-
PDF
- Split View
-
Views
-
Cite
Cite
Philippe Ritter, Gabor Z. Duray, Shu Zhang, Calambur Narasimhan, Kyoko Soejima, Razali Omar, Verla Laager, Kurt Stromberg, Eric Williams, Dwight Reynolds, for the Micra Transcatheter Pacing Study Group, The rationale and design of the Micra Transcatheter Pacing Study: safety and efficacy of a novel miniaturized pacemaker, EP Europace, Volume 17, Issue 5, May 2015, Pages 807–813, https://doi.org/10.1093/europace/euv026
- Share Icon Share
Abstract
Recent advances in miniaturization technologies and battery chemistries have made it possible to develop a pacemaker small enough to implant within the heart while still aiming to provide similar battery longevity to conventional pacemakers. The Micra Transcatheter Pacing System is a miniaturized single-chamber pacemaker system that is delivered via catheter through the femoral vein. The pacemaker is implanted directly inside the right ventricle of the heart, eliminating the need for a device pocket and insertion of a pacing lead, thereby potentially avoiding some of the complications associated with traditional pacing systems.
The Micra Transcatheter Pacing Study is currently undergoing evaluation in a prospective, multi-site, single-arm study. Approximately 720 patients will be implanted at up to 70 centres around the world. The study is designed to have a continuously growing body of evidence and data analyses are planned at various time points. The primary safety and efficacy objectives at 6-month post-implant are to demonstrate that (i) the percentage of Micra patients free from major complications related to the Micra system or implant procedure is significantly higher than 83% and (ii) the percentage of Micra patients with both low and stable thresholds is significantly higher than 80%. The safety performance benchmark is based on a reference dataset of 977 subjects from 6 recent pacemaker studies.
The Micra Transcatheter Pacing Study will assess the safety and efficacy of a miniaturized, totally endocardial pacemaker in patients with an indication for implantation of a single-chamber ventricular pacemaker.
NCT02004873.
Transcatheter pacing system
Novel delivery approach for pacemaker
Background
Since their introduction in the 1960s, implantable pacemakers have steadily shrunk in size and grown in sophistication, yet their basic function remains the same: provision of a more normal heart rate when it is too slow. Pacemaker treatment for bradycardia is frequently used, with more than 600 000 people worldwide receiving a cardiac pacemaker each year.1 Pacemakers remain the only known, long-term effective treatment for bradycardia.
Until now, pacing systems have remained much the same in their deployment since originally used more than a half century ago. The conventional pacing system consists of a pacemaker device containing the electronics and battery typically implanted in a subcutaneous pocket in the chest region, and one or more leads advanced from the device pocket through veins into the heart, that delivers the pacing therapy to the desired pacing site. Patients may encounter pocket complications such as haematoma, erosion/infection, and unaesthetic wounds, especially after several battery changes. Lead complications, such as dislodgement, breakage, venous occlusion, and infection, are major drawbacks of this technology and can be life-threatening. These complications frequently require reoperations with substantial risk, especially lead extractions. Lead dislodgements range from 0.7 to 5.6%.2 Pacemaker infection rates range between 0.1 (initial implant) and 3.5% (pacemaker replacement)3 with the majority originating at the device pocket.
Technological advances in electronics miniaturization and battery chemistries have enabled a device small enough to implant within the heart while still aiming to provide similar battery longevity, and without any lead.
The Micra® Transcatheter Pacing System (Medtronic Inc.) is a miniaturized single-chamber pacemaker system that is delivered via catheter through the femoral vein, and is implanted directly inside the right ventricle of the heart. It eliminates the need for a device pocket and insertion of a pacing lead, thereby potentially avoiding complications associated with traditional pacing systems.
Pre-clinical testing showed low pacing thresholds in animals,4 adequate fixation in the highly trabeculated human right ventricle,5 and accurate measurement of electrical values.6 This study will assess the safety and efficacy of Micra in humans.
Methods
Study device
The Micra implantable device is a self-contained, hermetically enclosed, miniaturized single-chamber pacemaker. The device is fixated via four electrically inactive extendable/retractable nitinol tines, located on the distal end of the device (Figures 1 and 2). Despite the differences in size and shape, the device functionality and features are similar to existing single-chamber pacemakers (Table 1).
