.0001 |
Coronary artery disease (%) | 3 (8) | 8 (36) | 8 (20) | 0.029 |
Previous myocardial infarction (%) | 18 (43) | 2 (9) | 6 (15) | 0.005 |
Dilated cardiomyopathy (%) | 13 (32) | 2 (9) | 15 (38) | 0.031 |
Other/unknown heart disease (%) | 7 (17) | 1 (5) | 2 (5) | 0.121 |
NYHA class | 2 (2–3) | 3 (2–3) | 2 (2–3) | 0.443 |
Baseline QRS duration (ms) | 85 (80–90) | 140 (120–161) | 156 (140–179) | <0.0001 |
Standard echocardiography | ||||
LV End-diastolic diameter (cm) | 6.2 (5.6–6.7) | 5.8 (5.0–6.1) | 6.5 (5.5–7.5) | 0.006 |
LV End-Systolic diameter (cm) | 4.5 (4.0–5.5) | 3.5 (2.8–4.5) | 5.2 (3.7–6.2) | 0.001 |
LV Ejection fraction (%) | 35 (27–40) | 50 (35–62) | 32 (24–53) | 0.001 |
TEI index | 0.6 (0.4–0.9) | 1.0 (0.7–2.0) | 1.0(0.7–2.0) | 0.198 |
Mitral regurgitant area (cm2) | 2.5 (0.1–6.0) | 2.2 (0.4–4.5) | 6.6 (1.8–12.5) | 0.047 |
Diastolic filling time (ms) | 396 (274–509) | 465 (386–630) | 370 (300–472) | 0.645 |
EMD | 0.358 | |||
Inter-ventricular delay (ms) | 10 (6–25) | 37 (6–52) | 35 (18–55) | <0.0001 |
Intra-LV delay (ms) | 33 (20–57) | 32 (23–50) | 50 (30–94) | <0.0001 |
Normally distributed data are reported as mean ± SD, whereas non-normally distributed data are reported as median (25th–75th percentile); P value indicates the comparison among the three groups.
NYHA, New York Heart Association; TEI index is defined as the time between closure and opening of the mitral valve minus aortic ejection time divided by aortic ejection time.
Clinical and echocardiographic characteristics of patients with HF and normal QRS complex, and patients with wide QRS
Parameters . | HF, normal QRS(n = 41) . | RBBB(n = 22) . | LBBB(n = 40) . | P value . |
---|---|---|---|---|
Male gender (%) | 31 (76) | 15 (68) | 26 (65) | 0.645 |
Age (years) | 66 ± 11 | 70 ± 11 | 69 ± 10 | 0.358 |
Sinus rhythm (%) | 24 (58) | 15 (96) | 35 (88) | <0.0001 |
HF (%) | 41 (100) | 8 (36) | 27 (68) | <0.0001 |
Coronary artery disease (%) | 3 (8) | 8 (36) | 8 (20) | 0.029 |
Previous myocardial infarction (%) | 18 (43) | 2 (9) | 6 (15) | 0.005 |
Dilated cardiomyopathy (%) | 13 (32) | 2 (9) | 15 (38) | 0.031 |
Other/unknown heart disease (%) | 7 (17) | 1 (5) | 2 (5) | 0.121 |
NYHA class | 2 (2–3) | 3 (2–3) | 2 (2–3) | 0.443 |
Baseline QRS duration (ms) | 85 (80–90) | 140 (120–161) | 156 (140–179) | <0.0001 |
Standard echocardiography | ||||
LV End-diastolic diameter (cm) | 6.2 (5.6–6.7) | 5.8 (5.0–6.1) | 6.5 (5.5–7.5) | 0.006 |
LV End-Systolic diameter (cm) | 4.5 (4.0–5.5) | 3.5 (2.8–4.5) | 5.2 (3.7–6.2) | 0.001 |
LV Ejection fraction (%) | 35 (27–40) | 50 (35–62) | 32 (24–53) | 0.001 |
TEI index | 0.6 (0.4–0.9) | 1.0 (0.7–2.0) | 1.0(0.7–2.0) | 0.198 |
