-
PDF
- Split View
-
Views
-
Cite
Cite
Ilse Lenaerts, Ronald B. Driesen, Nerea Hermida Blanco, Patricia Holemans, Hein Heidbüchel, Stefan Janssens, Jean-Luc Balligand, Karin R. Sipido, Rik Willems, Role of nitric oxide and oxidative stress in a sheep model of persistent atrial fibrillation, EP Europace, Volume 15, Issue 5, May 2013, Pages 754–760, https://doi.org/10.1093/europace/eut012
- Share Icon Share
Abstract
Oxidative stress can modulate nitric oxide (NO) signalling pathways. Both pathways have been shown to be involved in the pathophysiology of atrial fibrillation (AF), but data are conflicting. We aimed to characterize the NO-pathway and its relation to oxidative stress in persistent AF in a sheep model.
Persistent AF was induced by rapid atrial pacing for a mean of 136.5 ± 21.7 days. Non-stimulated sheep served as controls. Nicotine adenine dinucleotide phosphate (NADPH) oxidase-stimulated superoxide production was significantly increased in the AF group (+51.3 ± 23.2%, P < 0.01). Although there were no changes in mRNA expression of the different NADPH oxidase subunits, the increased activity was associated with markedly increased protein expression of the NADPH oxidase activator, Rac1 (+26 ± 4.6%, P < 0.05). No differences were seen in superoxide dismutase activity, but glutathione peroxidase activity was lower in the AF group. There was a marked accumulation of 3-nitrotyrosine, a biomarker for peroxynitrite, in atrial tissue of AF animals, as demonstrated by immunohistochemical staining and dot blot analysis (+15.6 ± 1.8%, P < 0.05). Expression of atrial NOS3 mRNA was 24.9 ± 4.4% lower in the AF group vs. control (P < 0.05), while NOS1 and 2 were unchanged. Immunoblot analysis revealed no changes in protein expression. Nitrite/nitrate levels were significantly lower during AF (−24.8 ± 5.8%, P < 0.05).
In a sheep model of persistent AF, NOS3 transcript levels are attenuated and circulating NOx levels decreased. Persistent AF is associated with increased oxidative stress, probably resulting from increased NADPH oxidase activity, without major changes in anti-oxidant capacity of the atrial tissue.
Atrial fibrillation (AF) attenuates atrial NOS3 expression and is associated with decreased circulating NOx levels in a sheep model of persistent AF.
Persistent AF is associated with increased oxidative stress, probably resulting from increased nicotine adenine dinucleotide phosphate (NADPH) oxidase activity, but without significant changes in anti-oxidant capacity of the atrial tissue.
Introduction
Atrial fibrillation (AF) is the most common cardiac arrhythmia and its prevalence will continue to increase over the next decades due to ageing of the population.1 Over the last years the pathophysiology of AF has been the subject of extensive research; however, it still remains incompletely understood. A link between oxidative stress and the pathogenesis of AF has been suggested.2,3 In atrial myocardium of patients with AF, oxidative damage to the myofibrils has been described.4 It was shown that the oxidative damage was mediated through the actions of hydroxyl radicals and peroxynitrite, leading to protein carbonylation and nitrosylation, respectively. As a result, important contractile proteins were modified in AF, including myofibrillar creatine kinase, resulting in its decreased activity. Reduced activity further contributes to the already depressed contractile atrial function during AF. Ascorbate treatment, which is a peroxynitrite scavenger, lowered the incidence of post-operative AF in patients.5 The causal relationship between oxidative stress and AF was further investigated in large animal models of short-term AF.5,6 Rapid atrial pacing for 48 h led to a shortening of the atrial effective refractory period and an associated increase in peroxynitrite formation, both of which could be reversed by treatment with ascorbate.5 More recently, treatment with statins has been shown to prevent AF promotion after 1 week of rapid atrial pacing in dogs.6 This effect was attributed to their anti-oxidant and anti-inflammatory properties. These data suggest that oxidative injury plays a pivotal role in atrial remodelling underlying AF under these experimental conditions.
