-
PDF
- Split View
-
Views
-
Cite
Cite
Shinya Yamada, Li-Wei Lo, Yu-Hui Chou, Wei-Lun Lin, Shih-Lin Chang, Yenn-Jiang Lin, Shin-Huei Liu, Wen-Han Cheng, Tsung-Ying Tsai, Shih-Ann Chen, Renal denervation ameliorates the risk of ventricular fibrillation in overweight and heart failure, EP Europace, Volume 22, Issue 4, April 2020, Pages 657–666, https://doi.org/10.1093/europace/euz335
- Share Icon Share
Abstract
Both obesity and heart failure (HF) are associated with sudden cardiac death. The current study aimed to investigate the effects of overweight and HF on the substrate for ventricular fibrillation (VF), and whether renal denervation (RDN) can protect the heart from sympathetic activation and cardiac remodelling in HF rabbits fed with high-fat diet (HFD).
Twenty-four rabbits randomized into control group fed with regular diet (Control), HFD, HFD-HF, and HFD-HF-RDN groups. Rapid ventricular pacing of 400 b.p.m. for 4 weeks was applied in HFD-HF and HFD-HF-RDN. Surgical and chemical RDNs were approached through bilateral retroperitoneal flank incisions in HFD-HF-RDN. All rabbits received electrophysiological study and a VF inducibility test. The ventricular myocardium was harvested for trichrome stain. After 3 months, mean body weight was heavier in HFD, compared with control (3.5 ± 0.1 kg vs. 2.6 ± 0.1 kg, P < 0.01). No differences in body weight among the three groups fed with HFD were observed. The ventricular refractory periods were longer in HFD-HF and HFD-HF-RDN than in control. An extension of ventricular fibrosis was observed in HFD and HFD-HF compared with control, and the degree of ventricular fibrosis was suppressed in HFD-HF-RDN compared with HFD-HF. The level of tyrosine hydroxylase staining was reduced in HFD-HF-RDN compared with HFD and HFD-HF. Importantly, VF inducibility was lower in HFD-RDN-HF (10 ± 4%), when compared with those in HFD-HF (58 ± 10%, P < 0.01) and HFD (42 ± 5%, P < 0.05), respectively.
Our results suggest that overweight and HF increase sympathetic activity, structural remodelling, and VF inducibility, but RDN prevents them.
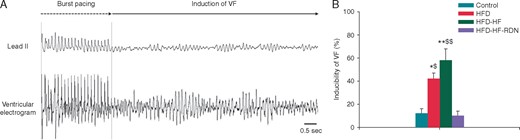
We have demonstrated that overweight is associated with increase of ventricular arrhythmogenesis, possibly through cardiac sympathetic activation.
In the overweight and heart failure (HF) models, prolongation of ventricular effective refractory period (ERP), severe ventricular fibrosis, cardiac sympathetic activation, and high ventricular fibrillation (VF) inducibility were noted. In the overweight and HF models with renal denervation (RDN), ventricular ERP was not shortened, but the degree of ventricular fibrosis and VF inducibility were significantly suppressed compared with the overweight and HF models without RDN. Additionally, cardiac sympathetic activity was also significantly attenuated by RDN. Therefore, RDN can prevent VF inducibility through reverse structural and autonomic remodelling.
Introduction
Heart failure (HF) is the final common pathway of various cardiac pathologies. Activation of the sympathetic nervous system plays an important role in the progression of HF, and it is associated with the development of proarrhythmic structural and electrical remodelling processes in the failing heart.1 Overweight and obesity are recognized as risk factors of cardiac remodelling and overt HF2 and have been linked to a high risk of sudden death.3 Although the epidemiology and aetiologies of sudden death vary, ventricular arrhythmias such as ventricular tachycardia and ventricular fibrillation (VF) are the most common cause.4 A previous study reported obese patients had a high risk of appropriate implantable cardioverter-defibrillator therapy in compared to non-obese patients.5 Therefore, obesity may be associated with a higher incidence of life-threatening ventricular arrhythmia. Overweight and obesity have been closely linked to propensity for atrial fibrillation. The relationship between overweight and atrial arrhythmogenic substrates was reported in a study by Abed et al.6 In ventricular arrhythmogenesis, corrected QT interval and its dispersion in electrocardiography and left ventricular mass in echocardiography were increased in the rabbits fed with a high-fat diet (HFD) compared with controls.7 Additionally, hydrogen peroxide, tumour necrosis factor-α, and C-reactive protein were increased in the rabbits with HFD compared with controls.8 These factors are generally considered to be associated with myocardial fibrosis. However, whether overweight and obesity result in ventricular remodelling and in turn cause high VF inducibility has not yet been fully elucidated.
