-
PDF
- Split View
-
Views
-
Cite
Cite
Eva Schuijt, Daniel Scherr, Gernot Plank, Ulrich Schotten, Jordi Heijman, Evolution in electrophysiology 100 years after Einthoven: translational and computational innovations in rhythm control of atrial fibrillation, EP Europace, Volume 27, Issue 1, January 2025, euae304, https://doi.org/10.1093/europace/euae304
- Share Icon Share
Abstract
In 1924, the Dutch physiologist Willem Einthoven received the Nobel Prize in Physiology or Medicine for his discovery of the mechanism of the electrocardiogram (ECG). Anno 2024, the ECG is commonly used as a diagnostic tool in cardiology. In the paper ‘Le Télécardiogramme’, Einthoven described the first recording of the now most common cardiac arrhythmia: atrial fibrillation (AF). The treatment of AF includes rhythm control, aiming to alleviate symptoms and improve quality of life. Recent studies found that early rhythm control might additionally improve clinical outcomes. However, current therapeutic options have suboptimal efficacy and safety, highlighting a need for better rhythm-control strategies. In this review, we address the challenges related to antiarrhythmic drugs (AADs) and catheter ablation for rhythm control of AF, including significant recurrence rates and adverse side effects such as pro-arrhythmia. Furthermore, we discuss potential solutions to these challenges including novel tools, such as atrial-specific AADs and digital-twin-guided AF ablation. In particular, digital twins are a promising method to integrate a wide range of clinical data to address the heterogeneity in AF mechanisms. This may enable a more mechanism-based tailored approach that may overcome the limitations of previous precision medicine approaches based on individual biomarkers. However, several translational challenges need to be addressed before digital twins can be routinely applied in clinical practice, which we discuss at the end of this narrative review. Ultimately, the significant advances in the detection, understanding, and treatment of AF since its first ECG documentation are expected to help reduce the burden of this troublesome condition.
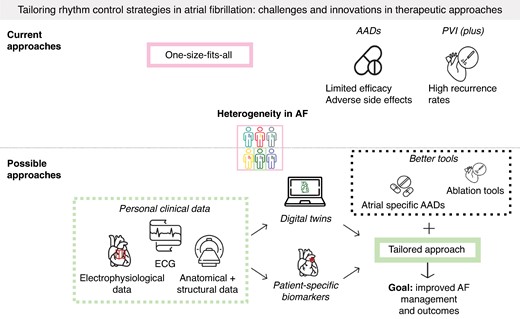
The discovery of the mechanisms of the electrocardiogram by Willem Einthoven, more than 100 years ago, has had a lasting impact on AF that persists today.
Despite significant advances, current rhythm-control therapies have suboptimal efficacy, likely in part due to a one-size-fits-all approach.
Digital twins are growing in popularity and are a promising tool for tailoring rhythm-control therapy that, together with advances in therapeutic modalities (new drugs, better ablation), may help to reduce the burden of AF.
Introduction
Around 1890, the Dutch physiologist Willem Einthoven started working on a capillary electrometer which could record the electrical activity of a human heart. In the recordings made with this instrument, he already labelled the now well-known deflections of an electrocardiogram (ECG): P, Q, R, S, and T. Subsequently, Einthoven developed the string galvanometer as the precursor of the modern ECG machine. In the 1906 paper ‘Le Télécardiogramme’, Einthoven showed recordings of various cardiac arrhythmias, including a first recording of atrial fibrillation (AF).1–3 One hundred years ago, in 1924, Willem Einthoven received the Nobel Prize in Physiology or Medicine for his discovery of the mechanisms of the ECG, enabling clinicians to diagnose and broaden their understanding of various cardiac conditions, including AF.
Atrial fibrillation affects >59 million people worldwide.4 For individuals of European ancestry, the lifetime risk of developing AF is 37% at index age of 55 years.2,5,6 Atrial fibrillation is associated with increased morbidity and mortality, including an increased risk of heart failure, stroke, and other cardiovascular complications.2,7,8 Furthermore, AF causes a significant impairment in quality of life and poses a significant economic9 and logistic burden on modern healthcare systems, being responsible for 0.9–2.6% of the healthcare costs in some European countries.10–13
The understanding of AF pathophysiology, as well as its detection and clinical management have advanced significantly since the first ECG documentation by Einthoven. Treatment and management strategies for AF are covered under the AF-CARE framework, in which ‘R’ refers to reducing symptoms by rate and rhythm control. In the current guidelines, rhythm control, i.e. the attempt to restore and maintain normal sinus rhythm, is recommended as a therapy to reduce symptoms, and in a selected patient group, reduce morbidity and mortality.6 Recent studies have shown that early rhythm control can improve clinical outcomes.14,15
Despite these promising results, existing rhythm-control strategies come with significant challenges. In this narrative review, we discuss these challenges and describe novel methods such as atrial-specific antiarrhythmic drugs (AADs) and tailored-ablation strategies based on patient-specific biomarkers or digital twins that could potentially overcome the existing limitations. Additionally, we highlight the translational challenges that need to be solved to implement these advances in clinical practice.
Current challenges
Heterogeneity of atrial fibrillation
Atrial fibrillation is promoted by numerous comorbidities and risk factors and has several underlying electrophysiological mechanisms.16 Causes and risk factors of AF include genetics, underlying cardiac disease, such as valvular disease, hypertension, and heart failure, as well as advancing age, obesity, obstructive sleep apnoea, and many more.6,17 Several electrophysiological mechanisms, including ectopic foci and different forms of re-entrant activity, including stable or meandering rotors and multiple wavelets, can contribute to the initiation and persistence of AF.17–20 These arrhythmogenic mechanisms are promoted by atrial fibrosis, endocardial–epicardial dissociation, and atrial enlargement (structural remodelling), as well as shortening of the effective refractory period (ERP), impaired electrical cell-to-cell coupling and calcium-handling abnormalities (electrical remodelling).21,22 Once AF has been initiated, additional AF-related atrial remodelling further promotes AF, reflected in the adage ‘AF begets AF’.23 The extent of remodelling can vary significantly between patients24–28 and can vary dynamically over time in an individual patient.29
The diverse risk factors and mechanisms result in a highly heterogeneous clinical manifestation. Some patients have stable short-lived episodic (paroxysmal) AF, while others may develop persistent or long-standing persistent AF. Moreover, patients can have frequent short episodes or infrequent prolonged episodes.30 Patients with AF also experience diverse symptoms such as palpitations, dizziness, fatigue, and shortness of breath, but the correlation between symptoms and rhythm is poor, and some patients are completely asymptomatic.6
In addition, sex-related differences are evident in AF. Women have a lower age-specific AF incidence than men, which is offset by a longer life expectance.31 Women have more risk factors than men, including hypertension and heart failure with preserved ejection fraction.31,32 Furthermore, women have worse AF-related symptoms and poorer quality of life.32,33 Compared with men, women are less frequently treated with electrical cardioversion or catheter ablation.31
The heterogeneity and variability in AF’s etiology, underlying mechanisms and clinical manifestations represent a central overarching challenge for rhythm control of AF, contributing substantially to the suboptimal efficacy of current one-size-fits-most therapeutic strategies, as detailed below.
Optimal time for rhythm control
Several previous studies have shown that early rhythm control in AF is associated with better clinical outcomes,15,34–36 but several questions remain about the impact of the timing for rhythm control on clinical outcomes. For instance, there are partially inconsistent results between studies evaluating the optimal timing for rhythm control. A meta-analysis showed a lower risk of AF recurrence for patients who underwent ablation within 1 year after diagnosis compared to those who had ablation more than 1 year after diagnosis.37,38 Moreover, longer delays between diagnosis and ablation were associated with a higher incidence of adverse outcomes, such as heart failure or ischaemic stroke.39 Conversely, in another study, there was no difference in arrhythmia recurrence between patients on AAD therapy randomized to early ablation (within 1 month of recruitment) or ablation 12 months after recruitment.40 Furthermore, the clinical relevance of small differences in AF recurrences are uncertain.41 These findings highlight the need for more studies to evaluate the optimal timing for rhythm control that should balance the principle of ‘the earlier the better’ with the practical consideration of what is clinically and logistically possible. The outcomes of such studies might have practical implications for the organization of AF care as some geographic regions for instance have considerable waiting lists for AF ablation, whereas others can provide rapid access to treatment.37,39,40,42
Early rhythm control is strongly dependent on early diagnosis of AF, which represents another important challenge. Early diagnosis of AF is challenging, particularly for asymptomatic patients, where diagnosis of AF mostly occurs incidentally during routine medical visits. Moreover, the paroxysmal nature of AF further complicates early diagnosis, even in patients with symptomatic episodes. A potential strategy for early diagnosis of AF is screening approaches, potentially enabled by advances in wearables. However, so far, the benefits of screening for AF and the anticoagulation of patients with device-detected AF appear to be limited in the general population. Large randomized clinical trials (RCTs) studying optimal screening approaches, target populations, and interventions to improve outcomes are limited,6 in part due to the significant logistical challenges and costs associated with such studies.43–45 These data underscore26,30 that further research is needed to determine the optimal approaches for early diagnosis of AF and timing for rhythm-control strategies.