Oxidative stress occurs as a result of excessive reactive oxygen species production, including superoxide anions. It is worth noting that this increased superoxide production can cause a decrease in the bioavailability of nitric oxide (NO), which results in the formation of peroxynitrite. Nitric oxide is synthesized by different nitric oxide synthases (NOS). The NO-pathway has been shown to be involved in the pathophysiology of AF, but there is a discrepancy in the literature on the expression of NOS during AF.7–9 In a pig model of AF based on rapid atrial pacing for 1 week, a down-regulation of endothelial NOS (NOS3) expression and a decrease in NO bioavailability was associated with AF.7 These data were confirmed in another pig model of AF, where aortic NOS3 downregulation was observed after 7 h of rapid atrial pacing.8 In contrast, in a dog model of short-term AF, an increase in NOS3 protein expression was observed after 1 week of rapid atrial pacing.9
Data on human atrial tissue samples however do not confirm these findings. In patients with paroxysmal and persistent AF, no change in endocardial NOS3 expression was reported.10,11 However, plasma levels of NO are reduced in AF patients and restored after cardioversion to sinus rhythm.12,13 The pathophysiology of AF in animal models may vary substantially compared with patients with persistent AF. Furthermore, it is noteworthy that the data observed in animal models are mostly short-term models of AF. The observed discrepancy between humans and animal models can therefore be related to the difference in duration of AF and the underlying associated degree of atrial remodelling. We therefore investigated changes in the NO-pathway and oxidative stress in a sheep model of persistent AF. The purpose of the present study was to investigate the impact of persistent AF on atrial myocardial NOS expression and circulating NO levels. Subsequently, we characterized oxidative stress markers and their relation to possible changes in NO bioavailability.
Material and methods
Animal characteristics and instrumentation
Thirty ewes (body weight: 56.6 ± 2.4 kg) were instrumented with an atrial and ventricular pacemaker using a transvenous approach. A neurostimulator (Itrel, Medtronic) for rapid pacing of the atria was implanted subcutaneously in the neck of the sheep. The ventricular pacemaker (Kappa, Medtronic) was inserted at the shoulder. Pacemaker insertion was carried out under general anaesthesia with isoflurane (1%) after pre-medication with ketamine [10 mg/kg intramuscular (IM)] and xylazine (0.2 mg/kg IM). Anaesthesia was induced with 5% isoflurane using a face-mask. The sheep were intubated and ventilated with 100% oxygen. After instrumentation, the sheep recovered for at least 1 week. The atrial pacemaker was then programmed for continuous stimulation at 900 b.p.m., resulting in atrial capture at ∼300 b.p.m. to induce persistent AF. The ventricular pacemaker was programmed in VVI at 40 b.p.m. as back-up. The animals used in this study did not undergo His-bundle ablation. Thirty-six ewes served as controls (CTRL, weight: 50.6 ± 1.5 kg). At regular time intervals, pacing was interrupted to assess the underlying rhythm. The sheep were considered to be in persistent AF if no sinus rhythm could be demonstrated anymore in the follow-up period. At the end of the follow-up period, the sheep were sacrificed under general anaesthesia, as described above, and after administration of an intravenous bolus of heparine (100 U/kg) and fentanyl (5 mg). The absence of deep tendon reflexes was ascertained and the heart was quickly removed through a thoracotomy. In the current study, sheep were sacrificed after a mean of 27.4 ± 1.9 weeks of rapid atrial pacing and were in persistent AF for a mean of 136.5 ± 21.7 days.
The experimental protocol was approved by the ethical committee on animal handling of the University of Leuven. The investigation conforms with the Guide for the Care and Use of Laboratory Animals published by the US National Institutes of Health (NIH Publication No. 85-23, revised 1996).
mRNA expression levels
RNA was isolated from the hearts of 20 AF and 20 CTRL sheep using a commercially available kit Nucleospin RNAII (Macherey-Nagel), reverse transcribed using QuantiTect reverse Transcription kit, and quantified using RiboGreen (Molecular Probes Europe BV). For quantitative analysis, real-time polymerase chain reaction (PCR) was performed using a 7500 fast real-time PCR system (Applied Biosystems). Gene expression of NOS1, NOS2, and NOS3 was studied using Assay-on-demand primer/probe sets (Applied Biosystems). For these genes, probes contained a FAM fluorescent dye at the 5′-end and TAMRA at the 3′-end. Custom-made primers were designed for genes gp91phox, NOX1, p67phox, p22phox, p47phox, p40phox, RAC1, and Xanthine oxidase (XO). Real-time PCR was performed using SYBR®Green dye (Applied Biosystems). The sequences of the forward primers, reverse primers, and TaqMan probes are shown in Table 1.