Attribute . | Medtronic Adapta ADSR01 . | Medtronic Micra . |
---|---|---|
Size | ||
Volume of device (cm3) | 9.7 | 0.8 |
Mass of device (g) | 21.5 | 2.0 |
Total volume of material within body (cm3) | 10.56 (assumes Adapta device + 30 cm, 6 Fr lead) | 0.8 (device with electrodes placed directly on the pacemaker capsule) |
Features | ||
Battery chemistry/capacity | Lithium-iodine, 830 mA h | Lithium silver vanadium oxide/carbon monoflouride, 120 mA h |
Threshold measurement and tracking | Measure 1/day Output = maximum of 2× threshold or 2.0 V | Measure 1/day, verify 1/h Output = threshold + 0.5 V |
Capture management | Evoked response (all leads) Works at 0.4 ms | Evoked response (one pair of low polarization, 18 mm spaced electrodes) Works at 0.24 and 0.4 ms |
Prolonged service period | 3 months | 6 months |
Magnet mode | Yes | No |
Rate response | SubQ accelerometer, (activity) | Cardiac three-axis accelerometer, (activity), individually selectable with one vector used |
Ability to inactivate device at EOS | No | Device can be manually deactivated with programmer and automatically deactives at EOS |
MRI conditional | No | Yes, by design |
Electrodes | Lead dependent | Pacing electrodes placed directly on the pacemaker capsule: Cathode: 2.5 mm2 TiN-coated and sintered, located at tip. Anode: 22 mm2 TiN-coated, located on ring on body |
Steroid elution | Lead dependent | Yes |
Attribute . | Medtronic Adapta ADSR01 . | Medtronic Micra . |
---|---|---|
Size | ||
Volume of device (cm3) | 9.7 | 0.8 |
Mass of device (g) | 21.5 | 2.0 |
Total volume of material within body (cm3) | 10.56 (assumes Adapta device + 30 cm, 6 Fr lead) | 0.8 (device with electrodes placed directly on the pacemaker capsule) |
Features | ||
Battery chemistry/capacity | Lithium-iodine, 830 mA h | Lithium silver vanadium oxide/carbon monoflouride, 120 mA h |
Threshold measurement and tracking | Measure 1/day Output = maximum of 2× threshold or 2.0 V | Measure 1/day, verify 1/h Output = threshold + 0.5 V |
Capture management | Evoked response (all leads) Works at 0.4 ms | Evoked response (one pair of low polarization, 18 mm spaced electrodes) Works at 0.24 and 0.4 ms |
Prolonged service period | 3 months | 6 months |
Magnet mode | Yes | No |
Rate response | SubQ accelerometer, (activity) | Cardiac three-axis accelerometer, (activity), individually selectable with one vector used |
Ability to inactivate device at EOS | No | Device can be manually deactivated with programmer and automatically deactives at EOS |
MRI conditional | No | Yes, by design |
Electrodes | Lead dependent | Pacing electrodes placed directly on the pacemaker capsule: Cathode: 2.5 mm2 TiN-coated and sintered, located at tip. Anode: 22 mm2 TiN-coated, located on ring on body |
Steroid elution | Lead dependent | Yes |
Attribute . | Medtronic Adapta ADSR01 . | Medtronic Micra . |
---|---|---|
Size | ||
Volume of device (cm3) | 9.7 | 0.8 |
Mass of device (g) | 21.5 | 2.0 |
Total volume of material within body (cm3) | 10.56 (assumes Adapta device + 30 cm, 6 Fr lead) | 0.8 (device with electrodes placed directly on the pacemaker capsule) |
Features | ||
Battery chemistry/capacity | Lithium-iodine, 830 mA h | Lithium silver vanadium oxide/carbon monoflouride, 120 mA h |
Threshold measurement and tracking | Measure 1/day Output = maximum of 2× threshold or 2.0 V | Measure 1/day, verify 1/h Output = threshold + 0.5 V |
Capture management | Evoked response (all leads) Works at 0.4 ms | Evoked response (one pair of low polarization, 18 mm spaced electrodes) Works at 0.24 and 0.4 ms |
Prolonged service period | 3 months | 6 months |
Magnet mode | Yes | No |
Rate response | SubQ accelerometer, (activity) | Cardiac three-axis accelerometer, (activity), individually selectable with one vector used |