Limitations of antiarrhythmic drugs
Historically, AADs have been the first line of therapy for rhythm control of AF.2,6,46 However, current AADs have limited efficacy in AF patients,46 likely in part because they were not specifically designed for rhythm control of AF.46 For instance, flecainide was originally developed for the suppression of ventricular arrhythmias, and its effectiveness for converting AF to sinus rhythm was empirically observed later.46–48 Similarly, amiodarone was originally introduced for the treatment of angina pectoris.49 Amiodarone is generally considered the most effective AAD for rhythm control of AF, but recurrence rates remain substantial (e.g. 35% over 16 months in CTAF50) and its use is limited by extra-cardiac toxicity. The mean relapse rate for flecainide is 38% based on the three studies with a minimum follow-up of 6 months,51 while patients treated with sotalol or propafenone had recurrence rates of up to 63%.50,52 Dronedarone, a derivative of amiodarone, is the only drug primarily developed and evaluated for long-term rhythm control of AF, with an emphasis on reducing amiodarone’s drug-induced toxicity.53,54 It is also the only AAD that improved clinical outcomes in an RCT.55 Antiarrhythmic drugs have different efficacies in sinus rhythm restoration, with some valuable efficacy in AF episodes whose onset is in the range of hours56 but with lower ability to prevent AF recurrences at long term, with quite variable success rate, ranging from 30 to 60%, indicating that rhythm-control strategies relying solely on these drugs often fail to provide long lasting benefits for a substantial portion of patients.52,57 However, promising data have been reported with AADs on AF progression58 or hard outcomes, at least in observational studies.59
In addition, significant adverse side effects have been reported for many AADs, and there is a risk of inducing life-threatening ventricular pro-arrhythmia, linked to different mechanisms, such as excessive QT prolongation (Class IA, Class III AADs) or facilitation of re-entry in patients with an underlying substrate with AADs slowing conduction velocity.2,52,55,60 Class IC AADs were used in the past for suppressing ventricular tachyarrhythmias and in the CAST trial performed on patients with prior infarction and depressed left ventricular function, use of flecainide was associated with increased mortality.61 Negative results in terms of mortality were also obtained with the selective blocker of the rapid delayed rectifier potassium current d-sotalol in the SWORD trial, again performed in patients with left ventricular systolic dysfunction after myocardial infarction.61–64 These findings highlight the importance of the underlying substrate in conditioning the onset of ventricular pro-arrhythmic effects related to AADs. Amiodarone and dronedarone exhibit properties of all four Vaughan William’s AAD classes and have a relatively low pro-arrhythmic potential. Amiodarone also has potential anti-inflammatory which may contribute to its effectiveness in reducing atrial remodelling. However, the extra-cardiac side effects can also contribute to adverse outcomes, as is evident from amiodarone’s effects on pulmonary fibrosis, thyroid dysfunction, and hepatic toxicity.52,55,65 While the pro-arrhythmic effect of AADs emerged in trials done in the past, mostly focused on ventricular tachyarrhythmias, a more rational and careful use of AADs following well-defined criteria for patient selection and monitoring, as done more recently for rhythm control in AF, e.g. in the EAST AFNET-4 trial, resulted in a low rate of pro-arrhythmia.34,66 These low rates of pro-arrhythmia were also shown for the use of flecainide in AF patients with coronary artery disease67 and for the racemic mixture of d- and l-sotalol in persistent AF patients.68 For an overview of the recurrence rates, adverse side effects and contraindications of the currently available AADs, the reader is referred to Table 1 and other reviews.52,55
AAD . | AF recurrence rates . | Adverse side effects . | Contraindications . |
---|---|---|---|
Amiodarone | 42.2% (95% CI 37.3–47.1%)69 | Non-cardiovascular: pulmonary fibrosis, thyroid dysfunction and hepatic toxicity Cardiovascular: Bradycardia and hypotension Pro-arrhythmia (bradyarrhythmias and tachyarrhythmias): 18 (95% CI 6–57) per 1000 people69 | Congenital long QT syndrome |
Dofetilide | 60.6% (95% CI 51.4–71.6%)69 | Non-cardiovascular: none Cardiovascular: risk of Torsades de pointes Pro-arrhythmia (bradyarrhythmias and tachyarrhythmias): 13 (95% CI 3–53) per 1000 people69 | Long QT syndrome |
Dronedarone | 65.1% (95% CI 61.3–69.7%)69 | Non-cardiovascular: gastrointestinal side effects, hepatotoxicity Cardiovascular: bradycardia Pro-arrhythmia (bradyarrhythmias and tachyarrhythmias): 36 (95% CI 14–91) per 1000 people69 | Congestive heart failure, permanent AF |
Flecainide | 45.4% (95% CI 38.4–53.8%)69 | Non-cardiovascular: dizziness and visual disturbance Cardiovascular: 1:1 atrioventricular conduction Pro-arrhythmia (bradyarrhythmias and tachyarrhythmias): 30 (95% CI 8–112) per 1000 people69 | Brugada syndrome, severe structural or coronary artery disease, severe renal failure |
Ibutilide | Short term evaluation study of efficacy: after 90 min recurrence rate of 29%70 | Non-cardiovascular: nausea Cardiovascular: risk of Torsades de pointes and QT prolongation Pro-arrhythmia (non-sustained monomorphic ventricular tachycardia): 9.76% of patients70 | Long QT syndrome, low left ventricular ejection fraction or left ventricular hypertrophy |
Propafenone | 48.9% (95% CI 44.5–54.0%)69 | Non-cardiovascular: dizziness Cardiovascular: effects on the atrioventricular node Pro-arrhythmia (bradyarrhythmias and tachyarrhythmias): lack of evidence (cochrane analysis)69 | Coronary artery disease, Brugada syndrome |
Sotalol | 65.4% (95% CI 63.1–68.6%)69 | Non-cardiovascular: bronchospasm Cardiovascular: bradycardia, hypotension Pro-arrhythmia (bradyarrhythmias and tachyarrhythmias): 41 (95% CI 25–68) per 1000 people69 | Long QT syndrome |
Vernakalant | Acute cardioversion only; after 90 days: recurrence rate of 51%71 | Non-cardiovascular: sneezing, nausea, dysgeusia Cardiovascular: hypotension, QT prolongation Pro-arrhythmia: absence of pro-arrhythmia in randomized controlled trial71 | Arterial hypotension, long QT syndrome, severe aortic stenosis, acute coronary syndrome |
AAD . | AF recurrence rates . | Adverse side effects . | Contraindications . |
---|---|---|---|
Amiodarone | 42.2% (95% CI 37.3–47.1%)69 | Non-cardiovascular: pulmonary fibrosis, thyroid dysfunction and hepatic toxicity Cardiovascular: Bradycardia and hypotension Pro-arrhythmia (bradyarrhythmias and tachyarrhythmias): 18 (95% CI 6–57) per 1000 people69 | Congenital long QT syndrome |
Dofetilide | 60.6% (95% CI 51.4–71.6%)69 | Non-cardiovascular: none Cardiovascular: risk of Torsades de pointes Pro-arrhythmia (bradyarrhythmias and tachyarrhythmias): 13 (95% CI 3–53) per 1000 people69 | Long QT syndrome |
Dronedarone | 65.1% (95% CI 61.3–69.7%)69 | Non-cardiovascular: gastrointestinal side effects, hepatotoxicity Cardiovascular: bradycardia Pro-arrhythmia (bradyarrhythmias and tachyarrhythmias): 36 (95% CI 14–91) per 1000 people69 | Congestive heart failure, permanent AF |
Flecainide | 45.4% (95% CI 38.4–53.8%)69 | Non-cardiovascular: dizziness and visual disturbance Cardiovascular: 1:1 atrioventricular conduction Pro-arrhythmia (bradyarrhythmias and tachyarrhythmias): 30 (95% CI 8–112) per 1000 people69 | Brugada syndrome, severe structural or coronary artery disease, severe renal failure |
Ibutilide | Short term evaluation study of efficacy: after 90 min recurrence rate of 29%70 | Non-cardiovascular: nausea Cardiovascular: risk of Torsades de pointes and QT prolongation Pro-arrhythmia (non-sustained monomorphic ventricular tachycardia): 9.76% of patients70 | Long QT syndrome, low left ventricular ejection fraction or left ventricular hypertrophy |
Propafenone | 48.9% (95% CI 44.5–54.0%)69 | Non-cardiovascular: dizziness Cardiovascular: effects on the atrioventricular node Pro-arrhythmia (bradyarrhythmias and tachyarrhythmias): lack of evidence (cochrane analysis)69 | Coronary artery disease, Brugada syndrome |
Sotalol | 65.4% (95% CI 63.1–68.6%)69 | Non-cardiovascular: bronchospasm Cardiovascular: bradycardia, hypotension Pro-arrhythmia (bradyarrhythmias and tachyarrhythmias): 41 (95% CI 25–68) per 1000 people69 | Long QT syndrome |
Vernakalant | Acute cardioversion only; after 90 days: recurrence rate of 51%71 | Non-cardiovascular: sneezing, nausea, dysgeusia Cardiovascular: hypotension, QT prolongation Pro-arrhythmia: absence of pro-arrhythmia in randomized controlled trial71 | Arterial hypotension, long QT syndrome, severe aortic stenosis, acute coronary syndrome |
Ablation (PVI) . | AF recurrence rates . | Adverse side effects/complications . | Contraindications . |
---|---|---|---|
Cryoballoon | Overall Meta-analysis (14 months follow-up): 26.9%72 paroxysmal vs. persistent AF patients (15 months follow-up): 21.1 vs. 29.1%73 | Vascular complications, pericardial effusion and tamponade, stroke/transient ischaemic attack, phrenic nerve palsy | Left atrial thrombus |
Radiofrequency | Overall Meta-analysis (14 months follow-up): 33.3%72 paroxysmal vs. persistent AF patients (12 months follow-up): 18 vs. 32%74 | Overall complication rate Systematic review: 4.51% (95% CI 3.76–5.32%)75 | Left atrial thrombus |
Pulsed-field ablation | Overall Systematic review (12 months follow-up): 14.24%76 paroxysmal vs. persistent AF patients (12 months follow-up): 30.5 vs. 37.7%77 | Pericardial tamponade, vascular events Major complications Observational study: ∼1%78 | Left atrial thrombus |
Ablation (PVI) . | AF recurrence rates . | Adverse side effects/complications . | Contraindications . |
---|---|---|---|
Cryoballoon | Overall Meta-analysis (14 months follow-up): 26.9%72 paroxysmal vs. persistent AF patients (15 months follow-up): 21.1 vs. 29.1%73 | Vascular complications, pericardial effusion and tamponade, stroke/transient ischaemic attack, phrenic nerve palsy | Left atrial thrombus |
Radiofrequency | Overall Meta-analysis (14 months follow-up): 33.3%72 paroxysmal vs. persistent AF patients (12 months follow-up): 18 vs. 32%74 | Overall complication rate Systematic review: 4.51% (95% CI 3.76–5.32%)75 | Left atrial thrombus |
Pulsed-field ablation | Overall Systematic review (12 months follow-up): 14.24%76 paroxysmal vs. persistent AF patients (12 months follow-up): 30.5 vs. 37.7%77 | Pericardial tamponade, vascular events Major complications Observational study: ∼1%78 | Left atrial thrombus |
AAD, antiarrhythmic drug; AF, atrial fibrillation; CI, confidence interval; PVI, pulmonary vein isolation.