Mitral regurgitant area (cm2) | 2.5 (0.1–6.0) | 2.2 (0.4–4.5) | 6.6 (1.8–12.5) | 0.047 |
Diastolic filling time (ms) | 396 (274–509) | 465 (386–630) | 370 (300–472) | 0.645 |
EMD | 0.358 | |||
Inter-ventricular delay (ms) | 10 (6–25) | 37 (6–52) | 35 (18–55) | <0.0001 |
Intra-LV delay (ms) | 33 (20–57) | 32 (23–50) | 50 (30–94) | <0.0001 |
Parameters . | HF, normal QRS(n = 41) . | RBBB(n = 22) . | LBBB(n = 40) . | P value . |
---|---|---|---|---|
Male gender (%) | 31 (76) | 15 (68) | 26 (65) | 0.645 |
Age (years) | 66 ± 11 | 70 ± 11 | 69 ± 10 | 0.358 |
Sinus rhythm (%) | 24 (58) | 15 (96) | 35 (88) | <0.0001 |
HF (%) | 41 (100) | 8 (36) | 27 (68) | <0.0001 |
Coronary artery disease (%) | 3 (8) | 8 (36) | 8 (20) | 0.029 |
Previous myocardial infarction (%) | 18 (43) | 2 (9) | 6 (15) | 0.005 |
Dilated cardiomyopathy (%) | 13 (32) | 2 (9) | 15 (38) | 0.031 |
Other/unknown heart disease (%) | 7 (17) | 1 (5) | 2 (5) | 0.121 |
NYHA class | 2 (2–3) | 3 (2–3) | 2 (2–3) | 0.443 |
Baseline QRS duration (ms) | 85 (80–90) | 140 (120–161) | 156 (140–179) | <0.0001 |
Standard echocardiography | ||||
LV End-diastolic diameter (cm) | 6.2 (5.6–6.7) | 5.8 (5.0–6.1) | 6.5 (5.5–7.5) | 0.006 |
LV End-Systolic diameter (cm) | 4.5 (4.0–5.5) | 3.5 (2.8–4.5) | 5.2 (3.7–6.2) | 0.001 |
LV Ejection fraction (%) | 35 (27–40) | 50 (35–62) | 32 (24–53) | 0.001 |
TEI index | 0.6 (0.4–0.9) | 1.0 (0.7–2.0) | 1.0(0.7–2.0) | 0.198 |
Mitral regurgitant area (cm2) | 2.5 (0.1–6.0) | 2.2 (0.4–4.5) | 6.6 (1.8–12.5) | 0.047 |
Diastolic filling time (ms) | 396 (274–509) | 465 (386–630) | 370 (300–472) | 0.645 |
EMD | 0.358 | |||
Inter-ventricular delay (ms) | 10 (6–25) | 37 (6–52) | 35 (18–55) | <0.0001 |
Intra-LV delay (ms) | 33 (20–57) | 32 (23–50) | 50 (30–94) | <0.0001 |
Normally distributed data are reported as mean ± SD, whereas non-normally distributed data are reported as median (25th–75th percentile); P value indicates the comparison among the three groups.
NYHA, New York Heart Association; TEI index is defined as the time between closure and opening of the mitral valve minus aortic ejection time divided by aortic ejection time.
Right bundle branch block patients had smaller LV diameters (P < 0.002), higher LV ejection fraction (P < 0.0001), and longer diastolic filling time (P = 0.007) than patients with HF and normal QRS and LBBB patients (Table 1). Left bundle branch block patients showed significantly more severe mitral regurgitation than both HF patients with normal QRS and RBBB patients (P = 0.021).
Intra-LV EMD
There were no differences in intra-LV EMD distribution between centres (P = 0.65).