Ability to inactivate device at EOS | No | Device can be manually deactivated with programmer and automatically deactives at EOS |
MRI conditional | No | Yes, by design |
Electrodes | Lead dependent | Pacing electrodes placed directly on the pacemaker capsule: Cathode: 2.5 mm2 TiN-coated and sintered, located at tip. Anode: 22 mm2 TiN-coated, located on ring on body |
Steroid elution | Lead dependent | Yes |
Attribute . | Medtronic Adapta ADSR01 . | Medtronic Micra . |
---|---|---|
Size | ||
Volume of device (cm3) | 9.7 | 0.8 |
Mass of device (g) | 21.5 | 2.0 |
Total volume of material within body (cm3) | 10.56 (assumes Adapta device + 30 cm, 6 Fr lead) | 0.8 (device with electrodes placed directly on the pacemaker capsule) |
Features | ||
Battery chemistry/capacity | Lithium-iodine, 830 mA h | Lithium silver vanadium oxide/carbon monoflouride, 120 mA h |
Threshold measurement and tracking | Measure 1/day Output = maximum of 2× threshold or 2.0 V | Measure 1/day, verify 1/h Output = threshold + 0.5 V |
Capture management | Evoked response (all leads) Works at 0.4 ms | Evoked response (one pair of low polarization, 18 mm spaced electrodes) Works at 0.24 and 0.4 ms |
Prolonged service period | 3 months | 6 months |
Magnet mode | Yes | No |
Rate response | SubQ accelerometer, (activity) | Cardiac three-axis accelerometer, (activity), individually selectable with one vector used |
Ability to inactivate device at EOS | No | Device can be manually deactivated with programmer and automatically deactives at EOS |
MRI conditional | No | Yes, by design |
Electrodes | Lead dependent | Pacing electrodes placed directly on the pacemaker capsule: Cathode: 2.5 mm2 TiN-coated and sintered, located at tip. Anode: 22 mm2 TiN-coated, located on ring on body |
Steroid elution | Lead dependent | Yes |
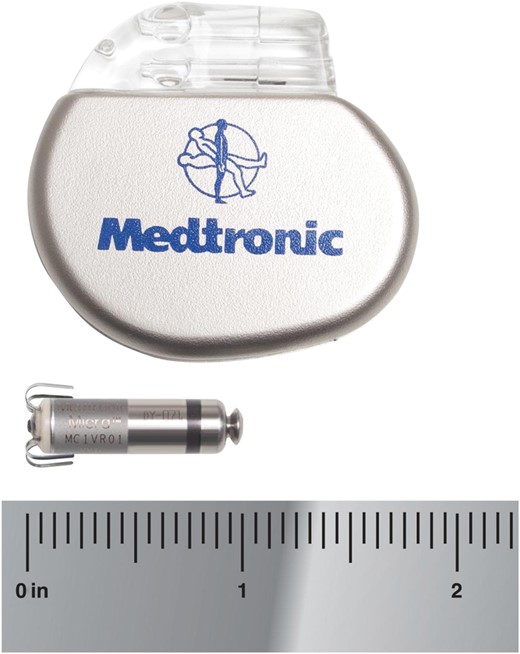
Miniaturized Micra technology: size of Micra compared with conventional pacemaker.
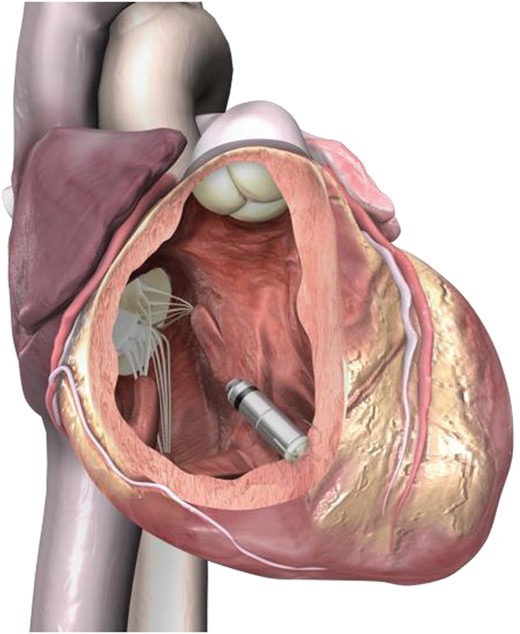
Micra reduces device energy needs while maintaining ability to deliver therapy. The primary differences are a three-axis accelerometer sensor (vs. a single axis) to allow the physician to select an alternate axis to sense activity, capture management with automatic hourly safety margin confirmation to ensure pacing outputs remain at safe levels, and an end-of-service (EOS) operation to allow subsequent implants without requiring a Micra explant. (The device can be permanently programmed ‘OFF to OOO mode’, and when the battery voltage reaches the EOS condition, the device automatically switches to the device ‘OFF to OOO mode’.) In addition, the Micra device battery longevity is optimized by setting the nominal pulse width duration to chronaxie (0.24 ms).7 Micra is estimated to have an average battery life of ∼10 years, assuming 1.5 V output, 600 Ω, 60 b.p.m., 100% pacing, and 0.24 ms.
A single use transfemoral catheter and an introducer sheath (23Fr ID) are used to deliver, deploy, and test the device placement. The standard Medtronic Model 2090 Programmer achieves device communication.
Study population, inclusion, and exclusion criteria
Up to 720 patients will be implanted at up to 70 centres worldwide. The inclusion and exclusion criteria are in Table 2. Patients with Class I or II indications for pacing are eligible. Patients who have previously received a cardiac implantable device are excluded from the study. Patients who are entirely pacemaker dependent (defined as escape rhythm <30 b.p.m.) are excluded from the initial portion of the study, until device reliability is verified from ambulatory ECG and device diagnostic data from 25 patients at 1-month post-implant.