AAD . | AF recurrence rates . | Adverse side effects . | Contraindications . |
---|---|---|---|
Amiodarone | 42.2% (95% CI 37.3–47.1%)69 | Non-cardiovascular: pulmonary fibrosis, thyroid dysfunction and hepatic toxicity Cardiovascular: Bradycardia and hypotension Pro-arrhythmia (bradyarrhythmias and tachyarrhythmias): 18 (95% CI 6–57) per 1000 people69 | Congenital long QT syndrome |
Dofetilide | 60.6% (95% CI 51.4–71.6%)69 | Non-cardiovascular: none Cardiovascular: risk of Torsades de pointes Pro-arrhythmia (bradyarrhythmias and tachyarrhythmias): 13 (95% CI 3–53) per 1000 people69 | Long QT syndrome |
Dronedarone | 65.1% (95% CI 61.3–69.7%)69 | Non-cardiovascular: gastrointestinal side effects, hepatotoxicity Cardiovascular: bradycardia Pro-arrhythmia (bradyarrhythmias and tachyarrhythmias): 36 (95% CI 14–91) per 1000 people69 | Congestive heart failure, permanent AF |
Flecainide | 45.4% (95% CI 38.4–53.8%)69 | Non-cardiovascular: dizziness and visual disturbance Cardiovascular: 1:1 atrioventricular conduction Pro-arrhythmia (bradyarrhythmias and tachyarrhythmias): 30 (95% CI 8–112) per 1000 people69 | Brugada syndrome, severe structural or coronary artery disease, severe renal failure |
Ibutilide | Short term evaluation study of efficacy: after 90 min recurrence rate of 29%70 | Non-cardiovascular: nausea Cardiovascular: risk of Torsades de pointes and QT prolongation Pro-arrhythmia (non-sustained monomorphic ventricular tachycardia): 9.76% of patients70 | Long QT syndrome, low left ventricular ejection fraction or left ventricular hypertrophy |
Propafenone | 48.9% (95% CI 44.5–54.0%)69 | Non-cardiovascular: dizziness Cardiovascular: effects on the atrioventricular node Pro-arrhythmia (bradyarrhythmias and tachyarrhythmias): lack of evidence (cochrane analysis)69 | Coronary artery disease, Brugada syndrome |
Sotalol | 65.4% (95% CI 63.1–68.6%)69 | Non-cardiovascular: bronchospasm Cardiovascular: bradycardia, hypotension Pro-arrhythmia (bradyarrhythmias and tachyarrhythmias): 41 (95% CI 25–68) per 1000 people69 | Long QT syndrome |
Vernakalant | Acute cardioversion only; after 90 days: recurrence rate of 51%71 | Non-cardiovascular: sneezing, nausea, dysgeusia Cardiovascular: hypotension, QT prolongation Pro-arrhythmia: absence of pro-arrhythmia in randomized controlled trial71 | Arterial hypotension, long QT syndrome, severe aortic stenosis, acute coronary syndrome |
AAD . | AF recurrence rates . | Adverse side effects . | Contraindications . |
---|---|---|---|
Amiodarone | 42.2% (95% CI 37.3–47.1%)69 | Non-cardiovascular: pulmonary fibrosis, thyroid dysfunction and hepatic toxicity Cardiovascular: Bradycardia and hypotension Pro-arrhythmia (bradyarrhythmias and tachyarrhythmias): 18 (95% CI 6–57) per 1000 people69 | Congenital long QT syndrome |
Dofetilide | 60.6% (95% CI 51.4–71.6%)69 | Non-cardiovascular: none Cardiovascular: risk of Torsades de pointes Pro-arrhythmia (bradyarrhythmias and tachyarrhythmias): 13 (95% CI 3–53) per 1000 people69 | Long QT syndrome |
Dronedarone | 65.1% (95% CI 61.3–69.7%)69 | Non-cardiovascular: gastrointestinal side effects, hepatotoxicity Cardiovascular: bradycardia Pro-arrhythmia (bradyarrhythmias and tachyarrhythmias): 36 (95% CI 14–91) per 1000 people69 | Congestive heart failure, permanent AF |
Flecainide | 45.4% (95% CI 38.4–53.8%)69 | Non-cardiovascular: dizziness and visual disturbance Cardiovascular: 1:1 atrioventricular conduction Pro-arrhythmia (bradyarrhythmias and tachyarrhythmias): 30 (95% CI 8–112) per 1000 people69 | Brugada syndrome, severe structural or coronary artery disease, severe renal failure |
Ibutilide | Short term evaluation study of efficacy: after 90 min recurrence rate of 29%70 | Non-cardiovascular: nausea Cardiovascular: risk of Torsades de pointes and QT prolongation Pro-arrhythmia (non-sustained monomorphic ventricular tachycardia): 9.76% of patients70 | Long QT syndrome, low left ventricular ejection fraction or left ventricular hypertrophy |
Propafenone | 48.9% (95% CI 44.5–54.0%)69 | Non-cardiovascular: dizziness Cardiovascular: effects on the atrioventricular node Pro-arrhythmia (bradyarrhythmias and tachyarrhythmias): lack of evidence (cochrane analysis)69 | Coronary artery disease, Brugada syndrome |
Sotalol | 65.4% (95% CI 63.1–68.6%)69 | Non-cardiovascular: bronchospasm Cardiovascular: bradycardia, hypotension Pro-arrhythmia (bradyarrhythmias and tachyarrhythmias): 41 (95% CI 25–68) per 1000 people69 | Long QT syndrome |
Vernakalant | Acute cardioversion only; after 90 days: recurrence rate of 51%71 | Non-cardiovascular: sneezing, nausea, dysgeusia Cardiovascular: hypotension, QT prolongation Pro-arrhythmia: absence of pro-arrhythmia in randomized controlled trial71 | Arterial hypotension, long QT syndrome, severe aortic stenosis, acute coronary syndrome |
Ablation (PVI) . | AF recurrence rates . | Adverse side effects/complications . | Contraindications . |
---|---|---|---|
Cryoballoon | Overall Meta-analysis (14 months follow-up): 26.9%72 paroxysmal vs. persistent AF patients (15 months follow-up): 21.1 vs. 29.1%73 | Vascular complications, pericardial effusion and tamponade, stroke/transient ischaemic attack, phrenic nerve palsy | Left atrial thrombus |
Radiofrequency | Overall Meta-analysis (14 months follow-up): 33.3%72 paroxysmal vs. persistent AF patients (12 months follow-up): 18 vs. 32%74 | Overall complication rate Systematic review: 4.51% (95% CI 3.76–5.32%)75 | Left atrial thrombus |
Pulsed-field ablation | Overall Systematic review (12 months follow-up): 14.24%76 paroxysmal vs. persistent AF patients (12 months follow-up): 30.5 vs. 37.7%77 | Pericardial tamponade, vascular events Major complications Observational study: ∼1%78 | Left atrial thrombus |
Ablation (PVI) . | AF recurrence rates . | Adverse side effects/complications . | Contraindications . |
---|---|---|---|
Cryoballoon | Overall Meta-analysis (14 months follow-up): 26.9%72 paroxysmal vs. persistent AF patients (15 months follow-up): 21.1 vs. 29.1%73 | Vascular complications, pericardial effusion and tamponade, stroke/transient ischaemic attack, phrenic nerve palsy | Left atrial thrombus |
Radiofrequency | Overall Meta-analysis (14 months follow-up): 33.3%72 paroxysmal vs. persistent AF patients (12 months follow-up): 18 vs. 32%74 | Overall complication rate Systematic review: 4.51% (95% CI 3.76–5.32%)75 | Left atrial thrombus |
Pulsed-field ablation | Overall Systematic review (12 months follow-up): 14.24%76 paroxysmal vs. persistent AF patients (12 months follow-up): 30.5 vs. 37.7%77 | Pericardial tamponade, vascular events Major complications Observational study: ∼1%78 | Left atrial thrombus |
AAD, antiarrhythmic drug; AF, atrial fibrillation; CI, confidence interval; PVI, pulmonary vein isolation.