Renal artery sympathetic denervation (RDN) has been demonstrated to reduce sympathetic activity. Therefore, RDN may have a great potential in the treatment of cardiovascular diseases such as HF,9 atrial fibrillation,10 and ventricular tachyarrhythmias.11 However, whether RDN can protect the heart from myocardial deterioration in obese patients with HF remains unclear. Thus, the current study aimed to investigate the effect of overweight on structural and electrical remodelling, resulting in high VF inducibility, and whether RDN can reduce the arrhythmic events in rabbits with pacing-induced HF and overweight.
Methods
Animal preparation
The present study conformed to the Guide for the Care and Use of Laboratory Animals in addition to being approved by the Institutional Animal Use and Care Committee of Taipei Veterans General Hospital (IACUC No 2016-028). A total of 24 male New Zealand white rabbits (weight 2–3.5 kg in the beginning, from Shulin Breeding facility, New Taipei, Taiwan), aged 12 weeks, were used. One animal per cage was housed in a temperature-regulated room with a 12:12 h light-dark cycle. Six control rabbits were fed with unlimited high fibre (22.6% fibre and 6.6% fat) food (Laboratory Rabbit Diet 5326 HF, PMI, Richmond, IN, USA) and water. To induce progressive weight gain, 18 rabbits were fed with a HFD which comprised of the normal food with an additional 15% fat (10% corn oil and 5% lard) for 3 months, as described in a previous study.7
Rabbit heart failure model
Heart failure was induced in the rabbits by rapid ventricular pacing through programmable right ventricular pacemakers (Sigma SS303; Medtronic, Minneapolis, MN, USA), implanted as described previously.10,11 In brief, an intramuscular injection of a mixture of Zoletil 50 (10 mg/kg) and xylocaine (5 mg/kg) was given at the beginning of the procedure. Repeated injections of Zoletil 50 and xylocaine were administered as required to maintain a deep level of anaesthesia. An intravenous catheter was inserted into the marginal ear vein for the anaesthetics. The rabbits were then intubated and ventilated artificially with room air or supplemented with oxygen to maintain oxygen saturation >95%. After opening the right third intercostal space, part of the pericardium was cut to expose the anterior surface of the heart. A unipolar pacing lead (Medtronic; pacing lead 6491) fixed to the right ventricular free wall was connected to the pacemaker, which was implanted subcutaneously at the right subaxillary area. For 3 days postoperatively, the rabbits received antibiotics infusion (gentamicin, 5–8 mg/kg) and ibuprofen (2–10 mg/kg) in their water for pain relief. Each pacemaker was programmed to a rate of 400 b.p.m. after the animal recovered from the surgery (1 week after implantation). Electrocardiograms were monitored once per week to adjust the pacing to the maximum ventricular rate, thus allowing for 1:1 capture. At the end of a 4-week pacing period, HF status was confirmed by the presentation of signs of ascites, oedema, drowsiness, and dyspnoea, which were compatible with experimental and clinical HF.10,11
Rabbit renal artery sympathetic denervation model
By using the same anaesthesia method, both kidneys were approached through a mid-abdominal incision. The chemical RDN has been described before.10–12 In brief, each rabbit underwent fasting for one night before the surgery. Bilateral kidneys were surgically denervated by cutting all visible nerves in the area of the renal hilus (surgical RDN) and by stripping approximately 1 cm of the adventitia from the renal artery. The area was then moistened with a 20% phenol solution for 10–15 min (chemical RDN). Following RDN, the animals were allowed to re-equilibrate for 1 h, the abdomen was closed layer by layer with sutures, and bleeding was checked.