Gene . | Sequence primers . |
---|---|
NOS1 | Forward: CCCTTGGTGGACCTGAGCTA |
Reverse: GGGTCTCGGAGGCAATCC | |
Probe: CTCCAGGGCGCTGTC | |
NOS2 | Forward: TCACCCATTTCCTGGACATCAC |
Reverse: TGCCTCTCAGCCTCTTCTGT | |
Probe: CCAGCTGCTGCTTCG | |
NOS3 | Forward: GAGCTCTGGAGCCTCATCTG |
Reverse: CACGGAGCTCTGCATCCA | |
Probe: AAACGGCCGCTTCGAC | |
Gp91phox | Forward: GGTGATGTTAGTGGGAGCAGG |
Reverse: TTATCCCAGTTGGGTCGTCC | |
P22phox | Forward: CTGGCGTCCGGTCTAATCCT |
Reverse: CCTCACTCGGCTTCTTGCG | |
NOX1 | Forward: GGAGCAGGAATTGGGGTTA |
Reverse: GGGTGAGCGGTAGCTATTGTA | |
P67phox | Forward: GCAGCAGCCCCAGGAAGAAAC |
Reverse: TCACACCACAGCGTCAGGCAG | |
P47phox | Forward: CCCAACTATGCAGGTGAGCCC |
Reverse: TGTTGCGCCGATAGGTGTCC | |
P40phox | Forward: GCCGAGATGCGAATACCTGC |
Reverse: CCTTGATGGTGCTGATGGTGTC | |
XDH | Forward: CTGGGAAGACTGGGTCATGG |
Reverse: ACCGCCTTGGAGGCATAGA | |
RAC1 | Sense: CATCAAGTGTGTGGTGGTGG |
Antisense: GATGGCGTCAGCTTCTTCTC |
Gene . | Sequence primers . |
---|---|
NOS1 | Forward: CCCTTGGTGGACCTGAGCTA |
Reverse: GGGTCTCGGAGGCAATCC | |
Probe: CTCCAGGGCGCTGTC | |
NOS2 | Forward: TCACCCATTTCCTGGACATCAC |
Reverse: TGCCTCTCAGCCTCTTCTGT | |
Probe: CCAGCTGCTGCTTCG | |
NOS3 | Forward: GAGCTCTGGAGCCTCATCTG |
Reverse: CACGGAGCTCTGCATCCA | |
Probe: AAACGGCCGCTTCGAC | |
Gp91phox | Forward: GGTGATGTTAGTGGGAGCAGG |
Reverse: TTATCCCAGTTGGGTCGTCC | |
P22phox | Forward: CTGGCGTCCGGTCTAATCCT |
Reverse: CCTCACTCGGCTTCTTGCG | |
NOX1 | Forward: GGAGCAGGAATTGGGGTTA |
Reverse: GGGTGAGCGGTAGCTATTGTA | |
P67phox | Forward: GCAGCAGCCCCAGGAAGAAAC |
Reverse: TCACACCACAGCGTCAGGCAG | |
P47phox | Forward: CCCAACTATGCAGGTGAGCCC |
Reverse: TGTTGCGCCGATAGGTGTCC | |
P40phox | Forward: GCCGAGATGCGAATACCTGC |
Reverse: CCTTGATGGTGCTGATGGTGTC | |
XDH | Forward: CTGGGAAGACTGGGTCATGG |
Reverse: ACCGCCTTGGAGGCATAGA | |
RAC1 | Sense: CATCAAGTGTGTGGTGGTGG |
Antisense: GATGGCGTCAGCTTCTTCTC |
Gene . | Sequence primers . |
---|---|
NOS1 | Forward: CCCTTGGTGGACCTGAGCTA |
Reverse: GGGTCTCGGAGGCAATCC | |
Probe: CTCCAGGGCGCTGTC | |
NOS2 | Forward: TCACCCATTTCCTGGACATCAC |
Reverse: TGCCTCTCAGCCTCTTCTGT | |
Probe: CCAGCTGCTGCTTCG | |
NOS3 | Forward: GAGCTCTGGAGCCTCATCTG |
Reverse: CACGGAGCTCTGCATCCA | |
Probe: AAACGGCCGCTTCGAC | |
Gp91phox | Forward: GGTGATGTTAGTGGGAGCAGG |
Reverse: TTATCCCAGTTGGGTCGTCC | |
P22phox | Forward: CTGGCGTCCGGTCTAATCCT |