Controls
On average, LV activation was completed within 104 ms of QRS onset (Figure 1). The delay in activation among the six walls was rather short. The posterior wall was the latest activated after a median of 9 ms after the inferior septum (P = 0.009) (Table 2 and Figure 1). The median intra-LV EMD was 17 ms, (inter-quartile range 13–30); as 95% of controls showed an intra-LV EMD below 41 ms, intra-LV electromechanical dyssynchrony was defined as a value of intra-LV EMD longer than 41 ms.
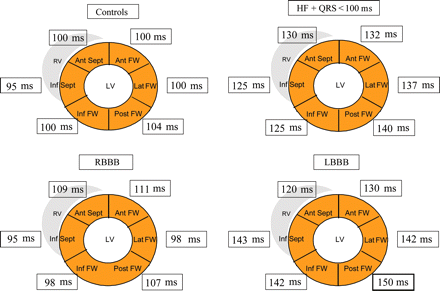
Time to onset of systolic velocity (from the onset of QRS complex to the onset of myocardial systolic velocity on TDI) in the ‘bull-eye’ projection for the six LV walls assessed in controls, HF patients with normal QRS (duration < 100 ms), RBBB and LBBB patients. Ant, anterior; FW, free wall; Inf, inferior; Lat, lateral; Post, posterior; Sept, septum.
Comparison of intra-LV EMD among normal subjects and patients with HF and normal QRS, right bundle branch block, and left bundle branch block
LV wall . | Normal subjects(n = 59) . | HF, normal QRS(n = 41) . | RBBB(n = 22) . | LBBB(n = 40) . |
---|---|---|---|---|
Anterior septum (ms) | 100 (90–101) | 130 (101–159)* | 109 (88–125) | 120 (105–140)* |
Inferior septum (ms) | 95 (90–104) | 125 (103–162)*,** | 95 (79–115)*** | 143 (111–175)*,**** |
Inferior free wall (ms) | 100 (90–104) | 136 (115–165)*,** | 98 (80–124)*** | 142 (114–186)*,**** |
Posterior free wall (ms) | 104 (95–110) | 140 (120–166)*,** | 107 (92–129)*** | 150 (130–186)*,**** |
Lateral free wall (ms) | 100 (94–115) | 137 (109–170)* | 98 (81–158) | 142 (127–176)*,** |
Anterior free wall (ms) | 100 (97–105) | 132 (106–165)* | 111 (86–134) | 130 (107–148)***** |
LV wall . | Normal subjects(n = 59) . | HF, normal QRS(n = 41) . | RBBB(n = 22) . | LBBB(n = 40) . |
---|---|---|---|---|
Anterior septum (ms) | 100 (90–101) | 130 (101–159)* | 109 (88–125) | 120 (105–140)* |
Inferior septum (ms) | 95 (90–104) | 125 (103–162)*,** | 95 (79–115)*** | 143 (111–175)*,**** |
Inferior free wall (ms) | 100 (90–104) | 136 (115–165)*,** | 98 (80–124)*** | 142 (114–186)*,**** |
Posterior free wall (ms) | 104 (95–110) | 140 (120–166)*,** | 107 (92–129)*** | 150 (130–186)*,**** |
Lateral free wall (ms) | 100 (94–115) | 137 (109–170)* | 98 (81–158) | 142 (127–176)*,** |
Anterior free wall (ms) | 100 (97–105) | 132 (106–165)* | 111 (86–134) | 130 (107–148)***** |
Data are reported as median (25th–75th percentile).
*P = 0.0001 vs. normal subjects; **P = 0.010 vs. RBBB patients; ***P = 0.01 vs. HF normal QRS patients; ****P < 0.0001 vs. RBBB patients; *****P < 0.05 vs. normal subjects.