Experimental procedures
As shown in Figure 1A, the rabbits were randomized into four groups:
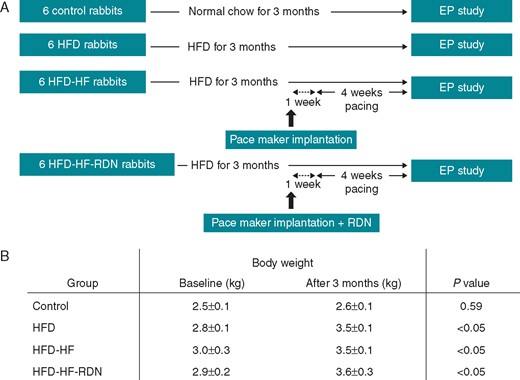
Study protocol and weight gain during the follow-up. (A) Study outline. (B) The changes of body weight during a 3-month follow-up. EP, electrophysiological; HF, heart failure; HFD, high-fat diet; RDN, renal denervation.
Control group (n = 6): there was no pacemaker implantation or RDN in this group.
HFD group (n = 6): there was no pacemaker implantation or RDN in this group. A HFD was administered and followed by observation for 3 months before electrophysiology study.
HFD-HF group (n = 6): the rabbits, fed with a HFD for 3 months, received right thoracotomy with implantation of a pacemaker. After 1 week of recovery, rapid right ventricular pacing was started and followed by observation for 4 weeks before electrophysiology study.
HFD-HF-RDN group (n = 6): the rabbits, fed with a HFD for 3 months, received both right thoracotomy with pacemaker implantation and bilateral RDNs. After 1 week of recovery, rapid right ventricular pacing was started and followed by observation for 4 weeks before electrophysiology study.
General preparation
On the day of electrophysiology study, a warming blanket was used for the rabbits to maintain their body temperature. An intravenous line was set-up for the medication and fluid supplement. All rabbits were anaesthetized with an intraperitoneal injection of sodium pentobarbital (40 mg/kg). They were artificially ventilated through a cuffed endotracheal tube by a constant volume cycled respirator with room air or oxygen as needed. They were then put in the supine position. A mid-thoracotomy was performed and the muscle was dissected with carefully bleeding check-up in the procedure until the mediastinal space was exposed. The pericardium was then incised to expose the whole heart completely.
Conventional electrophysiology study and induction of ventricular fibrillation
In all rabbits, programmed electrical stimulation was performed by a custom-made stimulator (Model 5325, Medtronic, Ltd, Minneapolis, MN, USA) that delivered constant-current pulses of 1-ms duration. Initially, effective refractory periods (ERPs) of bi-atria and bi-ventricles were measured. Programmed electrical stimulation was delivered to the multielectrode catheter distal tips at 2 and 10 times the pacing threshold that sutured epicardially at the bi-atria and bi-ventricles. Eight consecutive stimuli (S1-S1 = 300 ms cycles) followed by a premature stimulus (S1-S2). The S1-S2 were initially decreased from 200 ms by decrements of 10 ms and then 1 ms when achieving ERP. The shortest S1-S2 interval that resulted in a propagated atrial and ventricular responses were taken as the atrial and ventricular ERPs, respectively. Subsequently, burst S1S1 ventricular pacing (cycle length decreasing from 250 ms to failure of 1:1 ventricular capture) was delivered to induce VF. VF inducibility was defined as an incidence of sustained (>30 s) VF induced by a shortest ventricular pacing at 1:1 cycle length (usually between 100 and 150 ms, burst S1S1 pacing for 10 s), with a maximum pacing output (10 mA) during 10 inductions, as described previously.11 The percentage of VF induction with induced VF was compared among the four groups. If the VF was sustained for more than 30 s, an external electrical defibrillation was performed to restore to sinus rhythm.
Tissue harvest
The rabbits were sacrificed by exsanguination under anaesthesia at the end of the electrophysiology study, and the atrial and ventricular myocardia were obtained. Atrial and ventricular tissues were sampled at the bi-atrial and bi-ventricular free walls, and flushed free of blood. A fixation procedure was performed immediately in both 20% formalin and liquid nitrogen to prevent sample degradation. Renal sampling was also performed with kidneys frozen in liquid nitrogen and stored at −80°C to allow adrenaline and noradrenaline concentrations to be determined.