Limitations of catheter ablation
Catheter ablation has emerged as a pivotal therapeutic strategy for rhythm control in AF patients.2,6,79 Catheter ablation is recommended as a first-line treatment option for patients with paroxysmal AF to reduce symptoms and progression.6,80 Pulmonary vein (PV) isolation (PVI) is the cornerstone of AF ablation and can provide significant improvement for patients.6 However, recurrences after PVI remain common, either due to AF triggers outside the PVs or an incomplete isolation of the PVs, especially in patients with persistent AF (Table 1).81–83 For instance, in the RACE-AF trial, 20% of the paroxysmal AF patients experienced AF recurrence 4–6 months after PVI.84 The CAPLA trial included patients with persistent AF undergoing wide antral circumferential ablation to achieve PVI. After 12 months, 53.6% of the patients experienced AF recurrence after a single procedure.85
However, it should be noted that these studies, as well as those discussed next, were based on the recurrence of AF of >30 s on a single ECG during follow-up, which might not be the most clinically relevant outcome. Both studies also demonstrate a significant reduction in AF burden indicating that despite recurrences, patients significantly benefit from AF ablation.
Over the years, numerous ablation strategies have been investigated that aim to improve the efficacy of AF ablation, commonly referred to as ‘PVI plus’ strategies.86 These strategies aim to target other triggers contributing to AF, such as triggers arising from the superior vena cava, coronary sinus, and left atrial appendage.86–89 In addition, linear ablation lines, e.g. a roof line, mitral isthmus line, or box lesion,90,91 as well as ablation of ganglionated plexi has been studied.92–94 The SMASH-AF meta-analysis evaluated the efficacy of different ‘PVI plus’ strategies compared with PVI only, showing a combined incremental success rate of only 7.6% compared with PVI alone. An absolute improvement of 15.1% was observed with ‘PVI plus’ ablation of the superior vena cava, but the residual heterogeneity was large.95 Thus, although ‘PVI plus’ ablation methods can slightly increase the success rate of catheter ablation, results remain suboptimal and there is at present no consensus on the optimal lesion set. Furthermore, these approaches share a common limitation: they are applied in a one-size-fits-all manner. Given the heterogeneity in AF mechanisms, there is a need for tailored-ablation approaches to improve long-term outcomes.
Possible solutions: future directions for rhythm control of atrial fibrillation
The diverse presentations of AF highlight the need for individualized mechanism-based therapeutic strategies. Several potential solutions for these challenges have recently emerged, as discussed below.
Early rhythm control enabled by technological advances
The methods to detect (and ultimately diagnose) AF have changed dramatically over time (Figure 1). Einthoven’s discovery of the precursor of the modern ECG was followed by other methods such as Holter monitoring, handheld single-lead, and smartwatch devices, as well as implantable recorders for continuous rhythm monitoring, greatly facilitating the detection of AF.97,98 For example, the REHEARSE-AF study was the first to show that a handheld ECG increased the diagnosis of AF compared with routine ECG-based AF diagnosis.99 Subsequently, the Apple Heart study and similar studies by Huawei and FitBit showed the feasibility of wearable-based AF detection based on photoplethysmography (PPG) signals.100,101 The ongoing HEARTLINE trial aims to evaluate whether detection of AF with an Apple Watch combining PPG and ECG signals can also improve clinical outcomes.102 Although these emerging technologies have raised important questions, e.g. about the need for anticoagulation in patients with device-detected AF,103 they present promising tools for the early detection of AF, potentially paving the way for early rhythm control or better assessment of therapeutic efficacy of new rhythm-control strategies.
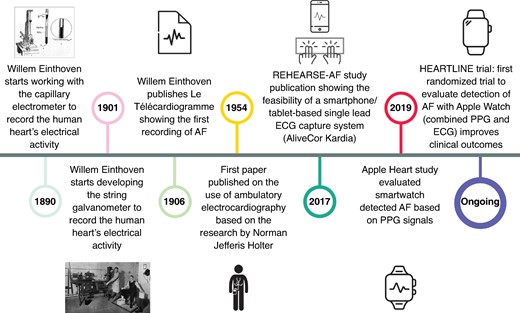
Timeline of key developments in atrial fibrillation detection methods. Photographs reproduced from Salam96 under the Creative Commons Attribution-NonCommercial-ShareAlike 4.0 License.
Advances in pharmacological rhythm control
Antiarrhythmic drugs that are specifically tailored to the unique pathophysiology of AF may show higher efficacy while avoiding ventricular pro-arrhythmia. Several ion channels have an atrial-predominant effect and are up-regulated in AF, making them promising therapeutic targets. For example, small conductance calcium-activated potassium channels (SK or KCa2 channels) show a predominant functional expression in the human atria.104,105 The SK-channel current (ISK) is up-regulated in dogs after AF-induced electrical remodelling and in human patients with long-standing persistent AF, contributing to shortening of the ERP and promoting AF-maintaining re-entry.105 SK-channel inhibition is more effective at restoring sinus rhythm than currently available AADs in large animal models.106 Moreover, in a recent Phase 2 RCT, 48% of the patients with paroxysmal AF receiving the SK-channel inhibitor AP30663 converted to sinus rhythm compared with 6% of the patients receiving placebo. Importantly, no ventricular arrhythmias were observed.107 Another opportunity for advancing pharmacological rhythm control is the repurposing of existing drugs. For example, the respiratory stimulant doxapram is also an inhibitor of atrial-specific TASK-1 potassium channels.108 In a porcine model of persistent AF pigs, long-term treatment with doxapram demonstrated its potential as an AAD for rhythm-control therapy.109 Other novel targets of interest have been suggested, including the acetylcholine-activated inward-rectifier potassium current, abnormal intracellular Ca2+ handling and transient receptor potential channels, as reviewed in detail elsewhere.55,110,111 Although so far no compounds inhibiting these targets have made it to clinical approval, and the regulatory process for novel AADs is highly challenging,46 these promising results suggest that there may be opportunities for expanding the available therapeutic options for pharmacological rhythm control.
Another emerging topic in the context of AADs is the development of novel application strategies. The ‘pill-in-the-pocket’ approach56 enables certain patients with incidental AF to independently perform a pharmacological cardioversion when they experience symptoms, avoiding the risks associated with continuous use of AADs and reducing the frequency of hospital visits for electrical cardioversion.112 Inhalation is a novel application method that allows for rapid drug delivery to the most relevant part of the heart and short-lived exposure to an AAD. The Phase 2 INSTANT trial showed promising results for the efficacy, safety, and feasibility of inhaled flecainide.113 However, effective pill/inhaler-in-the-pocket strategies rely on accurate detection of AF episodes and can therefore be more challenging for asymptomatic AF patients.114 The aforementioned technological advances in wearables (Figure 1) represent a potential solution, allowing (a)symptomatic patients to effectively implement the pill-in-the-pocket approach by using these devices to monitor episodes.115 However, whether independent patient-initiated cardioversion of device-detected AF is a safe and effective rhythm-control strategy needs to be assessed in future RCTs.
Together, the availability of novel, safer atrial-specific AADs and improved options for the detection of AF followed by rapid initiation of rhythm-control therapy, are expected to improve the efficacy and reduce the adverse side effects of pharmacological rhythm-control therapies, potentially improving AF-related outcomes.
Towards tailored-ablation strategies
Recent studies have explored ways to tailor ablation procedures based on patient-specific biomarkers (Table 2) or digital twins (Table 3) with the goal of improving outcomes and minimizing recurrence (Figure 2).
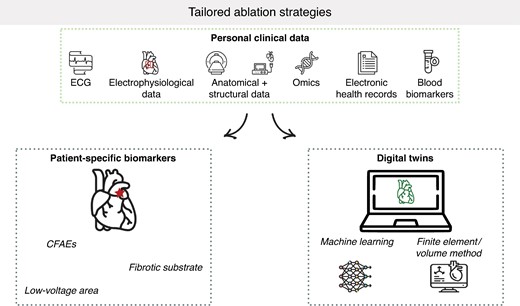
Overview of tailored-ablation strategies. CFAEs, complex fractionated atrial electrograms; ECG, electrocardiogram.