Inclusion criteria |
Class I or II indication for implantation of a single-chamber ventricular pacemaker2–4 |
Able to undergo the study requirements |
18 years of age (or older, if required by local law) |
Exclusion criteria |
Entirely pacemaker dependenta (defined as escape rhythm ≤30 b.p.m.) |
Existing or prior pacemaker, ICD, or CRT device implant |
Unstable angina pectoris, acute myocardial infarction within 30 days |
Current implantation of neurostimulator or any other chronically implanted device which uses current |
Mechanical tricuspid valve, implanted vena cava filter, or left ventricular assist device |
Morbidly obese where telemetry communication of 12.5 cm cannot be obtained with programmer |
Femoral venous anatomy unable to accommodate a 23 French introducer sheath or implant on the right side of the heart |
Unable to tolerate urgent sternotomy |
Known intolerance to nickel–titanium (nitinol) alloy |
Contraindication for single dose of 1.0 mg dexamethasone acetate |
Life expectancy <12 months |
Enrolment in concurrent confounding study |
Pregnant or breastfeeding women |
Inclusion criteria |
Class I or II indication for implantation of a single-chamber ventricular pacemaker2–4 |
Able to undergo the study requirements |
18 years of age (or older, if required by local law) |
Exclusion criteria |
Entirely pacemaker dependenta (defined as escape rhythm ≤30 b.p.m.) |
Existing or prior pacemaker, ICD, or CRT device implant |
Unstable angina pectoris, acute myocardial infarction within 30 days |
Current implantation of neurostimulator or any other chronically implanted device which uses current |
Mechanical tricuspid valve, implanted vena cava filter, or left ventricular assist device |
Morbidly obese where telemetry communication of 12.5 cm cannot be obtained with programmer |
Femoral venous anatomy unable to accommodate a 23 French introducer sheath or implant on the right side of the heart |
Unable to tolerate urgent sternotomy |
Known intolerance to nickel–titanium (nitinol) alloy |
Contraindication for single dose of 1.0 mg dexamethasone acetate |
Life expectancy <12 months |
Enrolment in concurrent confounding study |
Pregnant or breastfeeding women |
aRestriction removed after device reliability is verified.
Inclusion criteria |
Class I or II indication for implantation of a single-chamber ventricular pacemaker2–4 |
Able to undergo the study requirements |
18 years of age (or older, if required by local law) |
Exclusion criteria |
Entirely pacemaker dependenta (defined as escape rhythm ≤30 b.p.m.) |
Existing or prior pacemaker, ICD, or CRT device implant |
Unstable angina pectoris, acute myocardial infarction within 30 days |
Current implantation of neurostimulator or any other chronically implanted device which uses current |
Mechanical tricuspid valve, implanted vena cava filter, or left ventricular assist device |
Morbidly obese where telemetry communication of 12.5 cm cannot be obtained with programmer |
Femoral venous anatomy unable to accommodate a 23 French introducer sheath or implant on the right side of the heart |
Unable to tolerate urgent sternotomy |
Known intolerance to nickel–titanium (nitinol) alloy |
Contraindication for single dose of 1.0 mg dexamethasone acetate |
Life expectancy <12 months |
Enrolment in concurrent confounding study |
Pregnant or breastfeeding women |
Inclusion criteria |
Class I or II indication for implantation of a single-chamber ventricular pacemaker2–4 |
Able to undergo the study requirements |
18 years of age (or older, if required by local law) |
Exclusion criteria |
Entirely pacemaker dependenta (defined as escape rhythm ≤30 b.p.m.) |
Existing or prior pacemaker, ICD, or CRT device implant |
Unstable angina pectoris, acute myocardial infarction within 30 days |
Current implantation of neurostimulator or any other chronically implanted device which uses current |
Mechanical tricuspid valve, implanted vena cava filter, or left ventricular assist device |
Morbidly obese where telemetry communication of 12.5 cm cannot be obtained with programmer |
Femoral venous anatomy unable to accommodate a 23 French introducer sheath or implant on the right side of the heart |
Unable to tolerate urgent sternotomy |
Known intolerance to nickel–titanium (nitinol) alloy |
Contraindication for single dose of 1.0 mg dexamethasone acetate |
Life expectancy <12 months |
Enrolment in concurrent confounding study |
Pregnant or breastfeeding women |
aRestriction removed after device reliability is verified.
Study procedures and implant
Periprocedural anticoagulation management is determined according to implanter discretion. Patients are implanted following the instructions in the implant manual. Insertion, positioning, and deployment of the device are guided by fluoroscopy. Adequate device fixation is verified by ensuring device tines are engaged firmly in the cardiac tissue (by ‘tug’ or ‘pull and hold’ test under X-ray), and electrical measurements are within recommended values (pacing threshold at 0.24 ms <1.0 V, pacing impedance 400–1500 Ω, and R-wave amplitude >5 mV). A tether is attached to the device and provides the mechanism for retention and re-capture of the Micra device during implant. The device is relocated until targeted values are obtained. Data collection includes device interrogations, ambulatory ECG recordings, and exercise testing (Table 3).