Histological study and immunohistochemistry
Bi-atrial and bi-ventricular tissues were dehydrated by sequential washes with 70% ethanol, 80% ethanol, 90% ethanol, and 100% ethanol, and then imbedded in paraffin. Sections were cut parallel to the plane of the atrioventricular annulus to include the epicardial and endocardial aspects of the myocardium in each slice and were dyed with Masson’s trichrome, which resulted in fibrotic (collagen-enriched) areas appearing blue. This was in contrast with cellular elements, which appeared red. Masson’s trichrome-stained myocardial sections were imaged, and the collagen areas of the ventricles and atria were calculated as percentages of the total ventricular and atrial myocardial areas, respectively, by using an image analyzer (Image-Pro Plus 6.0, Media Cybernetics). These results served as an estimate of the progression of the fibrosis. For the immunohistochemistry staining, antibodies of myocardium for tyrosine hydroxylase (TH) were used to stain sympathetic nerves. In addition, TH staining of left stellate ganglion was performed to determine if there are damaged neurons in animals with RDN. Sympathetic neuron marker densities were measured as total area of nerves (μm2) per square millimetre by using an image analyzer (Image-Pro Plus 6.0).
Statistical analysis
Continuous data were reported as mean value ± standard error. Categorical data were presented as absolute values and percentages. Data variables among the groups (intergroup) were compared with the Kruskal–Wallis test. A P-value of <0.05 was considered statistically significant, and resulted in follow-up comparisons of the different groups using the Dunn test. Additionally, a Wilcoxon signed-rank test was used to evaluate whether there were differences in body weight at baseline and after 3 months. Analysis was performed by using SPSS statistical software (Version 22.0, SPSS Institute Inc., Chicago, IL, USA).
Results
Baseline characteristics
Twenty-four rabbits randomized into control group fed with regular diet (Control), HFD, HFD-HF, and HFD-HF-RDN groups as shown in Figure 1A. The changes of body weight during a 3-month follow-up are shown in Figure 1B. There was no significant difference in body weight at baseline among the four groups (P = 0.378). The mean weight of the rabbits that were administered a HFD for 3 months was higher than that of the controls (3.5 ± 0.1 kg vs. 2.6 ± 0.1 kg, P < 0.01). There were no body weight differences among the HFD (3.5 ± 0.1 kg), HFD-HF (3.5 ± 0.1 kg), and HFD-HF-RDN (3.6 ± 0.3 kg) rabbits. Progressive weight gain was observed among the three groups fed with HFD. However, there was no weight change in the control rabbits.
Effective refractory period and induction of ventricular fibrillation
The atrial and ventricular ERPs of the four groups are shown in Figure 2. The bi-atrial and bi-ventricular ERPs of the HFD-HF rabbits at 10 times the pacing thresholds were significantly longer compared with those of the controls. In left atrium, the ERP of the HFD-HF rabbits at two times the pacing thresholds was also significantly longer compared with the controls. The left ventricular and right atrial ERPs of the HFD-HF rabbits at 10 times the pacing thresholds were significantly longer than those of the HFD rabbits, and the right ventricular and left atrial ERPs of the HFD-HF-RDN rabbits at 10 times the pacing thresholds were significantly longer than those of the controls. In the left atrium, the ERP of the HFD-HF-RDN rabbits at two times the pacing thresholds was also significantly longer than that of the control rabbits. However, there were no significant differences in bi-atrial and bi-ventricular ERPs between the control and HFD rabbits.
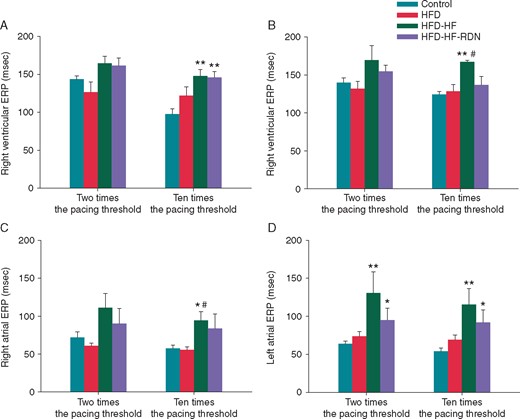
Comparisons of ERPs among the four groups. (A) Right ventricular ERPs at 2 and 10 times the pacing threshold. (B) Left ventricular ERPs at 2 and 10 times the pacing threshold. (C) Right atrial ERPs at 2 and 10 times the pacing threshold. (D) Left atrial ERPs at 2 and 10 times the pacing threshold. ERP, effective refractory periods; HF, heart failure; HFD, high-fat diet; RDN, renal denervation. *P<0.05 vs. Control, **P<0.01 vs. Control and #P < 0.05 vs. HFD.