Overview of studies and trials of tailored-ablation approaches using the identification of patient-specific biomarkers
Study (year of publication) . | Patients . | Description . | Primary endpoint . | Outcome . |
---|---|---|---|---|
STAR AF II (2015)82,115 | Persistent AF patients (n = 589) | Multicentre, prospective, single-blind, randomized trial Three ablation strategies:
| During FU (3 months blanking period) freedom from AF recurrence (documented episode >30 s) after a single ablation procedure, with or without AADs | 18 months FU No significant differences in success rates between the three ablation strategies; 49, 46, and 59% of the patients experienced recurrences, respectively (P = 0.15) |
DECAAF II (2022)116,117 | Persistent AF patients (n = 843) | Multicentre, prospective, single-blind, randomized trial Two ablation strategies:
| During FU period (90 day blanking period) first recurrence of AF, atrial flutter or AT (documented episode ≥30 s) after a single ablation procedure or repeat ablation | 12–18 months FU No significant difference in success rates between Group 1 and Group 2, 57.0 and 53.9%, respectively (P = 0.63) |
STABLE-SR-II (2022)118 | Persistent AF patients (n = 300) | Multicentre, prospective, single-blind, randomized trial Two ablation strategies:
| During FU period (3 months blanking period) freedom from AF or AT (documented episode >30 s) after a single ablation procedure without the use of AADs | 18 months FU No significant difference in success rates between both ablation strategies, 67.2 and 67.4%, respectively. (P = 0.52) |
ERASE-AF (2022)119 | Persistent AF patients (n = 324) | Multicentre, prospective, open-label, randomized trial Two ablation strategies:
| During FU period (3 months blanking period) first recurrence of AF or AT (documented episode >30 s) after a single ablation procedure | 12 months FU Significantly higher success rate in the PVI + low voltage area ablation group compared with the PVI only group, 65 vs. 50%, respectively (log rank P = 0.006) |
FLOW-AF (2024)120 | Persistent and long-standing persistent AF patients (n = 85) | Multicentre, prospective, open-label, randomized trial Two (redo) ablation strategies:
| (Secondary effectiveness endpoint) During FU (3 month blanking period) freedom from AF, AT, or atrial flutter | 12 months FU Higher success rate in the PVI in combination with EGF-guided ablation group compared with the PVI only group, 51 vs. 14% (P = 0.103) |
Study (year of publication) . | Patients . | Description . | Primary endpoint . | Outcome . |
---|---|---|---|---|
STAR AF II (2015)82,115 | Persistent AF patients (n = 589) | Multicentre, prospective, single-blind, randomized trial Three ablation strategies:
| During FU (3 months blanking period) freedom from AF recurrence (documented episode >30 s) after a single ablation procedure, with or without AADs | 18 months FU No significant differences in success rates between the three ablation strategies; 49, 46, and 59% of the patients experienced recurrences, respectively (P = 0.15) |
DECAAF II (2022)116,117 | Persistent AF patients (n = 843) | Multicentre, prospective, single-blind, randomized trial Two ablation strategies:
| During FU period (90 day blanking period) first recurrence of AF, atrial flutter or AT (documented episode ≥30 s) after a single ablation procedure or repeat ablation | 12–18 months FU No significant difference in success rates between Group 1 and Group 2, 57.0 and 53.9%, respectively (P = 0.63) |
STABLE-SR-II (2022)118 | Persistent AF patients (n = 300) | Multicentre, prospective, single-blind, randomized trial Two ablation strategies:
| During FU period (3 months blanking period) freedom from AF or AT (documented episode >30 s) after a single ablation procedure without the use of AADs | 18 months FU No significant difference in success rates between both ablation strategies, 67.2 and 67.4%, respectively. (P = 0.52) |
ERASE-AF (2022)119 | Persistent AF patients (n = 324) | Multicentre, prospective, open-label, randomized trial Two ablation strategies:
| During FU period (3 months blanking period) first recurrence of AF or AT (documented episode >30 s) after a single ablation procedure | 12 months FU Significantly higher success rate in the PVI + low voltage area ablation group compared with the PVI only group, 65 vs. 50%, respectively (log rank P = 0.006) |
FLOW-AF (2024)120 | Persistent and long-standing persistent AF patients (n = 85) | Multicentre, prospective, open-label, randomized trial Two (redo) ablation strategies:
| (Secondary effectiveness endpoint) During FU (3 month blanking period) freedom from AF, AT, or atrial flutter | 12 months FU Higher success rate in the PVI in combination with EGF-guided ablation group compared with the PVI only group, 51 vs. 14% (P = 0.103) |
AAD, antiarrhythmic drug; AF, atrial fibrillation; AT, atrial tachycardia; CFAE, complex fractionated atrial electrogram; CPVI, circumferential pulmonary vein isolation; EGF, electrographic flow; FU, follow-up; MRI, magnetic resonance imaging; PEERP, pacing at the end of the effective refractory period; PVI, pulmonary vein isolation.
Overview of studies and trials of tailored-ablation approaches using the identification of patient-specific biomarkers
Study (year of publication) . | Patients . | Description . | Primary endpoint . | Outcome . |
---|---|---|---|---|
STAR AF II (2015)82,115 | Persistent AF patients (n = 589) | Multicentre, prospective, single-blind, randomized trial Three ablation strategies:
| During FU (3 months blanking period) freedom from AF recurrence (documented episode >30 s) after a single ablation procedure, with or without AADs | 18 months FU No significant differences in success rates between the three ablation strategies; 49, 46, and 59% of the patients experienced recurrences, respectively (P = 0.15) |
DECAAF II (2022)116,117 | Persistent AF patients (n = 843) | Multicentre, prospective, single-blind, randomized trial Two ablation strategies:
| During FU period (90 day blanking period) first recurrence of AF, atrial flutter or AT (documented episode ≥30 s) after a single ablation procedure or repeat ablation | 12–18 months FU No significant difference in success rates between Group 1 and Group 2, 57.0 and 53.9%, respectively (P = 0.63) |
STABLE-SR-II (2022)118 | Persistent AF patients (n = 300) | Multicentre, prospective, single-blind, randomized trial Two ablation strategies:
| During FU period (3 months blanking period) freedom from AF or AT (documented episode >30 s) after a single ablation procedure without the use of AADs | 18 months FU No significant difference in success rates between both ablation strategies, 67.2 and 67.4%, respectively. (P = 0.52) |
ERASE-AF (2022)119 | Persistent AF patients (n = 324) | Multicentre, prospective, open-label, randomized trial Two ablation strategies:
| During FU period (3 months blanking period) first recurrence of AF or AT (documented episode >30 s) after a single ablation procedure | 12 months FU Significantly higher success rate in the PVI + low voltage area ablation group compared with the PVI only group, 65 vs. 50%, respectively (log rank P = 0.006) |
FLOW-AF (2024)120 | Persistent and long-standing persistent AF patients (n = 85) | Multicentre, prospective, open-label, randomized trial Two (redo) ablation strategies:
| (Secondary effectiveness endpoint) During FU (3 month blanking period) freedom from AF, AT, or atrial flutter | 12 months FU Higher success rate in the PVI in combination with EGF-guided ablation group compared with the PVI only group, 51 vs. 14% (P = 0.103) |
Study (year of publication) . | Patients . | Description . | Primary endpoint . | Outcome . |
---|---|---|---|---|
STAR AF II (2015)82,115 | Persistent AF patients (n = 589) | Multicentre, prospective, single-blind, randomized trial Three ablation strategies:
| During FU (3 months blanking period) freedom from AF recurrence (documented episode >30 s) after a single ablation procedure, with or without AADs | 18 months FU No significant differences in success rates between the three ablation strategies; 49, 46, and 59% of the patients experienced recurrences, respectively (P = 0.15) |
DECAAF II (2022)116,117 | Persistent AF patients (n = 843) | Multicentre, prospective, single-blind, randomized trial Two ablation strategies:
| During FU period (90 day blanking period) first recurrence of AF, atrial flutter or AT (documented episode ≥30 s) after a single ablation procedure or repeat ablation | 12–18 months FU No significant difference in success rates between Group 1 and Group 2, 57.0 and 53.9%, respectively (P = 0.63) |
STABLE-SR-II (2022)118 | Persistent AF patients (n = 300) | Multicentre, prospective, single-blind, randomized trial Two ablation strategies:
| During FU period (3 months blanking period) freedom from AF or AT (documented episode >30 s) after a single ablation procedure without the use of AADs | 18 months FU No significant difference in success rates between both ablation strategies, 67.2 and 67.4%, respectively. (P = 0.52) |
ERASE-AF (2022)119 | Persistent AF patients (n = 324) | Multicentre, prospective, open-label, randomized trial Two ablation strategies:
| During FU period (3 months blanking period) first recurrence of AF or AT (documented episode >30 s) after a single ablation procedure | 12 months FU Significantly higher success rate in the PVI + low voltage area ablation group compared with the PVI only group, 65 vs. 50%, respectively (log rank P = 0.006) |
FLOW-AF (2024)120 | Persistent and long-standing persistent AF patients (n = 85) | Multicentre, prospective, open-label, randomized trial Two (redo) ablation strategies:
| (Secondary effectiveness endpoint) During FU (3 month blanking period) freedom from AF, AT, or atrial flutter | 12 months FU Higher success rate in the PVI in combination with EGF-guided ablation group compared with the PVI only group, 51 vs. 14% (P = 0.103) |
AAD, antiarrhythmic drug; AF, atrial fibrillation; AT, atrial tachycardia; CFAE, complex fractionated atrial electrogram; CPVI, circumferential pulmonary vein isolation; EGF, electrographic flow; FU, follow-up; MRI, magnetic resonance imaging; PEERP, pacing at the end of the effective refractory period; PVI, pulmonary vein isolation.