Procedure . | Baseline . | Implant . | Pre-discharge . | 1 month . | 3 months . | 6 months . | Recurring 6 months . | Unscheduled visit . |
---|---|---|---|---|---|---|---|---|
All subjects | ||||||||
Informed consent | ✓ | |||||||
Eligibility criteria | ✓ | |||||||
Chest X-ray | ✓ | ✓ | 12 months | |||||
Fluoroscopy cine motion recordings | ✓ | |||||||
Electrical testing | ✓ | ✓ | ✓ | ✓ | ✓ | ✓ | ||
Programmer strips | ✓ | ✓ | ✓ | |||||
Device interrogation | ✓ | ✓ | ✓ | ✓ | ✓ | ✓ | ✓ | |
Patient questionnaires (EQ-5D, SF-36) | ✓ | ✓ | Annual visits | |||||
Physician questionnaire | ✓ | |||||||
First ∼50 subjects only—incremental data collection | ||||||||
Additional electrical testing and programmer strips | ✓ | ✓ | ✓ | |||||
24 h ambulatory ECGs (Holter) | ✓ | ✓ | ||||||
Short exercise test(s) | ✓ | ✓ | ||||||
Long treadmill test | ✓ | ✓ |
Procedure . | Baseline . | Implant . | Pre-discharge . | 1 month . | 3 months . | 6 months . | Recurring 6 months . | Unscheduled visit . |
---|---|---|---|---|---|---|---|---|
All subjects | ||||||||
Informed consent | ✓ | |||||||
Eligibility criteria | ✓ | |||||||
Chest X-ray | ✓ | ✓ | 12 months | |||||
Fluoroscopy cine motion recordings | ✓ | |||||||
Electrical testing | ✓ | ✓ | ✓ | ✓ | ✓ | ✓ | ||
Programmer strips | ✓ | ✓ | ✓ | |||||
Device interrogation | ✓ | ✓ | ✓ | ✓ | ✓ | ✓ | ✓ | |
Patient questionnaires (EQ-5D, SF-36) | ✓ | ✓ | Annual visits | |||||
Physician questionnaire | ✓ | |||||||
First ∼50 subjects only—incremental data collection | ||||||||
Additional electrical testing and programmer strips | ✓ | ✓ | ✓ | |||||
24 h ambulatory ECGs (Holter) | ✓ | ✓ | ||||||
Short exercise test(s) | ✓ | ✓ | ||||||
Long treadmill test | ✓ | ✓ |
This table is intended to highlight key elements of study visits and does not include all study and sub-study requirements.
Procedure . | Baseline . | Implant . | Pre-discharge . | 1 month . | 3 months . | 6 months . | Recurring 6 months . | Unscheduled visit . |
---|---|---|---|---|---|---|---|---|
All subjects | ||||||||
Informed consent | ✓ | |||||||
Eligibility criteria | ✓ | |||||||
Chest X-ray | ✓ | ✓ | 12 months | |||||
Fluoroscopy cine motion recordings | ✓ | |||||||
Electrical testing | ✓ | ✓ | ✓ | ✓ | ✓ | ✓ | ||
Programmer strips | ✓ | ✓ | ✓ | |||||
Device interrogation | ✓ | ✓ | ✓ | ✓ | ✓ | ✓ | ✓ | |
Patient questionnaires (EQ-5D, SF-36) | ✓ | ✓ | Annual visits | |||||
Physician questionnaire | ✓ | |||||||
First ∼50 subjects only—incremental data collection | ||||||||
Additional electrical testing and programmer strips | ✓ | ✓ | ✓ | |||||
24 h ambulatory ECGs (Holter) | ✓ | ✓ | ||||||
Short exercise test(s) | ✓ | ✓ | ||||||
Long treadmill test | ✓ | ✓ |
Procedure . | Baseline . | Implant . | Pre-discharge . | 1 month . | 3 months . | 6 months . | Recurring 6 months . | Unscheduled visit . |
---|---|---|---|---|---|---|---|---|
All subjects | ||||||||
Informed consent | ✓ | |||||||
Eligibility criteria | ✓ | |||||||
Chest X-ray | ✓ | ✓ | 12 months | |||||
Fluoroscopy cine motion recordings | ✓ | |||||||
Electrical testing | ✓ | ✓ | ✓ | ✓ | ✓ | ✓ | ||
Programmer strips | ✓ | ✓ | ✓ | |||||
Device interrogation | ✓ | ✓ | ✓ | ✓ | ✓ | ✓ | ✓ | |
Patient questionnaires (EQ-5D, SF-36) | ✓ | ✓ | Annual visits | |||||
Physician questionnaire | ✓ | |||||||
First ∼50 subjects only—incremental data collection | ||||||||
Additional electrical testing and programmer strips | ✓ | ✓ | ✓ | |||||
24 h ambulatory ECGs (Holter) | ✓ | ✓ | ||||||
Short exercise test(s) | ✓ | ✓ | ||||||
Long treadmill test | ✓ | ✓ |
This table is intended to highlight key elements of study visits and does not include all study and sub-study requirements.
Study endpoints
The study will assess early and long-term performance. Primary and secondary endpoints will be analysed at 6-month follow-up. The long-term safety objective will be evaluated at 12-month follow-up.