Reverse: CCTCACTCGGCTTCTTGCG | |
NOX1 | Forward: GGAGCAGGAATTGGGGTTA |
Reverse: GGGTGAGCGGTAGCTATTGTA | |
P67phox | Forward: GCAGCAGCCCCAGGAAGAAAC |
Reverse: TCACACCACAGCGTCAGGCAG | |
P47phox | Forward: CCCAACTATGCAGGTGAGCCC |
Reverse: TGTTGCGCCGATAGGTGTCC | |
P40phox | Forward: GCCGAGATGCGAATACCTGC |
Reverse: CCTTGATGGTGCTGATGGTGTC | |
XDH | Forward: CTGGGAAGACTGGGTCATGG |
Reverse: ACCGCCTTGGAGGCATAGA | |
RAC1 | Sense: CATCAAGTGTGTGGTGGTGG |
Antisense: GATGGCGTCAGCTTCTTCTC |
Gene . | Sequence primers . |
---|---|
NOS1 | Forward: CCCTTGGTGGACCTGAGCTA |
Reverse: GGGTCTCGGAGGCAATCC | |
Probe: CTCCAGGGCGCTGTC | |
NOS2 | Forward: TCACCCATTTCCTGGACATCAC |
Reverse: TGCCTCTCAGCCTCTTCTGT | |
Probe: CCAGCTGCTGCTTCG | |
NOS3 | Forward: GAGCTCTGGAGCCTCATCTG |
Reverse: CACGGAGCTCTGCATCCA | |
Probe: AAACGGCCGCTTCGAC | |
Gp91phox | Forward: GGTGATGTTAGTGGGAGCAGG |
Reverse: TTATCCCAGTTGGGTCGTCC | |
P22phox | Forward: CTGGCGTCCGGTCTAATCCT |
Reverse: CCTCACTCGGCTTCTTGCG | |
NOX1 | Forward: GGAGCAGGAATTGGGGTTA |
Reverse: GGGTGAGCGGTAGCTATTGTA | |
P67phox | Forward: GCAGCAGCCCCAGGAAGAAAC |
Reverse: TCACACCACAGCGTCAGGCAG | |
P47phox | Forward: CCCAACTATGCAGGTGAGCCC |
Reverse: TGTTGCGCCGATAGGTGTCC | |
P40phox | Forward: GCCGAGATGCGAATACCTGC |
Reverse: CCTTGATGGTGCTGATGGTGTC | |
XDH | Forward: CTGGGAAGACTGGGTCATGG |
Reverse: ACCGCCTTGGAGGCATAGA | |
RAC1 | Sense: CATCAAGTGTGTGGTGGTGG |
Antisense: GATGGCGTCAGCTTCTTCTC |
For NOS1 and NOS3, the transcript levels were determined using plasmid standards according to the method described and validated by Peirson et al.14 A dilution series of a plasmid standard was used for each gene to allow comparison of the copy numbers and the results were normalized to glyceraldehyde 3-phosphate dehydrogenase (GAPDH). The remaining genes were analysed using the comparative threshold method. The level of RNA expression was calculated using the threshold cycle (Ct) value,15 normalized with the housekeeping gene GAPDH. Subsequently, ΔΔCt (ΔCt,sample−ΔCt,calibrator) was determined, and the relative expression levels were calculated from 2−ΔΔCt. RNA expression levels are thus indicated as arbitrary units ± SEM. Average values in AF were normalized to the average values in CTRL. All samples were run in duplicate. Measurements were performed on samples obtained from 10 animals in each group.