Comparison of intra-LV EMD among normal subjects and patients with HF and normal QRS, right bundle branch block, and left bundle branch block
LV wall . | Normal subjects(n = 59) . | HF, normal QRS(n = 41) . | RBBB(n = 22) . | LBBB(n = 40) . |
---|---|---|---|---|
Anterior septum (ms) | 100 (90–101) | 130 (101–159)* | 109 (88–125) | 120 (105–140)* |
Inferior septum (ms) | 95 (90–104) | 125 (103–162)*,** | 95 (79–115)*** | 143 (111–175)*,**** |
Inferior free wall (ms) | 100 (90–104) | 136 (115–165)*,** | 98 (80–124)*** | 142 (114–186)*,**** |
Posterior free wall (ms) | 104 (95–110) | 140 (120–166)*,** | 107 (92–129)*** | 150 (130–186)*,**** |
Lateral free wall (ms) | 100 (94–115) | 137 (109–170)* | 98 (81–158) | 142 (127–176)*,** |
Anterior free wall (ms) | 100 (97–105) | 132 (106–165)* | 111 (86–134) | 130 (107–148)***** |
LV wall . | Normal subjects(n = 59) . | HF, normal QRS(n = 41) . | RBBB(n = 22) . | LBBB(n = 40) . |
---|---|---|---|---|
Anterior septum (ms) | 100 (90–101) | 130 (101–159)* | 109 (88–125) | 120 (105–140)* |
Inferior septum (ms) | 95 (90–104) | 125 (103–162)*,** | 95 (79–115)*** | 143 (111–175)*,**** |
Inferior free wall (ms) | 100 (90–104) | 136 (115–165)*,** | 98 (80–124)*** | 142 (114–186)*,**** |
Posterior free wall (ms) | 104 (95–110) | 140 (120–166)*,** | 107 (92–129)*** | 150 (130–186)*,**** |
Lateral free wall (ms) | 100 (94–115) | 137 (109–170)* | 98 (81–158) | 142 (127–176)*,** |
Anterior free wall (ms) | 100 (97–105) | 132 (106–165)* | 111 (86–134) | 130 (107–148)***** |
Data are reported as median (25th–75th percentile).
*P = 0.0001 vs. normal subjects; **P = 0.010 vs. RBBB patients; ***P = 0.01 vs. HF normal QRS patients; ****P < 0.0001 vs. RBBB patients; *****P < 0.05 vs. normal subjects.
HF patients with normal QRS
Intra-LV electromechanical dyssynchrony was detectable in 39% of HF patients (P < 0.0001 vs. controls) (Table 3). Although, on average, LV activation pattern was similar to that found in controls, the time to onset of systolic velocity was significantly prolonged in all LV walls (Table 2 and Figure 1).
Distribution of electromechanical ventricular dyssynchrony according to the different electrocardiographic characteristics of the study patients
. | HF, normal QRS (n = 41) . | RBBB (n = 22) . | LBBB (n = 40) . |
---|---|---|---|
Inter-ventricular dyssynchrony alone (%) | 4 (10)*,** | 9 (41) | 20 (50) |
Intra-LV dyssynchrony alone (%) | 16 (39) | 8 (36) | 24 (60) |
Inter-ventricular and intra-LV dyssynchrony (%) | 3 (7)** | 5 (23) | 14 (35) |
. | HF, normal QRS (n = 41) . | RBBB (n = 22) . | LBBB (n = 40) . |
---|---|---|---|
Inter-ventricular dyssynchrony alone (%) | 4 (10)*,** | 9 (41) | 20 (50) |
Intra-LV dyssynchrony alone (%) | 16 (39) | 8 (36) | 24 (60) |
Inter-ventricular and intra-LV dyssynchrony (%) | 3 (7)** | 5 (23) | 14 (35) |
*P < 0.05 vs. RBBB patients; **P < 0.05 vs. LBBB patients.
Distribution of electromechanical ventricular dyssynchrony according to the different electrocardiographic characteristics of the study patients
. | HF, normal QRS (n = 41) . | RBBB (n = 22) . | LBBB (n = 40) . |
---|---|---|---|
Inter-ventricular dyssynchrony alone (%) | 4 (10)*,** | 9 (41) | 20 (50) |
Intra-LV dyssynchrony alone (%) | 16 (39) | 8 (36) | 24 (60) |
Inter-ventricular and intra-LV dyssynchrony (%) | 3 (7)** | 5 (23) | 14 (35) |
. | HF, normal QRS (n = 41) . | RBBB (n = 22) . | LBBB (n = 40) . |
---|---|---|---|
Inter-ventricular dyssynchrony alone (%) | 4 (10)*,** | 9 (41) | 20 (50) |
Intra-LV dyssynchrony alone (%) | 16 (39) | 8 (36) | 24 (60) |
Inter-ventricular and intra-LV dyssynchrony (%) | 3 (7)** | 5 (23) | 14 (35) |
*P < 0.05 vs. RBBB patients; **P < 0.05 vs. LBBB patients.