Early performance assessment
The study will feature multiple assessments at various timepoints. The early performance objectives will be evaluated after 60 subjects (including the first 25 patients who undergo ambulatory ECG recording for lifting the exclusion indication criterion of pacemaker dependency) have been followed for 3 months.
The first early evaluation objective demonstrates freedom from unanticipated serious adverse event rate at 3 months is significantly >85%. The second early performance objective demonstrates the mean pacing capture threshold at 3 months is significantly <2 V at 0.24 ms. Both early performance objectives will, if met, ensure that the Micra system is operating as intended. These objectives are intentionally different from the study's primary objectives in order to maintain the validity of the statistical inference related to the study's primary objectives.
Primary and secondary endpoints at 6-month follow-up
The Micra Transcatheter Pacing study has two primary endpoints: (i) a safety endpoint to assess freedom from major complications and (ii) effectiveness endpoint to evaluate pacing capture thresholds (Table 4).
Safety | Freedom from major complicationsa related to the Micra transcatheter pacing system and/or procedures at 6-month post-implant (within 183 days) >83% |
Efficacy | At the month 6 visit, >80% of subjects with a pacing capture threshold is low (≤2 V at a pulse width of 0.24 ms) and stable (increase of ≤1.5 V) |
Safety | Freedom from major complicationsa related to the Micra transcatheter pacing system and/or procedures at 6-month post-implant (within 183 days) >83% |
Efficacy | At the month 6 visit, >80% of subjects with a pacing capture threshold is low (≤2 V at a pulse width of 0.24 ms) and stable (increase of ≤1.5 V) |
aMajor complication is defined as any complication related to the Micra system or procedure that results in death, permanent loss of device function due to a mechanical or electrical malfunction, hospitalization, prolongation of hospitalization for 48 or more hours, or system change (removal, repositioning, or replacement).
Safety | Freedom from major complicationsa related to the Micra transcatheter pacing system and/or procedures at 6-month post-implant (within 183 days) >83% |
Efficacy | At the month 6 visit, >80% of subjects with a pacing capture threshold is low (≤2 V at a pulse width of 0.24 ms) and stable (increase of ≤1.5 V) |
Safety | Freedom from major complicationsa related to the Micra transcatheter pacing system and/or procedures at 6-month post-implant (within 183 days) >83% |
Efficacy | At the month 6 visit, >80% of subjects with a pacing capture threshold is low (≤2 V at a pulse width of 0.24 ms) and stable (increase of ≤1.5 V) |
aMajor complication is defined as any complication related to the Micra system or procedure that results in death, permanent loss of device function due to a mechanical or electrical malfunction, hospitalization, prolongation of hospitalization for 48 or more hours, or system change (removal, repositioning, or replacement).
Primary safety endpoint
Major complications are defined as events related to the Micra system or procedure resulting in death, permanent loss of device function due to mechanical or electrical dysfunction, hospitalization, prolonged hospitalization by at least 48 h, or system revision. The primary safety objective will be met if the freedom from major complications related to the Micra system or procedure at 6 months is significantly higher than 83% (i.e. lower boundary of confidence interval must be >83%).
Micra performance expectations were based on Medtronic analyses of six recent studies of dual-chamber pacing systems since recent pacing studies have only been conducted on dual-chamber systems: 3830,85076,9 EnRhythm,10 EnRhythm MRI,11 Advisa MRI,12 and SAVEPACe13 (Figure 3). As the primary difference between complication rates in dual- vs. single-chamber systems is due to the right atrial lead, the single-chamber complication rates were estimated by excluding any complications due to the RA lead. Patients (n = 977) with atrial fibrillation from these six pacemaker studies were selected to benchmark the safety performance goal to reflect a single-chamber patient population. Figure 3 displays Kaplan–Meier estimates of the major complication freedom rate at 6 months and 95% confidence intervals were used to set the performance benchmark. This reference dataset was corroborated by the recent Danish registry,14 where 6-month complication rates for single-chamber pacing systems were as high as 10%, higher than generally acknowledged.
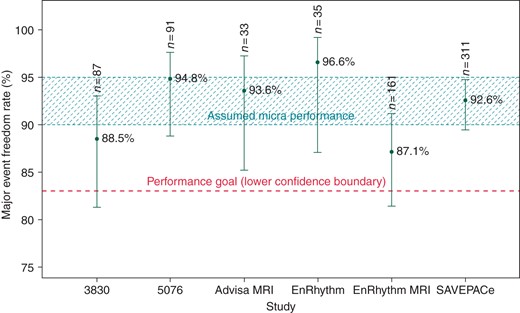
Freedom from major system or procedure complications at 6-month post-implant among subjects reporting AF at baseline in previous pacing studies. Notes: n is the number of subjects with AF reported at baseline at risk for a major complication related to the pacemaker system (excluding right atrial lead-related events) and/or procedure.