Sustained VF could be induced by burst ventricular pacing (Figure 3A). As shown in Figure 3B, the VF inducibility was higher in the HFD-HF rabbits, when compared with the control and HFD-HF-RDN rabbits, respectively. The VF inducibility was also higher in the HFD rabbits, when compared with the control and HFD-HF-RDN rabbits, respectively.
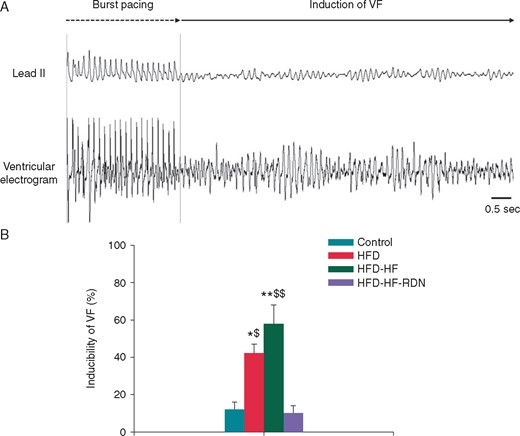
Incidence of VF by burst ventricular pacing. (A) A representative example of VF induction. In this model, 1:1 cycle length of a shortest ventricular pacing was 140 ms. VF was induced during a ventricular burst pacing at 1:1 cycle length of 140 ms in a HFD-HF rabbit. (B) Inducibility of VF among the four groups. HF, heart failure; HFD, high-fat diet; RDN, renal denervation; VF, ventricular fibrillation. *P<0.05 vs. Control, **P<0.01 vs. Control, $P < 0.05 vs. HFD-HF-RDN and $$P < 0.01 vs. HFD-HF-RDN.
Kidney tissue catecholamine after renal denervation
Information on the renal catecholamine levels is shown in Figure 4. There were no significant differences in the adrenaline content of the renal tissue specimens among the four groups. However, the noradrenaline content of the renal tissue specimens was significantly lower in the HFD-HF-RDN rabbits as compared to the HFD and HFD-HF rabbits.
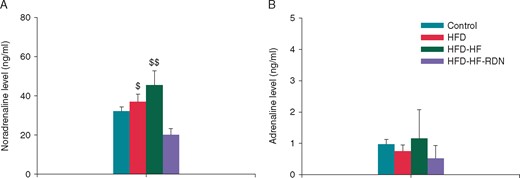
Comparisons of renal catecholamine levels among the four groups. (A) Noradrenaline level. (B) Adrenaline level. HF, heart failure; HFD, high-fat diet; RDN, renal denervation. $P < 0.05 vs. HFD-HF-RDN and $$P < 0.01 vs. HFD-HF-RDN.
Tissue fibrosis in ventricle and atrium after renal denervation
To assess fibrosis progression, both bi-ventricular and bi-atrial tissue samples were evaluated with Masson’s trichrome staining, as shown in Figure 5. The degree of ventricular and atrial fibrosis was extensive in the HFD-HF rabbits compared with those of the control, HFD and HFD-HF-RDN rabbits, respectively. In addition, the degree of cardiac fibrosis in the HFD-HF rabbits was prevented by RDN. However, the degrees of ventricular and atrial fibrosis in the HFD rabbits was extensive compared to those of the control rabbits.