Overview of studies and trials of tailored-ablation approaches using digital twins
Study (year of publication) . | Patients . | Description . | Primary endpoint . | Outcome . |
---|---|---|---|---|
Virtual in silico modelling (2017)121 | Persistent AF patients (n = 108) | Multicentre, prospective, randomized trial Two ablation strategies:
| During FU (3 months blanking period) freedom from AF or atrial tachycardia after a single ablation procedure | 12.6 ± 3.8 months FU In silico model approach was feasible but no improvement with the in silico model-based approach compared with empirical ablation: success rate of 86 and 81.1%, respectively (P = 0.538) |
OPTIMA (2019)122 clinical trial ongoing | Persistent AF patients (n = 10) | Prospective clinical feasibility study—proof of concept Ablation strategy: using an iterative pacing protocol, OPTIMA ablation targets were identified using personalized atrial models | Not applicable (proof-of-concept study, clinical trial ongoing) | 309 days FU No persistent AF recurrence in any patient (Note, prospective clinical feasibility study) |
Computational model-guided ablation (2021)123 | Persistent AF patients (n = 170) | Multicentre, prospective, open-label randomized trial Two ablation strategies:
| During FU (3 months blanking period) freedom from AF or atrial tachycardia episodes (>30 s) after a single ablation procedure, without AADs | 16.3 ± 5.3 months FU Significantly higher success rate for the V-DF group compared with the E-PVI group, 74.7 and 48.2%, respectively (P < 0.001) |
PersonAL plan (2023)124 | Persistent AF patients (n = 29) | In silico proof-of-concept feasibility study Thirteen ablation strategies consisting of state-of-the-art anatomical ablation strategies and ablation of HDF regions (PersonAL plan) | Inducibility suppression (evaluated with the PEERP protocol) | After the first round of PersonAL ablation plan, >98% success (no inducible recurrences). After second round of PersonAL ablation plan, 100% success |
i-STRATIFICATION study (2024)125 | Virtual AF patient cohort (n = 800), virtual patients experiencing AF after PVI (n = 522) | 12 rhythm control strategies including various ablation strategies, AAD therapy (amiodarone or vernakalant), or a combination of ablation and AAD therapy | Not applicable | The in silico framework has proven to be feasible to identify an optimal rhythm control strategy based on virtual patient data |
Tailored-AF, interim analysis (2024)126 | Persistent and long-standing persistent AF patients (interim analysis: n = 73, total study population: n = 374) | Multicentre, prospective, randomized trial Two ablation strategies:
| During FU (3 months blanking period), freedom from AF (documented episode >30 s), after a single ablation procedure, with or without AADs | 12 months FU A significant higher success rate for the tailored-approach group compared with the PVI only group, 89 vs. 67%, respectively (P = 0.001) |
Study (year of publication) . | Patients . | Description . | Primary endpoint . | Outcome . |
---|---|---|---|---|
Virtual in silico modelling (2017)121 | Persistent AF patients (n = 108) | Multicentre, prospective, randomized trial Two ablation strategies:
| During FU (3 months blanking period) freedom from AF or atrial tachycardia after a single ablation procedure | 12.6 ± 3.8 months FU In silico model approach was feasible but no improvement with the in silico model-based approach compared with empirical ablation: success rate of 86 and 81.1%, respectively (P = 0.538) |
OPTIMA (2019)122 clinical trial ongoing | Persistent AF patients (n = 10) | Prospective clinical feasibility study—proof of concept Ablation strategy: using an iterative pacing protocol, OPTIMA ablation targets were identified using personalized atrial models | Not applicable (proof-of-concept study, clinical trial ongoing) | 309 days FU No persistent AF recurrence in any patient (Note, prospective clinical feasibility study) |
Computational model-guided ablation (2021)123 | Persistent AF patients (n = 170) | Multicentre, prospective, open-label randomized trial Two ablation strategies:
| During FU (3 months blanking period) freedom from AF or atrial tachycardia episodes (>30 s) after a single ablation procedure, without AADs | 16.3 ± 5.3 months FU Significantly higher success rate for the V-DF group compared with the E-PVI group, 74.7 and 48.2%, respectively (P < 0.001) |
PersonAL plan (2023)124 | Persistent AF patients (n = 29) | In silico proof-of-concept feasibility study Thirteen ablation strategies consisting of state-of-the-art anatomical ablation strategies and ablation of HDF regions (PersonAL plan) | Inducibility suppression (evaluated with the PEERP protocol) | After the first round of PersonAL ablation plan, >98% success (no inducible recurrences). After second round of PersonAL ablation plan, 100% success |
i-STRATIFICATION study (2024)125 | Virtual AF patient cohort (n = 800), virtual patients experiencing AF after PVI (n = 522) | 12 rhythm control strategies including various ablation strategies, AAD therapy (amiodarone or vernakalant), or a combination of ablation and AAD therapy | Not applicable | The in silico framework has proven to be feasible to identify an optimal rhythm control strategy based on virtual patient data |
Tailored-AF, interim analysis (2024)126 | Persistent and long-standing persistent AF patients (interim analysis: n = 73, total study population: n = 374) | Multicentre, prospective, randomized trial Two ablation strategies:
| During FU (3 months blanking period), freedom from AF (documented episode >30 s), after a single ablation procedure, with or without AADs | 12 months FU A significant higher success rate for the tailored-approach group compared with the PVI only group, 89 vs. 67%, respectively (P = 0.001) |
AAD, antiarrhythmic drug; AF, atrial fibrillation; FU, follow-up; HDF, high-dominant frequency; MRI, magnetic resonance imaging; PEERP, pacing at the end of the effective refractory period; PVI, pulmonary vein isolation.
Overview of studies and trials of tailored-ablation approaches using digital twins
Study (year of publication) . | Patients . | Description . | Primary endpoint . | Outcome . |
---|---|---|---|---|
Virtual in silico modelling (2017)121 | Persistent AF patients (n = 108) | Multicentre, prospective, randomized trial Two ablation strategies:
| During FU (3 months blanking period) freedom from AF or atrial tachycardia after a single ablation procedure | 12.6 ± 3.8 months FU In silico model approach was feasible but no improvement with the in silico model-based approach compared with empirical ablation: success rate of 86 and 81.1%, respectively (P = 0.538) |
OPTIMA (2019)122 clinical trial ongoing | Persistent AF patients (n = 10) | Prospective clinical feasibility study—proof of concept Ablation strategy: using an iterative pacing protocol, OPTIMA ablation targets were identified using personalized atrial models | Not applicable (proof-of-concept study, clinical trial ongoing) | 309 days FU No persistent AF recurrence in any patient (Note, prospective clinical feasibility study) |
Computational model-guided ablation (2021)123 | Persistent AF patients (n = 170) | Multicentre, prospective, open-label randomized trial Two ablation strategies:
| During FU (3 months blanking period) freedom from AF or atrial tachycardia episodes (>30 s) after a single ablation procedure, without AADs | 16.3 ± 5.3 months FU Significantly higher success rate for the V-DF group compared with the E-PVI group, 74.7 and 48.2%, respectively (P < 0.001) |
PersonAL plan (2023)124 | Persistent AF patients (n = 29) | In silico proof-of-concept feasibility study Thirteen ablation strategies consisting of state-of-the-art anatomical ablation strategies and ablation of HDF regions (PersonAL plan) | Inducibility suppression (evaluated with the PEERP protocol) | After the first round of PersonAL ablation plan, >98% success (no inducible recurrences). After second round of PersonAL ablation plan, 100% success |
i-STRATIFICATION study (2024)125 | Virtual AF patient cohort (n = 800), virtual patients experiencing AF after PVI (n = 522) | 12 rhythm control strategies including various ablation strategies, AAD therapy (amiodarone or vernakalant), or a combination of ablation and AAD therapy | Not applicable | The in silico framework has proven to be feasible to identify an optimal rhythm control strategy based on virtual patient data |
Tailored-AF, interim analysis (2024)126 | Persistent and long-standing persistent AF patients (interim analysis: n = 73, total study population: n = 374) | Multicentre, prospective, randomized trial Two ablation strategies:
| During FU (3 months blanking period), freedom from AF (documented episode >30 s), after a single ablation procedure, with or without AADs | 12 months FU A significant higher success rate for the tailored-approach group compared with the PVI only group, 89 vs. 67%, respectively (P = 0.001) |
Study (year of publication) . | Patients . | Description . | Primary endpoint . | Outcome . |
---|---|---|---|---|
Virtual in silico modelling (2017)121 | Persistent AF patients (n = 108) | Multicentre, prospective, randomized trial Two ablation strategies:
| During FU (3 months blanking period) freedom from AF or atrial tachycardia after a single ablation procedure | 12.6 ± 3.8 months FU In silico model approach was feasible but no improvement with the in silico model-based approach compared with empirical ablation: success rate of 86 and 81.1%, respectively (P = 0.538) |
OPTIMA (2019)122 clinical trial ongoing | Persistent AF patients (n = 10) | Prospective clinical feasibility study—proof of concept Ablation strategy: using an iterative pacing protocol, OPTIMA ablation targets were identified using personalized atrial models | Not applicable (proof-of-concept study, clinical trial ongoing) | 309 days FU No persistent AF recurrence in any patient (Note, prospective clinical feasibility study) |
Computational model-guided ablation (2021)123 | Persistent AF patients (n = 170) | Multicentre, prospective, open-label randomized trial Two ablation strategies:
| During FU (3 months blanking period) freedom from AF or atrial tachycardia episodes (>30 s) after a single ablation procedure, without AADs | 16.3 ± 5.3 months FU Significantly higher success rate for the V-DF group compared with the E-PVI group, 74.7 and 48.2%, respectively (P < 0.001) |
PersonAL plan (2023)124 | Persistent AF patients (n = 29) | In silico proof-of-concept feasibility study Thirteen ablation strategies consisting of state-of-the-art anatomical ablation strategies and ablation of HDF regions (PersonAL plan) | Inducibility suppression (evaluated with the PEERP protocol) | After the first round of PersonAL ablation plan, >98% success (no inducible recurrences). After second round of PersonAL ablation plan, 100% success |
i-STRATIFICATION study (2024)125 | Virtual AF patient cohort (n = 800), virtual patients experiencing AF after PVI (n = 522) | 12 rhythm control strategies including various ablation strategies, AAD therapy (amiodarone or vernakalant), or a combination of ablation and AAD therapy | Not applicable | The in silico framework has proven to be feasible to identify an optimal rhythm control strategy based on virtual patient data |
Tailored-AF, interim analysis (2024)126 | Persistent and long-standing persistent AF patients (interim analysis: n = 73, total study population: n = 374) | Multicentre, prospective, randomized trial Two ablation strategies:
| During FU (3 months blanking period), freedom from AF (documented episode >30 s), after a single ablation procedure, with or without AADs | 12 months FU A significant higher success rate for the tailored-approach group compared with the PVI only group, 89 vs. 67%, respectively (P = 0.001) |
AAD, antiarrhythmic drug; AF, atrial fibrillation; FU, follow-up; HDF, high-dominant frequency; MRI, magnetic resonance imaging; PEERP, pacing at the end of the effective refractory period; PVI, pulmonary vein isolation.