Immunoblot analysis
After sacrifice, left atrial appendage tissue samples were taken and immediately frozen in liquid nitrogen and stored at −80°C. Atrial tissue was homogenized in a buffer containing (in mM) imidazole 10, sucrose 300, dithiotreitol 1, sodium metabisulfite 1, sodium fluoride 25, sodium ethylene diaminetetraacetic acid (EDTA) 5, sodium pyrophosphate 5, phenylmethylsulfonyl fluoride 0.3, and a protease inhibitor cocktail (‘Complete-Mini’ Roche Molecular Biochemicals). Equal amounts of proteins were separated on 4–12% Bis–Tris gradient gels (NuPage™ electrophoresis system, Invitrogen) and blotted onto polyvinylidene difluoride membranes. Membranes were incubated overnight with antibodies for NOS1 and NOS3 (both 1/500, ABR,), RAC (1/500, Pierce), 3-nitrotyrosine (1/1000, Chemicon), NOX4 (1/500, Pierce), and GAPDH (1/500, Santa Cruz Biotechnology). Detection was performed with alkaline phosphatase-labelled secondary antibodies, using ECF system (Amersham). After scanning the blots, the number of pixels of each positively stained band (antigen–antibody interaction) was calculated. Results were normalized to expression levels of GAPDH and subsequently to protein expression level of the control group.
Assays for activity measurements of superoxide dismutase and glutathione peroxidase and nitrite/nitrate measurement
Left atrial tissue samples were collected at the time of sacrifice. Venous blood samples were taken from the left jugular vein at each follow-up time point. Blood was collected in tubes containing EDTA (1 mg/mL), centrifuged at 4°C and 4000 r.p.m. for 30 min. Plasma was stored at −80°C until the assay. Activity of glutathione peroxidase (GPx), superoxide dismutase (SOD), and nitrite/nitrate levels was analysed using commercially available kits (Cayman Chemical Company). Measurements were performed on samples obtained from 8 CTRL and 11 AF animals.
Histological analysis
Atrial samples from sinus rhythm and AF sheep hearts were fixed in 4% paraformaldehyde for 24 h and routinely embedded in paraffin. After deparaffinization, 7.5 μm thick sections were incubated with the nitrotyrosine antibody (Chemicon) followed by a biotin-labelled secondary antibody. The presence of nitrotyrosine was visualized using the Tyramide signal amplification system (Perkin Elmer, TSA Indirect NEL700001KT). After immunostaining, the sections were counterstained for Harris Haematoxilin and dehydrated in graded series of ethanol. Histological sections were finally embedded in Depex mounting medium. Nitrotyrosine staining was assessed semi-quantitatively in paraffin sections. A score ranging from 0 to 4 points was given to evaluate the level of staining: 0: no staining, 1: weak staining, 2: mild staining, 3: strong staining, and 4: abundant staining. All morphometric analyses were performed using a Zeiss Axiovert 200N microscope with an Axiocam HrC camera and Axiovision 4.6 morphometry software (Carl Zeiss). Two operators blinded for the groups of the animals performed the analysis. Measurements were performed on samples obtained from five animals in each group.
Lucigenin-enhanced luminescence
Superoxide production by atrial tissue homogenates was measured using lucigenin-enhanced chemiluminescence in a microplate luminometer (Victor™ X4 2030 Multi label reader, Perkin Elmer). A low lucigenin concentration (5 μmol/L) was employed to minimize artefactual superoxide production due to redox cycling. Briefly, proteins were diluted in modified Krebs/HEPES buffer and distributed (1 mg/mL) into a 24-well microplate. Nicotine adenine dinucleotide phosphate (NADPH) (100 μM) and dark-adapted lucigenin were added to the wells just before reading. Superoxide production was measured over 20 min. Subsequently, a cell-permeable superoxide scavenger Tiron (20 mM) was added. Superoxide production was defined as the Tiron-inhibitable fraction of the signal expressed in relative light units.16 Measurements were performed on tissue samples obtained from six animals in each group.
Statistics
Data are presented as mean ± SEM. Comparisons between AF and CTRL were performed using Student's t-test for unpaired data, where normal distribution of the data could be assumed (Figures 1A–C, E, F, 2D, 3), or a non-parametrical Mann–Whitney U test, when the data were not normally distributed (Figures 1D, 2C). A value of P < 0.05 was considered statistically significant.