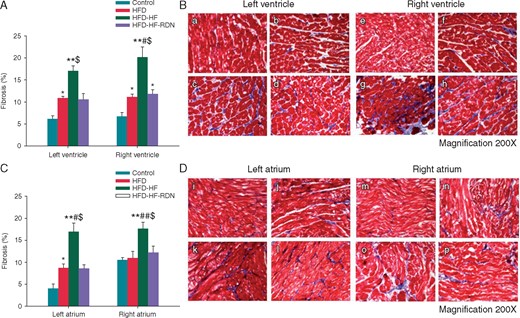
Ventricular and atrial tissue fibrosis among all groups. (A) Quantitative analysis of fibrotic area in the ventricle. (B) The example of Masson’s trichrome staining: (a) control rabbits left ventricle; (b) HFD rabbits left ventricle; (c) HFD-HF rabbits left ventricle; (d) HFD-HF-RDN rabbits left ventricle; (e) control rabbits right ventricle; (f) HFD rabbits right ventricle; (g) HFD-HF rabbits right ventricle; and (h) HFD-HF-RDN rabbits right ventricle. (C) Quantitative analysis of fibrotic area in the atrium. (D) The example of Masson’s trichrome staining: (i) control rabbits left atrium; (j) HFD rabbits left atrium; (k) HFD-HF rabbits left atrium; (l) HFD-HF-RDN rabbits left atrium; (m) control rabbits right atrium; (n) HFD rabbits right atrium; (o) HFD-HF rabbits right atrium; and (p) HFD-HF-RDN rabbits right atrium. HF, heart failure; HFD, high-fat diet; RDN, renal denervation. *P<0.05 vs. Control, **P<0.01 vs. Control, #P < 0.05 vs. HFD, ##P < 0.01 vs. HFD and $P < 0.05 vs. HFD-HF-RDN.
Immunohistochemistry staining
The results of the histological studies from the myocardium with TH staining of the four groups are shown in Figure 6. Sympathetic neuron marker densities in bi-ventricle were significantly lower in the control or HFD-HF-RDN rabbits compared with those of the HFD and HFD-HF rabbits, respectively. However, there were no significant differences in bi-ventricular sympathetic neuron marker densities between the control and HFD-HF-RDN rabbits.
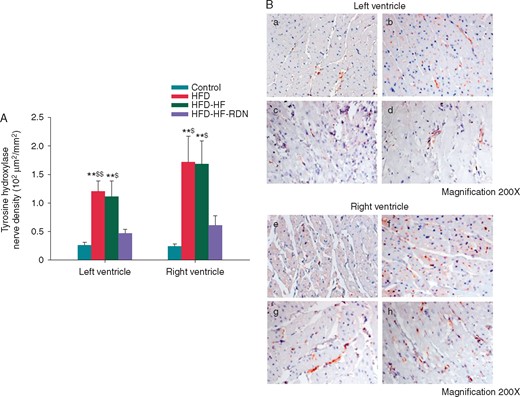
Cardiac sympathetic nerve activities in the ventricle. (A) Sympathetic neuron marker densities among all groups. (B) The example of TH staining. (a) Control rabbits left ventricle; (b) HFD rabbits left ventricle; (c) HFD-HF rabbits left ventricle; (d) HFD-HF-RDN rabbits left ventricle; (e) Control rabbits right ventricle; (f) HFD rabbits right ventricle; (g) HFD-HF rabbits right ventricle; and (h) HFD-HF-RDN rabbits right ventricle. HF, heart failure; HFD, high-fat diet; RDN, renal denervation. **P<0.01 vs. Control, $P < 0.05 vs. HFD-HF-RDN and $$P<0.01 vs. HFD-HF-RDN.
We also evaluated TH staining of the stellate ganglion. Sympathetic neuron marker densities were significantly higher in the HFD and HFD-HF rabbits compared with those of the control rabbits, respectively. However, there were no significant differences in sympathetic neuron marker densities between the control and HFD-HF-RDN rabbits (Figure 7).
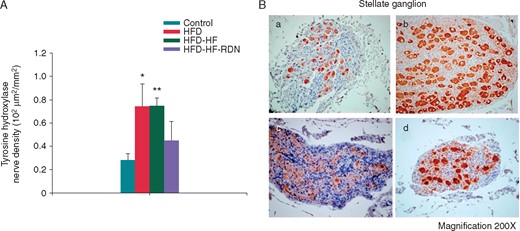
Sympathetic nerve activities in the left stellate ganglion. (A) Sympathetic neuron marker densities among all groups. (B) The example of TH staining: (a) control rabbits stellate ganglion; (b) HFD rabbits stellate ganglion; (c) HFD-HF rabbits stellate ganglion; and (d) HFD-HF-RDN rabbits stellate ganglion. HF, heart failure; HFD, high-fat diet; RDN, renal denervation. *P<0.05 vs. Control and **P<0.01 vs. Control.