Primary efficacy endpoint
The primary efficacy objective evaluates adequate pacing capture thresholds at 6 months. A subject must have a pacing capture threshold of ≤2 V at 0.24 ms at 6 months, without increasing by more than 1.5 V from implant. The primary efficacy objective will be met if the percentage of subjects meeting this endpoint is significantly >80% (i.e. lower boundary of the two-sided confidence interval must be >80%). The 80% performance benchmark was established based on existing pacemaker systems (daily pacing capture thresholds within Medtronic's CareLink® network). Meeting this objective will ensure that Micra pacing capture thresholds are stable and device longevity is predictable. Both primary objectives must be met for the study to be successful.
Secondary endpoints
Two secondary endpoints will be examined with pre-specified hypotheses with type I error controlled using the method of Holm. The first secondary endpoint confirms the automated ventricular capture management (VCM) feature by comparing the percentage of subjects with a VCM within ±0.5 V of pacing capture thresholds evaluated manually at 6 months. This objective will be met if the percentage of subjects meeting this endpoint is significantly >85%. The other secondary objective is to confirm rate response during treadmill testing in a subset of subjects. This objective will be met if the mean slope parameter from the Kay–Wilkoff model15 is equivalent to unity.
In addition, Micra longevity estimates at 6 months, adverse events, electrical performance, implant procedure ambulatory ECG monitoring, quality of life, and device orientation will be summarized.
Long-term safety objective at 12-month follow-up
The long-term safety objective will be assessed after all implanted subjects have the opportunity to complete 12-month follow-up. The long-term safety objective will evaluate freedom from major complications related to the Micra system or procedure at 12 months and will be met if the freedom rate is significantly >82% (i.e. lower boundary of the two-sided 95% confidence interval must be >82%).
Study management and event adjudication
The data collection and monitoring are managed by the sponsor. All system or procedure-related adverse events are classified by a Clinical Events Committee, physician experts in cardiology.
A separate Data Monitoring Committee (DMC) will assess accumulating safety data. The DMC is independent from the study sponsor and not involved as study sites. They will evaluate study results from interim analysis of study objectives and may formulate recommendations for data management and quality control.
Statistical considerations
A sample size of 720 successfully implanted subjects provides >90% power to test the study's two primary objectives. Up to 780 subjects will be consented to ensure 720 successful Micra implants. For the primary safety objective, we assumed a Micra system or procedure-related major complication freedom rate of no lower than 90% at 6 months, a two-sided overall α level of 0.05, and an annualized attrition rate of 17%. The sample size assumptions allow for up to three planned analyses of the two primary objectives which will be reviewed by the independent DMC when 300, 450, and 600 implanted subjects complete 6 months. Success boundaries are based on Hwang et al.16 alpha spending function. The primary safety objective compares the 6-month Micra Kaplan–Meier freedom from Micra-related event rate to the performance goal of 83%.
For the primary efficacy objective, we hypothesize that no fewer than 89% of subjects will have a low and stable pacing capture threshold at 6 months and meet the primary efficacy endpoint. An exact binomial test will compare the percentage of subjects meeting the objective to the performance goal of 80%. As the study includes many geographies, the primary endpoints will be assessed for heterogeneity across geography.
The study will be successful at the first analysis where both primary objectives are met. The secondary objectives will then be evaluated at this time point. The study continues to enrol and follow subjects until at least 600 subjects have been followed for at least 12 months to evaluate the long-term objective.
Discussion
Traditional pacing involves the implantation of the pulse generator ‘can’ into a subcutaneous pocket. The device is connected by one or two pacing leads. This same scheme has been used since the 1960s and is intended for patients of all ages. While not common, complications tend to occur with the pocket and the lead, especially in patients who may exhaust several devices during their life. In addition, these patients may require several new leads over time, filling the venous system and risking total occlusion. Infection requires complete explantation of the equipment and is associated with serious risks. The rate of infection increases with the number of procedures,17 therefore, patients who will need multiple devices implanted during their life are at greater danger of high-risk complications. It is therefore attractive to develop a more compact system that can eliminate the subcutaneous pacemaker can, the presence of material in the venous system, and leads.
In 1970, Spickler et al.18 conducted animal research demonstrating the feasibility of a self-contained, capsule-shaped, and leadless pacemaker. Challenges in this era included lack of a power source to provide sufficient longevity, and insufficient fixation and delivery technology.
Unprecedented development work has allowed the implantation of a device directly into the right ventricle via catheterization from the femoral vein. The miniaturized device is only one-tenth the volume of the traditional single-chamber pacemaker (Table 1). Compared with a traditional system, Micra reduces the amount of material in the body by 93%. This order of magnitude miniaturization was obtained by more efficient electronics and pacing to reduce energy consumption, and using higher energy density battery chemistry. Despite the dramatically smaller size, the Micra pacemaker retains all the features of a modern VVIR pacemaker, including comparable longevity to conventional pacemakers based on a lower pulse width at chronaxae8 and capture management with automatic hourly safety margin confirmation. Animal trials have demonstrated the reliability of the device fixation, and the long-term tolerance of the device inside the ventricular cavity with good electrical performance, and therefore, a decrease in the rate of complications including infections is expected.