RBBB patients
Intra-LV electromechanical dyssynchrony was detectable in 36% of RBBB patients (Table 3). These patients showed LV activation pattern different from controls and patients with HF and normal QRS (Table 2 and Figure 1). The first LV wall to be mechanically activated was the inferior septum (59 vs. 44% in HF and 20% in LBBB; P = 0.006). The last LV wall to be mechanically activated were the anterior septum (32 vs. 15% in HF and 10% in LBBB; P = 0.07) and anterior free wall (23 vs. 20% in HF and 3% in LBBB; P = 0.031). Activation of the anterior wall occurred with a median delay of 16 ms after activation of the inferior septum (P = 0.04).
LBBB patients
Intra-LV electromechanical dyssynchrony was detectable in 60% of LBBB patients (Table 3). LBBB patients showed the longest intra-LV EMD (Table 1). The activation pattern in this group was opposite to RBBB group, with the anterior septum being the first activated LV segment and the posterior and lateral free walls the last activated (Figure 1). Activation of the posterior free wall occurred with a median delay of 30 ms after activation of the anterior septum (P = 0.005) (Table 2 and Figure 1).
Additional findings
Predictors of dyssynchrony
When a multivariable linear regression model was applied to five baseline variables (age, QRS duration, LV ejection fraction, LV end-diastolic diameter, and TEI index), QRS duration (P = 0.035) and LV end-diastolic diameter (P = 0.004) were independent predictors of intra-LV dyssynchrony. The correlation between QRS duration and intra-LV EMD was weak (R2 = 0.26, P < 0.0001). In particular, the highest percentage of intra-LV electromechanical dyssynchrony (77%) was observed in the patients with HF and QRS duration >150 ms (P = 0.034 vs. the others).
Inter-ventricular EMD
In controls, right ventricular activation preceded LV activation by 13 ± 11 ms, and 95% of controls had an inter-ventricular EMD below 38 ms; inter-ventricular electromechanical dyssynchrony was, therefore, defined as a value of inter-ventricular EMD longer than 38 ms. In HF patients with normal QRS, inter-ventricular EMD values were similar to those of controls, whereas in patients with wide QRS they were longer (Tables 1 and 3).
Discussion
Our results show that, despite the fact that intra-LV dyssynchrony is related to QRS duration and LV dilatation, the correlation is poor and that LV systolic dyssynchrony can frequently be detected not only in patients with HF and narrow QRS (i.e. QRS duration <120 ms as previously shown18,19) but also in HF patients with normal QRS duration (i.e. QRS duration ≤100 ms). In addition, we showed that LV systolic dyssynchrony is frequent in patients with RBBB, a group of patients usually underrepresented in clinical trials of CRT. Conversely, many LBBB patients (up to 40% of total) showed values of intra-LV EMD within the normal range. Therefore, commonly used LBBB electrocardiographic criteria are not reliable indicators of LV dyssynchrony.
In patients with RBBB and LBBB, the prevalence of LV dyssynchrony would probably have been higher, if only those with HF were selected, as was the case in the patients with narrow QRS. In that the comparison with this latter group may be biased. However, such a comparison was not the aim of the study.