Discussion
In the present study, severe ventricular fibrosis, increased cardiac sympathetic activity, and high VF inducibility were observed in the HFD rabbits. Therefore, we demonstrated that overweight is associated with increase of ventricular arrhythmogenesis, possibly through cardiac sympathetic activation. In the HFD-HF rabbits, prolongation of ventricular ERP, severe ventricular fibrosis, and high VF inducibility were noted. In the HFD-HF-RDN rabbits, ventricular ERP was not shortened, but the degree of ventricular fibrosis and VF inducibility were significantly suppressed when compared with the HFD-HF rabbits. Additionally, cardiac sympathetic activity was also significantly attenuated by RDN. Therefore, RDN can prevent VF inducibility through reverse structural and autonomic remodelling.
Cardiac remodelling in overweight and heart failure
Overweight and obesity are considered to be a risk factor of cardiac remodelling, which may lead to life-threatening ventricular arrhythmia and sudden cardiac death.5 However, the histological and electrophysiological characteristics of the heart in overweight have not yet been fully examined. In the present study, the HFD rabbits demonstrated the extension of ventricular and atrial fibrosis compared with those of the control rabbits. These results indicate that increased body weight developed ventricular and atrial structural remodelling. Overweight and obese patients are known to experience concomitant cardiovascular diseases, such as hypertension, coronary artery disease, and HF.2 However, sympathetic activity, structural remodelling, and effect of RDN on VF inducibility in overweight with HF have not been fully elucidated. Therefore, we also studied these issues in the present study. As a result, the extension of ventricular and atrial fibrosis was observed in the HFD-HF rabbits as compared to the control and HFD rabbits. These results suggest that the combination of overweight and HF leads to more advanced structural remodelling.
Sympathetic nerve stimulation is considered to decrease ventricular ERP and action potential duration.13 However, Li et al.14 reported that rapid pacing-induced HF was associated with prolongation of ventricular ERP, and structural remodelling might be a factor in electrophysiological change. Indeed, the ventricular and atrial ERPs of the HFD-HF rabbits in the current study were significantly longer than those of the controls, whereas the differences of ERPs in the control and HFD rabbits were not statistically significant. One possible reason for this result is that the degree of increased body weight in our study was not enough to induce electrophysiological change, although structural remodelling occurred.
Ventricular fibrillation inducibility in overweight and heart failure
Hyperactivity of the sympathetic nervous system leads to ventricular arrhythmia and sudden cardiac death. Additionally, cardiac sympathetic nerve activity contributes to the pathogenesis of ventricular arrhythmias.11 In overweight and obesity, leptin, which is produced by adipose tissue, appears to play a pivotal role in cardiac sympathetic nerve activation.15 Therefore, hyperactivity of cardiac sympathetic nervous system in overweight and obesity may be associated with the genesis of ventricular arrhythmia. In the present study, an increased cardiac sympathetic activity was observed in the HFD and HFD-HF rabbits compared with the control rabbits. In addition, TH staining of the stellate ganglion support that sympathetic neurons in animals with RDN might be damaged in accordance with a study by Tsai et al.16 Therefore, it was possible that VF inducibility was higher in the HFD and HFD-HF rabbits compared with the control rabbits, whereas VF inducibility of the HFD-HF-RDN and control rabbits was similar.
In HF status, cardiac sympathetic activity is initially increased, and then renal sympathetic activity is increased.17 In the present study, renal catecholamine levels in the control and HFD-HF rabbits were similar. Therefore, our study models might be at an early stage of progressive HF. Nevertheless, high VF inducibility was observed in the HFD-HF rabbits.