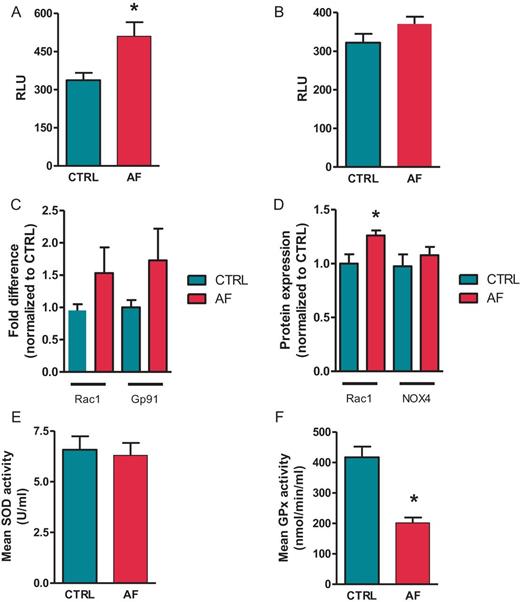
Disrupted oxidant and anti-oxidant properties during AF. (A) NADPH oxidase stimulated and (B) basal superoxide production in atrial tissue homogenates from CTRL and AF. Results are expressed as relative luminescent units (RLU). (N = 6 in both groups). (C) mRNA expression level of NADPH oxidase activator Rac1 and the subunit Gp91 of the NADPH oxidase complex in atrial tissue of CTRL and AF (N = 20 in both groups). Data are normalized to expression levels of CTRL. (D) Protein expression of Rac1 and NOX4 in atrial tissue homogenates in CTRL and AF (CTRL N = 6, AF N = 5). Data are normalized to expression levels of CTRL. (E) Activity of SOD and (F) GPx in atrial tissue homogenates. Data are expressed as units/mL and nmol/mL homogenate, respectively (CTRL N = 8, AF N = 11). *P < 0.05.

3-Nitrotyrosine accumulation during AF. Example of immunohistochemical staining for 3-nitrotyrosine in atrial tissue of CTRL (A) and AF (B). (C) Semi-quantitative analysis of immunohistochemical staining for 3-nitrotyrosine (N = 5 in both groups). (D) Dot blot analysis of atrial tissue samples of CTRL and AF (CTRL N = 11, AF N = 16). *P < 0.05.
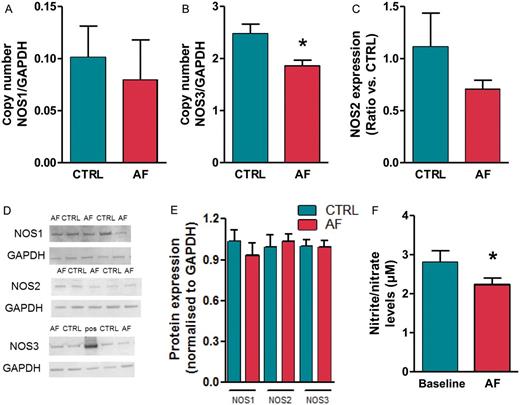
Decreased NOS expression in atrial tissue of AF. mRNA expression level of NOS1 (A), NOS3 (B), and NOS2 (C) in atrial tissue of CTRL and AF (N = 10 in both groups). Data are normalized to expression levels of GAPDH. (D) Immunoblot and (E) mean signal density for NOS1, NOS2, and NOS3, normalized to expression levels GAPDH, in CTRL (N = 16) and AF (N = 15) samples, including a positive control for NOS3 (‘pos’ in the example shown, tissue homogenate from a NOS3 overexpression mouse29). (F) Nitrite/nitrate levels in venous plasma samples of CTRL and AF (CTRL N = 8, AF N = 11). *P < 0.05.
Results
Atrial superoxide production in control and atrial fibrillation
In atrial cells, NADPH oxidase is the major source of superoxide production. NADPH-stimulated superoxide production was significantly higher in left atrium (LA) from sheep with AF compared with controls (+51.3 ± 23.2%, Figure 1A, P < 0.01). Basal superoxide production has tended to be higher in the AF group (+15 ± 7.7%, Figure 1B, P = 0.07). mRNA expression levels of different NADPH subunits were measured using quantitative PCR (qPCR). There was a tendency of increased levels of gp91phox in the AF group (Figure 1C, P = 0.06). Expression of other subunits p22phox, p67phox, and p47phox were unchanged compared with the control group (data not shown).