Assessment of intra-LV dyssynchrony
In our study, the time to onset of systolic velocity was measured from the onset of the QRS complex to the onset of the positive component of the regional systolic velocity (S2), using a six-basal segmental model of LV activation. We defined EMD on the basis of differences in regional pre-ejection periods as a surrogate for regional electromechanical coupling interval,19–22 and not, as others have done,12,23 on the basis of the difference in the time from QRS to peak systolic velocity. Our choice was based on two considerations: one theoretical (a pre-ejection parameter is less affected by LV loading conditions and inter-segment interactions than a parameter that depends on a variable amount of LV ejection), and the other practical (the onset of systolic velocity may be easier to identify than its peak, especially in patients with severe LV dysfunction). However, Bordachar et al.21 have demonstrated that both indexes are equally sensitive to the patient's haemodynamic status. In their study, improvement in both of these parameters not only correlated with improved cardiac output but also with a reduction in the severity of mitral regurgitation.21 Finally, we used TDI in the pulsed-wave mode to assess the intra-LV EMD. Single-point measurements of myocardial velocities by means of pulsed-wave TDI can only be performed once in the same heart beat; thus, assessment of EMD may, theoretically, be influenced by heart rate differences, changes in loading conditions and breathing. However, Doppler tracings of the six LV walls are usually recorded in a few minutes, with the patient in suspended respiration. Changes in heart rate can be monitored, and major variations in loading conditions are unlikely to occur in such a brief period in haemodynamically stable patients.
Pulsed-wave TDI has a time resolution of 5 ms, while two-dimensional colour TDI at its best (frame scanning rate = 140 Hz)18,24 has shown a time resolution of 7 ms. Furthermore, two-dimensional colour Doppler has frequently been reported to have a time resolution between 8 and 10 ms (frame scanning rates = 100–120 Hz).23 Lastly, the use of pulsed-wave TDI allowed us to perform our study in a multi-centre setting, without requiring each participating centre to have its own specialized software.
Incidence of intra-LV dyssynchrony and QRS duration and morphology
Cardiac resynchronization therapy is currently indicated in patients with symptomatic HF refractory to medical treatment, poor LV function, and QRS duration longer than 120 ms.25 The rationale for the use of QRS duration in selecting patients for CRT is based on two considerations: firstly, this index is simple, widely available, and easily standardized; second, it is reasonable to assume that the structural modifications that occur in the failing heart are responsible for cardiac dyssynchrony, and that QRS duration is a marker of such structural alterations.26 Studies performed on carefully selected patients have shown that QRS duration broadens as the severity of LV failure increases, and that the QRS complex is an independent marker of increased risk of death in HF patients.27–29 However, in a series of consecutive patients reported by Farwell et al.,30 the 1-year mortality rate was identical (28%) in patients with QRS ≥120 ms or <120 ms. In addition, there is increasing evidence that, in patients with LBBB, the timing and extent of mechanical dyssynchrony are poorly related to QRS duration,31 and that 27–56% of patients with HF and a QRS complex duration <120 ms may present significant LV mechanical dyssynchrony.18,19,32,33
Several studies have attempted to explain why surface electrocardiography is not a good measure of mechanical dyssynchrony. Left ventricular depolarization sequences have been shown, in patients with HF and LBBB, to vary with preserved left bundle activation in some patients,31,34 while a disturbance in electromechanical coupling has been invoked to explain the mechanical dyssynchrony observed in patients with normal QRS duration.35
The prerequisite and the rationale for the benefit of CRT is that there is something to resynchronize, i.e. some cardiac wall that will benefit from an anticipated electrical activation. Left ventricular mechanical dyssynchrony may be present despite a normal QRS complex; conversely, it may be absent when LBBB is revealed by surface ECG. These findings may explain the increasing evidence that CRT may be beneficial in patients with normal QRS duration, but with some degree of mechanical dyssynchrony.36,37 They may also explain the unsatisfactory percentage of patients whose clinical status improves after CRT when QRS complex duration is used as the only surrogate indicator of LV mechanical dyssynchrony.38–41 Accordingly, in patients with wide QRS complexes, mechanical dyssynchrony assessed by M-mode echocardiography,10 TDI,11,14,15,23 or magnetic resonance imaging42 has proved superior to QRS duration in predicting the acute clinical or functional response to CRT.