Patient-specific biomarkers
A first step towards a tailored-AF-ablation strategy was the focal ablation of complex fractionated atrial electrograms (CFAEs) as markers for areas with slow heterogeneous conduction that are more likely to sustain AF.82,127,128 These areas can be mapped for each patient using an electroanatomic mapping system, creating a set of personalized ablation targets.127,128 Although initial results were promising,127 subsequent studies did not show a benefit with CFAE ablation compared with PVI only.82,115,128
The DECAAF study estimated atrial tissue fibrosis using late gadolinium enhancement magnetic resonance imaging (LGE-MRI) of AF patients undergoing catheter ablation. This study showed an independent association between atrial tissue fibrosis and the likelihood of recurrent arrhythmia after ablation.24 Consequently, the DECAAF II trial randomly assigned patients with persistent AF to PVI combined with MRI-guided atrial fibrosis ablation or PVI only. Despite the promising results of the DECAAF study, there was no significant difference in atrial arrhythmia recurrence observed in the DECAAF II trial. Moreover, in the PVI plus MRI-guided ablation group, a significantly higher rate of safety outcomes was observed (2.2 vs. 0.0% with PVI only; P = 0.001), including six patients (1.5%) experiencing an ischaemic stroke.116,117 However, AF burden was reduced, irrespective of the recurrences observed after the procedure.129
Atrial low-voltage areas have been identified as substrates for re-entry and therefore as possible AF ablation targets130,131 that can be detected using voltage mapping during sinus rhythm.132,133 However, in the STABLE-SR-II trial (2022), there was no difference in AF recurrence between persistent AF patients undergoing PVI or PVI plus low-voltage area guided ablation after 18 months of follow-up. The minimal presence of low-voltage areas resulted in only 41% of the patients in the PVI plus low-voltage ablation group receiving additional ablation, potentially explaining the similarity in AF recurrence.118 In contrast, in the ERASE-AF trial (2022), persistent AF patients randomized to PVI plus low-voltage area guided ablation had lower recurrence rates (35%) compared with patients receiving PVI only (50%).119
In the FLOW-AF trial, persistent and long-standing persistent AF patients undergoing PVI in combination with electrographic flow guided source redo ablation showed an absolute improvement of AF-free survival of 51% compared with patients undergoing PVI only redo ablation.120
An overview of the studies and trials of tailored-ablation approaches using patient-specific biomarkers is provided in Table 2. With some notable exceptions, most studies unfortunately failed to show a clear improvement in freedom from AF recurrence with tailored-ablation strategies compared with PVI only. In all studies, AF recurrence rates remained substantial, even with personalized ablation strategies. However, again it should be noted that these studies were based on recurrence of AF of >30 s on a single ECG during follow-up, which might not be the most clinically relevant outcome.134,135 Future studies should assess whether tailored-ablation strategies based on these or other biomarkers can reduce AF burden and/or clinical outcomes.
Digital twins
One of the most exciting prospects for tailoring rhythm-control therapy is the development of digital twins. A digital twin is a detailed virtual model of, in this case, a patient’s heart, created using clinical data, imaging data and/or electrophysiological recordings in combination with biophysical laws that control the spatiotemporal changes in cardiac electrophysiology in computational simulations. In particular, for each digital twin a 3D geometric mesh is created from patient-specific MRI or computed tomography (CT) data (anatomical twinning). Subsequently, each node in the mesh is assigned specific electrophysiological properties, which may depend on location, local substrate (e.g. fibrosis vs. myocardium) and occasionally may be personalized based on mapping data or non-invasive ECG(-imaging), a process termed functional twinning. Finally, conductivity between nodes is assigned based on assumptions about the local fibre directions (typically derived from anatomically determined rules).136,137 The resulting models can simulate atrial electrical activation under various conditions, providing mechanistic insights, helping predict the risk of arrhythmias and enabling clinicians to simulate various rhythm-control strategies to predict the most effective treatment option.138,139 In particular, AF can be induced by pacing the model from different locations. The digital twin allows for modification of electrophysiological properties to simulate the effects of ablation. The digital twin is then re-assessed to determine whether AF remains inducible. If AF persists, an iterative process is applied to refine and evaluate ablation targets until AF is no longer inducible, thereby identifying the optimal set of ablation targets (Figure 3). Some initial studies that showcase the potential of digital twins for personalizing rhythm-control therapy are highlighted below and are summarized in Table 3.
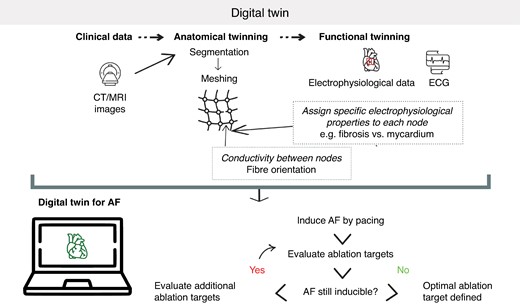
Schematic representation of the use of a digital twin for determining atrial fibrillation ablation strategies.
Several computational studies have shown the theoretical potential of digital twins in guiding rhythm-control therapy. For example, Azzolin et al.,124 created digital twin models of 29 persistent AF patients to compare various ablation strategies currently used in clinical practice with the so-called Personalized Ablation Lines (PersonAL) approach. These digital twins contain data on a patient’s anatomy, fibrosis, either from CT or LGE-MRI data, and electrophysiology obtained from electrophysiological mapping performed during sinus rhythm before PVI. In the PersonAL approach, high-dominant-frequency regions are identified as ablation targets. Virtual ablation of these targets terminated AF in ∼50% of cases and converted AF to atrial tachycardia in 48% of simulations. Another computational study that combines both ablation and AADs is the i-STRATIFICATION study, in which in silico models of a virtual patient population were developed, accounting for variability in anatomy, electrophysiology, and tissue structure. The final evaluation included 522 models with persistent AF after PVI. These models underwent various secondary treatments consisting of different ablation strategies, pharmacological strategies using amiodarone or vernakalant, or a combination of ablation and AAD, highlighting the potential of in silico modelling to predict the optimal combined treatment approach.125
While these and other studies suggest the potential of digital-twin-guided rhythm-control therapy, prospective RCTs are necessary to establish the effectiveness of this approach. The first multicentre prospective RCT evaluating the use of in silico models to optimize ablation therapy was performed in 2017 and compared an empirical ablation strategy (consisting of circumferential PVI; PVI plus a posterior box lesion; PVI plus a posterior box lesion with an anterior line lesion; PVI plus roof and left-lateral isthmus line lesions; or PVI plus CFAE-guided ablation) with a strategy selected by an in silico model. The study demonstrated the feasibility of the in silico model to guide the selection of the ablation strategy. Nevertheless, the outcomes of the in silico model-guided ablations were not superior to the outcomes of the empirical ablation group, despite more extensive lesions in the simulation-guided ablation arm.121 A potential explanation is the fact that the models only consisted of CT-derived patient-specific geometric information and did not include any patient-specific information on structural and electrophysiological characteristics. Furthermore, the strategies were pre-defined and did not take into account the location of AF drivers in the models.