Since activity of NADPH oxidase is regulated by Rac1, we investigated changes in expression levels of Rac1 during AF. A clear trend to increased mRNA expression was seen (Figure 1C, P = 0.06) and at protein level the increase in expression levels of Rac1 in the AF group was significant (+26 ± 4.6%, Figure 1D, P < 0.05). A trend towards higher NOX4 expression was observed in AF samples (+15 ± 5.2%, Figure 1D, P = 0.08). Xanthine oxidase is another source of superoxide production. No difference was seen in mRNA expression levels of XO during AF (data not shown).
To neutralize the effect of harmful excessive superoxide production, nature has developed an anti-oxidant enzymatic defence, including SOD and GPx. Therefore, we determined whether the atrial anti-oxidant capacity was also affected during AF. Measurements of total SOD activity in atrial tissue samples appeared to be unchanged compared with controls (Figure 1E). A decrease in the activity of GPx was observed during AF (Figure 1F, P < 0.05).
Atrial tissue 3-nitrotyrosine content during persistent atrial fibrillation
An example of an immunohistochemical staining of left atrial appendages for 3-nitrotyrosine in CTRL and AF is shown in Figure 2A and B, respectively. Semi-quantitative analysis revealed a significant increase in 3-nitrotyrosine content in the AF group compared with controls (P < 0.05, Figure 2C). 3-Nitrotyrosine was identified as an abundant cytoplasmic deposition in AF myocytes. This was contrary to control myocytes in which 3-nitrotyrosine was either absent or present as weak cytoplasmic staining. Dot blot analysis confirmed the increase in 3-nitrotyrosine content in the AF group (Figure 2D).
Effect of atrial fibrillation on endocardial nitric oxide synthase expression and nitrite/nitrate levels
Endocardial NOS expression from AF and control animals was quantified by mRNA and protein expression analysis. Different NOS isoforms were expressed in atrial tissue. NOS3 mRNA expression in the AF group was 24.9 ± 4.4% less than in control animals (Figure 3B, P < 0.05). mRNA expressions of NOS1 and 2 were unchanged between groups (Figure 3A and C, respectively). NOS3 protein expression was however not significantly changed with AF (Figure 3D, E). Protein expression of NOS1 and NOS2 was also not different between groups (Figure 3D, E). Expression levels were normalized to expression levels of GAPDH.
Plasma levels of nitrite/nitrate in animals with AF, measured after 18 weeks of pacing, were significantly lower than animals in sinus rhythm. During AF, nitrite/nitrate levels diminished by 24.8 ± 5.8% (Figure 3F).
Discussion
In the present study we demonstrate that in a sheep model of persistent AF, AF increases superoxide production, possibly resulting from increased NAPDH-oxidase activity. Atrial fibrillation attenuates NOS3 transcript levels and is associated with decreased circulating nitrite/nitrate levels. This reduction is associated with a significant accumulation of peroxynitrite. These findings affirm that oxidative stress and NO-related changes play a role in atrial remodelling underlying AF.
Atrial superoxide production and oxidative stress during atrial fibrillation
The decreased levels of circulating nitrite/nitrate suggest a decrease in bioavailability of NOx. This could be the result of increased scavenging by excessive superoxide anions production, which is present during oxidative stress. The resulting peroxynitrite formation has the capacity to cause nitration of tyrosine residues of proteins, leading to the formation of 3-nitrotyrosine. Previously, an important role for peroxynitrite in atrial remodelling during atrial tachycardia has been suggested.5 Our data confirm an accumulation of peroxynitrite in atrial tissue samples of sheep with persistent AF, as described in humans.5
Emerging evidence indicates that the main source of superoxide production in atrial myocytes is NADPH oxidase.17 NADPH-dependent superoxide production is increased in samples of right atrium (RA) of patients with AF. Moreover, NADPH-stimulated superoxide production in RA of patients in AF is independently associated with an increased risk of post-operative AF. In a pig model of atrial tachycardia, basal superoxide production was unchanged in RA, but increased in tissue samples of the LA.18 A very recent study demonstrated that the myocardial NO-redox imbalance can differ between the RA and LA with the underlying degree of atrial remodelling.11 We investigated in our sheep model of persistent AF if the accumulation of peroxynitrite could be ascribed to increased superoxide production in LA, and if so, from which source it originates. Basal superoxide in our animal model tended to be higher. As in humans, increased NADPH oxidase activity contributes to the excessive superoxide production seen during persistent AF. Our data suggest that the underlying mechanism might be mediated via increased enzyme activation. NADPH oxidase consists of several proteins, including the membrane-bound catalytic unit, composed of Nox and p22phox subunits, and multiple cytoplasmic accessory subunits (p47phox, p40phox, and p67phox). Proper assembly of these subunits is essential in forming the active NADPH oxidase complex.19 Because mRNA expression of the different subunits was unchanged during AF in sheep, in accordance with recent data,11 we investigated the expression of Rac1, an important regulator of assembly of the different subunits, to elucidate the mechanism for increased activity. The amount of Rac1 has been shown to correlate with the production of superoxide via the active NADPH oxidase complex.20 A significant 26% increase in Rac1 protein expression was seen in AF.