Micra includes the essential device diagnostics provided on existing devices, such as sensing and pacing capture threshold trends, and pacing/sensing percentage histograms. Estimated device longevity is also provided. Micra technology is compatible with remote monitoring, with availability of home monitors planned in the future although this does not exist on the first iteration.
As Micra is inserted via the distensible femoral venous system rather than the arterial system, the vast majority of patients is expected to be suitable for the large introducer sheath (23ID, 27OD). The device is contraindicated in patients with extreme morbid obesity preventing telemetry communication within ≤12.5 cm.
After the tether is cut and prior to the Micra device being fully encapsulated, the device may be able to be recaptured and repositioned using a retrieval device such as a snare. Device extraction is not recommended after encapsulation although Micra has been extracted in the animal model after 18 months.19 If infection occurs post-encapsulation, the infection is unlikely to be related to Micra and attempting extraction may have higher risk than leaving the device in the heart. If an extraction is performed, operator skillset needs to be considered. In addition, Micra can be permanently programmed off at end of life, to avoid electrical interference with other devices.
There is one other miniaturized pacemaker that also began a US IDE clinical study in 2014. Similar to Micra, the Nanostim™ Leadless Pacemaker is also a self-contained VVIR pacemaker introduced through the femoral vein. Both systems allow for retrievability if needed; however, there are significant differences in designs worth noting: (i) the Micra device has an active fixation mechanism consisting of four electrically inactive extendable–retractable tines to anchor into the cardiac tissue whereas the Nanostim pacemaker uses an electrically active fixed helix, (ii) the Micra device is wider (20 Fr) and shorter (25.9 mm) than the Nanostim pacemaker (18 Fr and 41.4 mm), (iii) Micra communication between the device and programmer is established using a standard programming head whereas the Nanostim pacemaker communicates with the St Jude Medical Merlin™ Patient Care System using a Programmer Link and surface ECG electrodes, and (iv) Micra uses a three-axis accelerometer for rate response whereas the Nanostim pacemaker utilizes a blood temperature sensor.20
The follow-up procedures for management of the Micra device are the same clinical pathways conducted for current pacemakers. Micra does not require a different programmer and does not introduce the need for any new cables. The objectives of the Micra Transcatheter Pacing Study are to assess the safety and efficacy of the system for regulatory approval processes. From a clinical standpoint, according to the current recommendations of cardiac pacing, patients with chronic atrial fibrillation and a concomitant atrio-ventricular conduction disorder, as well as patients with infrequent and paroxysmal conduction disturbances represent a reasonable cohort for this technology. While appropriate concern about engendering pacemaker syndrome is justified in patients with mechanically functioning atria during ventricular only pacing, infrequent need for pacing mitigates those concerns substantially, as would assessment for ventriculo-atrial conduction. Even patients with rare though symptomatic sinus pauses might be considered candidates for this technology, again considering risk of pacemaker syndrome.
In addition to this being a single-chamber ventricular system, we must be aware of a number of limitations in the use of this type of system, such as inability to place the delivery tool in the heart due to major anatomical venous tortuosity or the presence of an inferior vena caval clip/filter (the approach from the upper body is not recommended at this time), the presence of other electrical intracardiac devices or a mechanical tricuspid valve prosthesis already in place. Study design limitations include using historical data for comparison, excluding subjects with existing or previous systems, and limited follow-up duration. Future studies could consider following patients for device lifetime or randomized designs.
Conclusions
In conclusion, the purpose of the Micra Transcatheter Pacing Study is to determine short- and long-term safety and reliability of a novel miniaturized ventricular pacemaker. A positive study would demonstrate clinically acceptable complication-free rates and pacing capture thresholds at 6 months. Consequently, this miniaturized pacemaker may help to reduce the complication rate and hospitalization time and thus healthcare costs. This technology opens the door to the development of more sophisticated systems to complement the range of cardiac devices implanted by a minimally invasive approach.
Funding
This work was supported by Medtronic Bakken Research Center BV, Maastricht, NL, Europe and Medtronic Inc., Minneapolis, MN, USA.
Conflict of interest: P.R. receives honoraria as a scientific consultant and is a co-principal investigator for the Micra Transcatheter Pacing Study. D.R. is a member of the MICRA Advisory board and a member of the speakers bureau for Medtronic. S.Z. receives honoraria from Medtronic. G.Z.D. is currently conducting research sponsored by Medtronic, Biotronik and Boston Scientific and is also a member of the speakers bureau for Medtronic, Biotronik, Johnson and Johnson, St Jude Medical and Bayer. E.W., K.S. and V.L. are employees of Medtronic and are currently conducting this research sponsored by Medtronic. C.N. receives consultant fees and honoraria from Medtronic. K.S. is a consultant from Medtronic Japan, St. Jude Medical Japan, and Boston Scientific Japan. R.O. receives honoraria from Medtronic for consulting fees and for serving as a member of the speakers bureau for Medtronic.