In our study, the incidence of intra-LV EMD in HF patients with normal QRS was 39%. Conversely, 40% of patients with LBBB did not show any mechanical evidence of intra-LV EMD. These percentages are in line with those reported in previous studies, despite differences in the definition of ‘narrow QRS complex’ and of ‘significant LV dyssynchrony’.18,19,32 The innovation of this study was the assessment of incidence of EMD in patients with RBBB, a cohort of patients not well studied and usually underrepresented in clinical trials of CRT. Among the 1034 patients enrolled in the MIRACLE4 and Contak CD43 trials, only 61 patients with RBBB were identified.44 However, Schuster et al.33 have shown that HF patients, even when they have a narrow QRS or RBBB, display significant LV dyssynchrony when investigated by means of TDI. In addition, Bader et al.22 reported no difference in the incidence of LV mechanical dyssynchrony in HF patients with RBBB and with LBBB. In our study, 36% of patients with RBBB showed a significant intra-LV EMD.
Pooled data from the MIRACLE and Contak CD trials indicated that patients with RBBB who underwent CRT showed no haemodynamic or functional benefit at 3- and 6-month follow-up examinations.44 However, these results may be biased by the fact that surface QRS duration was the selection criterion used in these trials. This hypothesis is supported by data from Garrigue et al.,42 who demonstrated that patients with QRS complex duration >140 ms and RBBB benefited from CRT, but only when RBBB was associated with detection of LV mechanical dyssynchrony by echocardiography.
Thus, neither QRS complex duration nor morphology is a reliable indicator of LV dyssynchrony in HF patients.
Incidence of inter-LV dyssynchrony and QRS duration and morphology
Inter-ventricular EMD values were within the normal range in most HF patients with normal QRS, whereas they were longer in many patients with wide QRS. Thus our data suggest that, in contrast to intra-LV EMD, inter-ventricular EMD is correlated with QRS duration either RBBB or LBBB. Our cut-off value was very similar to that of 41 ms, which was used as an inclusion criterion in the CARE-HF trial.8
LV activation pattern according to QRS duration and morphology
TDI studies performed in patients with non-ischaemic cardiomyopathy and LBBB have shown that the last LV wall to be activated is frequently the lateral wall (35%), followed by the anterior (26%) and posterior (23%) walls.15 However, comparative studies with patients with normal QRS or RBBB are not available. We found that, on average, the HF patients with normal QRS showed the same LV activation pattern as controls being the activation directed from the inferior septum toward the posterior free wall, but intra-LV EMD times were significantly prolonged in comparison with those found in controls. In patients with RBBB from the site of the earliest LV breakthrough (the posterior septum) the activation spread downward and reached the posterior and lateral walls before the anterior septum and anterior wall were activated. The anterior wall was activated significantly later than the posterior septum. Conversely, in LBBB patients the anterior septum and anterior wall were the first and the inferior septum, inferoposterior, and lateral walls were the last segments to be activated. These different activation sequences seem to be due to the different sites of electrical LV breakthrough in these three situations rather than a difference in the mechanism of myocardial contraction.
Identifying the most delayed LV walls and, consequently, the pacing site, seems to be a prerequisite to maximize the effect of CRT.15 Nevertheless, owing to the great inter-patient variability, the study of activation pattern represents an average behaviour and cannot be automatically translated to every individual patient. Therefore, this knowledge has only a conceptual meaning and cannot be used for the selection of the pacing site in an individual patient.
Conclusions
The QRS complex duration and morphology are not reliable indicators of LV dyssynchrony. Our findings suggest that assessment of LV synchronicity by means of imaging techniques may be more important than QRS duration or morphology in selecting patients for cardiac resynchronization. Moreover, owing to the heterogeneity of the activation patterns, individual stimulation site selection and optimization of the modalities of performing resynchronization seem to offer the best way to achieve improved clinical results. The utility of performing individual optimization in all patients with abnormal intra-LV EMD needs stronger clinical evidence, which can only be provided by future controlled prospective trials. This study forms the basis for the development of trials such as the APAF study.16