In the OPTIMA study (2019), atrial models were developed using patient-specific data on atrial geometry and fibrosis obtained from LGE-MRI as well as average (generic) human persistent AF electrophysiological properties. Pre-procedure, all potential AF sources within the fibrotic substrate, referred to as the OPTIMA ablation targets, were iteratively identified. By evaluating the model response to pacing, these optimal ablation targets were defined so that no inducible sites could be detected. These pre-defined targets were then integrated into an electro-anatomical mapping system to guide the ablation process. The initial study showed the feasibility of this approach.122 An RCT (ClinicalTrials.gov identifier NCT04101539) comparing PVI plus OPTIMA ablation to PVI only in symptomatic persistent AF patients was initiated based on the promising results of the proof-of-concept study.
In 2021, a multicentre RCT divided patients with persistent AF into two groups: PVI plus a computational model-guided ablation (V-DF group) or PVI only (E-PVI group). In the V-DF group, a virtual dominant-frequency map was generated during the ablation procedure based on a substrate map. Using this map, additional ablation lines were planned and executed. The trial demonstrated a significantly larger freedom from recurrences in the V-DF group (74.7%) compared with the E-PVI group (48.2%).123
In addition to mechanistic digital twins, personalized rhythm-control strategies may also be determined by artificial intelligence (AI)-based approaches. For example, an AI/machine-learning-based analysis of atrial electrograms to identify areas of spatiotemporal dispersion has been proposed to identify ablation targets.140 An interim analysis of the Tailored-AF trial (2024), performed in persistent and long-standing persistent AF patients, shows promising results of this concept. Tailored-AF compared PVI only with PVI combined with ablating AI-designated areas of spatiotemporal dispersion. At follow-up, the recurrences of AF in the tailored group were significantly lower (11%) compared with the PVI only group (33%).126 While AI-based approaches are perhaps less intuitive than mechanistic models that can actually show the atrial electrical activity, they have the unique ability to also consider signals and parameters that cannot readily be integrated in mechanistic models. In addition, advances in explainable AI may facilitate a better understanding of AI-based approaches, likely supporting their acceptance in clinical practice. Future work may combine mechanistic- and AI-based digital twin approaches. For example, AI-based approaches can predict ablation outcomes from ECG data,141–144 potentially helping to identify in which patients classical ablation strategies might suffice and in which patients it is worthwhile to invest the effort to construct mechanistic digital twins.
Better tools
Effective and safe ablation tools are required to implement these tailored-ablation strategies. Pulsed-field ablation has emerged as a promising novel energy source with fast effects and myocardial selectivity, resulting in less collateral damage and lower procedure times compared with radiofrequency ablation.145–147 A study including persistent AF patients undergoing pulsed-field ablation shows a success rate of 75% within the 90-day blanking period.145 The ADVENT trial shows that pulsed-field ablation, compared with thermal ablation, resulted in more patients having a post-ablation atrial arrhythmia burden below the clinically significant threshold of 0.1% burden.148 Further advances in pulsed-field ablation catheters are expected to facilitate their use beyond PVI, although the safety and effectiveness of each system will have to be carefully evaluated given the differences between systems.135
Translational challenges
While the methods proposed to improve rhythm-control therapies are promising, several translational challenges exist.
Challenges related to the clinical translation of novel AADs include the limitations of existing (small) animal models that can be used for testing therapeutic strategies. The important differences between the cardiovascular systems of small animals and humans limit the use of these models. Large animal models, such as dogs and horses, have shown to be useful to study AF mechanisms and novel therapies, but these are expensive, time-consuming, and raise potential ethical concerns.149 Ultimately, large-scale clinical trials are needed to establish the safety and efficacy of potential atrial-specific AADs. However, recent discoveries have made the design of these trials considerably more complex than traditional randomized controlled trials for AADs. For example, given the heterogeneity of AF mechanisms, it is unlikely that any novel AAD will be effective in every AF patient. As such, also in view of the costs associated with these trials, a careful balance will have to be found between a sufficiently large target (sub)population and maximizing the likelihood of efficacy. Similarly, application strategies (continuous vs. pill-in-the-pocket approaches), timing (e.g. clinical vs. device-detected AF; establishing a definition of early rhythm-control therapy), and follow-up (use of wearables, endpoints) may have to be carefully reconsidered.150,151
Improving rhythm-control therapies using digital twins is highly promising, as demonstrated by the various studies described in Table 3. The ultimate goal is to enhance rhythm control through a tailored mechanism-based approach, supported by large RCTs showing improved outcomes with digital-twin-guided care. However, several translational challenges must be addressed to achieve this. An important aspect of digital twins is the personalization of the models using various types of patient-specific clinical data. The distribution and level of fibrosis in the atria are highly relevant for AF ablation, as multiple studies have shown the association between fibrotic tissue and re-entrant drivers’ locations that play a role in the maintenance of AF.24,152–161 Late gadolinium enhancement-MRI is commonly performed as a non-invasive method to detect atrial fibrosis.162 While this imaging modality is effective, it is not 100% specific for fibrosis,163 and LGE-MRI of the atria requires (amongst others) a high spatial resolution and specialized scan protocols.151 Therefore, improvements in detection of atrial fibrosis, validated using mapping and/or histology are required. In addition, some patients have a primarily functional substrate meaning that re-entrant drivers that add to the maintenance of AF are not solely correlated with fibrotic tissue or other structural inhomogeneities. This indicates the importance of incorporating patient-specific electrophysiological parameters in addition to patient-specific atrial geometry and fibrosis patterns. However, the availability of non-invasive pre-procedural information on electrophysiological parameters is limited, and therefore, electrophysiological properties are not personalized in most studies to date, making it debatable whether these can be considered true digital twins. Moreover, even if these data would be available, the dynamic nature of these electrophysiological parameters poses another challenge. More research is needed to develop methods to measure electrophysiological parameters non-invasively and pre-procedurally and integrate this information into digital twins, an aspect sometimes referred to as ‘functional twinning’, while also incorporating their dynamical characteristics.151 On the other hand, it remains to be seen how much personalization will be necessary or feasible for guiding AF ablation.163
In addition to personalization, there are several other hurdles related to the practical implementation of digital-twin-guided care. Digital twins include a high-dimensional parameter space and integrate data across multiple scales which adds to the complexity and thereby computational requirements. Furthermore, including digital twins in clinical practice requires time-consuming methods such as segmentation of fibrotic structures from LGE-MRI and processing other patient-specific data for integration in the digital twin models. This all needs to be incorporated into the complex existing clinical workflow and systems. Additionally, the implementation of digital twin-guided rhythm-control therapies requires the cooperation of multiple experts including clinicians, industry, and modellers. Overall, the feasibility and economic incentives need to be demonstrated in appropriately designed clinical studies to enable digital twin-guided care.151,137 It is likely that digital twin-guided care is neither feasible nor necessary for all AF patients. Careful patient selection will therefore be required. Finally, the introduction of new rhythm-control therapies into clinical practice, as well as the implementation of earlier rhythm control compared with usual care requires new regulations and may face potential regulatory hurdles. For example, the integration of AI into clinical practice is not straightforward as several concerns arise including patient data privacy, ethical biases, and security. In Europe, medical decision-making using AI falls under the Medical Device Regulation to ensure safety. Furthermore, AI tools must comply with the General Data Protection Regulation requirements for patient data privacy and to ensure proper data handling. Finally, the implementation of these new methods necessitates updated reimbursement policies.
Conclusions
The discovery of the mechanisms of the ECG by Willem Einthoven, more than 100 years ago, has had a lasting impact that persists today. Currently, the ECG remains the main tool used to diagnose AF and increasing evidence suggest that a sinus rhythm ECG is associated with better outcomes than an AF ECG, raising the importance of effective rhythm-control therapy. At the same time, technological innovations have opened up numerous opportunities for the early detection and monitoring of AF. Despite significant advances, current rhythm-control therapies have limited efficacy and adverse side effects. The heterogeneity in the comorbidities and risk factors, electrophysiological mechanisms, and clinical manifestation of AF calls for a tailored mechanism-based approach in combination with better tools and early AF detection possibilities to optimize outcomes. Digital twins are growing in popularity and are a promising tool for tailoring rhythm-control therapy. However, the implementation of digital-twin-guided care comes with numerous translational challenges. Ultimately, large RCTs that show better outcomes with digital-twin-guided care are required to support the use of digital twins in clinical practice.
Funding
This work was supported by the Netherlands Heart Foundation (grant no. 01-002-2022-0118, EmbRACE: Electro-Molecular Basis and the Rapeutic management of Atrial Cardiomyopathy, fibrillation and associated outcomEs to U.S. and J.H.), the European Union (MAESTRIA: Machine Learning Artificial Intelligence Early Detection Stroke Atrial Fibrillation, grant no. 965286 to U.S. and BEAT-AF: Ground-BrEAking Electroporation-based inTervention for Atrial Fibrillation treatment, grant no. 945125 to D.S.), and the Netherlands Organization for Scientific Research (NWO/ZonMW Vidi 09150171910029 to J.H.).
Data availability
No new data were generated in support of this review article.
References
Author notes
Conflict of interest: U.S. received consultancy fees or honoraria from Università della Svizzera Italiana (USI, Switzerland), Roche Diagnostics (Switzerland), EP Solutions Inc. (Switzerland), Johnson & Johnson Medical Limited (UK), Bayer Healthcare (Germany). U.S. is co-founder and shareholder of YourRhythmics BV, a spin-off company of the University Maastricht. G.P. is a co-founder of NumeriCor GmbH. All the remaining authors have declared no conflicts of interest.