Interestingly, angiotensin II, via activation of angiotensin receptor type 1, is a well-known stimulator of NADPH oxidase activity.19 We previously demonstrated that in our sheep model of AF, levels of angiotensin II were significantly elevated.21,22 Patients receiving treatment with inhibitors of the angiotensin–renin system were proven to have a lower incidence of AF following atrial flutter ablation.23 These data imply that angiotensin-induced oxidative stress could also play a significant role in the atrial remodelling processes underlying persistent AF.
NOS3 expression in persistent atrial fibrillation
So far, there is some controversy about the role of NOS3 during atrial remodelling underlying AF. In a pig model of AF, induced by 2 weeks of rapid atrial pacing, it was demonstrated that NOS3 protein expression was profoundly attenuated and the availability of NO decreased.7 In contrast to these findings, atrial tachycardia for 8 days in dogs resulted in increased NOS3 protein expression.9 In contrast, data on NOS3 protein expression in patients with AF did not reveal any differences between groups.10,11 Our findings of decreased NOS3 expression during AF were observed at mRNA level, but were absent on protein level, as detected by western blot analysis, possibly due to longer half-life of NOS3 proteins compared with transcripts. Possible explanations for the discrepancy in expression levels between animal models and humans can be related to the presence of concomitant diseases, as well as dynamic expression patterns over time. The latter would imply that atrial tachycardia induces a decline in NOS expression during short-term follow-up, which normalizes during extended follow-up. This may be interesting in the setting of AF, since induction of persistent AF is a slow, progressive process with different levels of remodelling occurring at different moments in time.
Of note, our findings cannot exclude a change in NOS activity. NOS3 activity can be modulated via different pathways, including NOS ‘uncoupling’, phosphorylation, as well as via activation by Ca-calmodulin. Recent data however suggest unchanged phosphorylation status of NOS3.11 When deprived of the critical cofactor tetrahydrobiopterin or of the substrate l-arginine, NOS can generate superoxide (a process referred to as NOS uncoupling).24 It has been described that NOS uncoupling can contribute significantly to the increased superoxide production seen during AF and heart failure-induced atrial remodelling.11,17,25 Furthermore, in cardiomyocytes NOS3 activity is regulated by free cytosolic calcium concentrations, which bind to calmodulin and thereby stimulate NOS3 activation. Hence, a decrease in intracellular calcium concentrations reduces NOS3 activity.26,27 We previously described in our sheep model that persistent AF induces significant changes in intracellular calcium concentrations, resulting in decreased calcium influx in atrial myocytes.28
Conclusions
In summary, data from the present study demonstrate that in a sheep model of persistent AF, AF attenuates atrial NOS3 transcript levels and is associated with decreased circulating NOx levels. Persistent AF is associated with increased oxidative stress, probably resulting from increased NADPH oxidase activity, without major changes in anti-oxidant capacity of the atrial tissue. These findings suggest that oxidative stress and NO-related changes play a role in the atrial remodelling processes underlying AF.
Acknowledgements
This work was supported by the Research Foundation Flanders (FWO-Vlaanderen) of which I.L. and R.B.D. are postdoctoral fellows.
References
- antioxidants
- atrial fibrillation
- nicotine
- nitrate
- nitric oxide
- oxidative stress
- immunohistochemistry
- atrium
- adenine
- dinucleoside phosphates
- glutathione peroxidase
- immunoblotting
- nadp
- nadph oxidases
- nitrites
- phosphates
- rna, messenger
- sheep
- superoxides
- superoxide dismutase
- peroxynitrite
- oxidase
- atrial-based pacing
- 3-nitrotyrosine
- persistent atrial fibrillation