Effect of renal denervation on ventricular tachyarrhythmia
RDN may be an effective therapy for the management of ventricular arrhythmia because it can regulate sympathetic activity and ventricular electrical remodelling.11,12,18 We previously reported that RDN suppressed cardiac sympathetic nerve activity and the development of cardiac fibrosis in HF models paced for 4 weeks.10 We additionally examined the data (some are unpublished data) in those models. When compared with HF models paced for 4 weeks,10 mean sympathetic neuron marker densities in the left ventricle (1.1 ± 0.3 and 0.8 ± 0.1 × 102 μm2/mm2), degree of tissue fibrosis in the left ventricle (17.0 ± 1.1 and 13.6 ± 1.6%), and VF inducibility (58 ± 10% and 41 ± 9%) were higher in the HFD-HF rabbits, respectively. Nevertheless, in the present study, the levels of ventricular TH staining, renal noradrenaline, and cardiac fibrosis were decreased by RDN in the HFD-HF-RDN rabbits compared to those in the HFD-HF rabbits. Moreover, VF inducibility was also suppressed by RDN. Therefore, we demonstrated that RDN can suppress VF inducibility through sympathetic nerve modulation and reverse structural remodelling in overweight with HF. The ventricular and atrial ERPs of the HFD-HF-RDN rabbits were slightly longer than those of the controls. It has been reported that RDN prolonged ventricular ERP and action potential duration, reduced the dispersion of ventricular ERP and the restitution curve slope, because RDN might decrease sympathetic effects on the heart.19 In our study, decreased catecholamine levels in the HFD-HF-RDN rabbits might have been associated with ventricular ERP prolongation. These findings suggest that the similar electrophysiological changes between HFD-HF and HFD-HF-RDN rabbits actually represented different underlying mechanisms.
Obese patients are more likely to suffer concomitant HF, and the combination of obesity and HF is associated with a high risk of sudden death.3 From this study, we found that patients with obesity and HF might have the progressive structural remodelling and hyperactivity of the sympathetic nervous system, resulting in an increased risk of sudden cardiac death. Although there are convincing proofs that β blockade has cardioprotective effects in HF, Wang et al.20 reported that RDN had non-inferior effects on myocardial fibrosis and the activation of oxidative stress and renin–angiotensin system compared with beta-blocker therapy. In addition, they also showed that beneficial effects of RDN were independent of blood pressure in contrast to beta-blocker therapy. Therefore, RDN might be an alternative therapeutic strategy in selected patients, particularly when beta-blocker therapy has been ineffective or not tolerated.
Limitations
In the current study, the sample size was small and the follow-up period was limited. However, we were able to demonstrate that overweight with HF during a 3-month follow-up extended cardiac fibrosis and increased cardiac sympathetic activity, whereas RDN prevented the development of structural and autonomic remodelling. Next, effects of RDN on the other pro-VF factors were not fully investigated. In addition, HF alone was not tested in our study. Since the relation of obesity and HF is rather complicated, different results may be obtained from different HF status and the degree of obesity. Thus, our next step is to examine relative contribution of obesity and HF in alterations of sympathetic activity, structural remodelling, and VF inducibility. Finally, we did not measure blood pressure which might play an important role in cardiac changes. Therefore, it remains unclear whether the cardiac changes are a result of an increased blood pressure, the direct action of catecholamines or a combination. However, RDN may have a great potential in the treatment of HF independent of the blood pressure lowering effect.9,20 In addition, the effect of RDN on cardiac remodelling and arrhythmias might be better than that in antihypertensive treatment using angiotensin-converting-enzyme inhibitors and angiotensin receptor blockers because RDN can regulate not only renin–angiotensin–aldosterone system but also sympathetic nervous system10,11 Furthermore, blood pressure only can be measured under anaesthesia condition with arterial line monitoring, and the blood pressure during general anaesthesia may not reflect the blood pressure during ambulatory status. Therefore, we did not record blood pressure in the present study.
Conclusion
Overweight and HF are related to the development of structural remodelling and the hyperactivity of the cardiac sympathetic nervous system, resulting in high VF inducibility. The reverse structural remodelling and the sympathetic nerve modulation are pivotal mechanisms of RDN in the protection of the heart from overweight and HF.
Conflict of interest: none declared.
Funding
This work was supported by the Taipei Veterans General Hospital (V104C-131, V104E7-003, V105C-60, V106C-114, V107C-099, and VGHUST107-G1-8–1), Ministry of Science and Technology (MOST 104-2314-B-075-065-MY2, MOST 106-2314-B-010-054), and Research Foundation of Cardiovascular Medicine (RFCM 101-01-001, 104-01-009-01).