-
PDF
- Split View
-
Views
-
Cite
Cite
Louisa C Malcolme-Lawes, Phang Boon Lim, Michael Koa-Wing, Zachary I Whinnett, Shahnaz Jamil-Copley, Sajad Hayat, Darrel P Francis, Pipin Kojodjojo, D Wyn Davies, Nicholas S Peters, Prapa Kanagaratnam, Robotic assistance and general anaesthesia improve catheter stability and increase signal attenuation during atrial fibrillation ablation, EP Europace, Volume 15, Issue 1, January 2013, Pages 41–47, https://doi.org/10.1093/europace/eus244
- Share Icon Share
Abstract
Recurrent arrhythmias after ablation procedures are often caused by recovery of ablated tissue. Robotic catheter manipulation systems increase catheter tip stability which improves energy delivery and could produce more transmural lesions. We tested this assertion using bipolar voltage attenuation as a marker of lesion quality comparing robotic and manual circumferential pulmonary vein ablation for atrial fibrillation (AF).
Twenty patients were randomly assigned to robotic or manual AF ablation at standard radiofrequency (RF) settings for our institution (30 W 60 s manual, 25 W 30 s robotic, R30). A separate group of 10 consecutive patients underwent robotic ablation at increased RF duration, 25 W for 60 s (R60). Lesions were marked on an electroanatomic map before and after ablation to measure distance moved and change in bipolar electrogram amplitude during RF. A total of 1108 lesions were studied (761 robotic, 347 manual). A correlation was identified between voltage attenuation and catheter movement during RF (Spearman's rho −0.929, P < 0.001). The ablation catheter was more stable during robotic RF; 2.9 ± 2.3 mm (R30) and 2.6 ± 2.2 mm (R60), both significantly less than the manual group (4.3 ± 3.0 mm, P < 0.001). Despite improved stability, there was no difference in signal attenuation between the manual and R30 group. However, there was increased signal attenuation in the R60 group (52.4 ± 19.4%) compared with manual (47.7 ± 25.4%, P = 0.01). When procedures under general anaesthesia (GA) and conscious sedation were analysed separately, the improvement in signal attenuation in the R60 group was only significant in the procedures under GA.
Robotically assisted ablation has the capability to deliver greater bipolar voltage attenuation compared with manual ablation with appropriate selection of RF parameters. General anaesthesia confers additional benefits of catheter stability and greater signal attenuation. These findings may have a significant impact on outcomes from AF ablation procedures.
Our study has demonstrated the following findings:
1. Robotically assisted ablation confers additional catheter stability over manual ablation
2. Greater catheter stability during ablation is associated with higher local electrogram attenuation in both robotic and manual modalities.
3. Robotically assisted ablation performed with both reduced power and duration of RF energy (adopted by many users do to potential safety concerns) produces similar electrogram attenuation to manual ablation.
4. Robotically assisted ablation performed at the same RF duration as manual ablation (while maintaining a reduction in power) produces greater signal attenuation compared with manual ablation.
5. Procedures performed under general anaesthesia achieved improved parameters of catheter stability and signal attenuation compared with those under conscious sedation.
Introduction
Achieving permanent pulmonary vein isolation with a single procedure is an ongoing challenge of atrial fibrillation (AF) ablation.1–4 Conventional ablation catheters were designed to deliver radiofrequency (RF) energy to discrete sites such as the slow pathway to treat re-entrant arrhythmias with very high success rates. Catheter ablation for AF requires an extensive lesion set and has yet to achieve equivalent success rates, which may in part be due to the limitations of such catheters.5,6 Robotic catheter navigation has been developed to overcome some of the limitations of manual catheters such as precision movements for placing contigious lesions and stable catheter position to maintain tissue contact throughout ablation delivery.7–9 In animal studies, we demonstrated that at equivalent RF settings, there was more rapid and greater reduction in local electrogram amplitude during robotic ablation compared with manual. This was also associated with more consistent transmurality on macroscopic examination of robotic lesions.10 However, this has not improved success rates for robotic ablation of AF in several non-randomized clinical studies compared with conventional manual approach.11–13 A possible explanation is that many users reduced the robotic RF power and duration compared with manual settings due to concern about increased catheter stability leading to excessive energy delivery increasing the risk of perforation and oesophageal injury.14,15
In order to understand the discrepancy between the animal and human work, we investigated whether selection of the energy level or limitations of robotic manoeuvrability in the left atrium affect the lesion quality of robotic ablation in humans.
Methods
Study patients
Patients with symptomatic paroxysmal AF, resistant to at least one anti-arrhythmic agent, and referred for AF ablation were screened. All patients stopped their anti-arrhythmic agent five half-lives prior to the procedure and only those in sinus rhythm on the day of the procedure were recruited. All patients provided written informed consent for participation in the study, which was approved by the Local Research Ethics Committee. The 20 study patients were randomized to receive either robotic or manual ablation at our institution's current standard RF settings of 30 W for 60 s for manual ablation and 25 W for 30 s for robotic ablation. In a subsequent non-randomized consecutive group of 10 patients, all undergoing robotic ablation, the duration of RF energy delivery was increased to 60 as for manual ablation, while maintaining the same reduced power setting of 25 W. All procedures were performed by experienced operators.
The Hansen Sensei system
The Sensei system has been described in detail previously.7,16 In brief, the system comprises three linked components: the physician's workstation (Sensei™ robotic control system), remote catheter manipulator and steerable guide catheter (Artisan™ Sheath). The steerable guide catheter comprises an outer (14F) and inner (10.5F) steerable sheath through which any 8.5F or less ablation catheter can be placed. The Artisan sheath maintains the catheter position by the tensile strength of four pullwires so that the shape adopted by the sheath is uniquely suited to the point of interest to which the catheter is being positioned.7 This is in contrast to the manual approach, where the operator has to dynamically apply torque and flexion to prevent the catheter displacing from the point of interest. The Sensei™ robotic control system also incorporates a pressure sensor (Intellisense™), which calculates the contact force at the tip of the catheter. This enhances the validation of tissue contact for optimal lesion production and helps prevent the use of excessive force.7
Ablation procedure
The procedure was performed in the fasted state, following trans-oesophageal echocardiogram to confirm the left atrial appendage was free of thrombus. Procedures were either performed under conscious sedation with morphine and midazolam or under general anaesthetic, according to the operator's preference. Arterial pressure monitoring was used in all patients. Two 8F sheaths were inserted into the right femoral vein and a 7F sheath inserted into the left femoral vein. For robotic procedures a long 14F sheath (30 cm) was inserted into the left femoral vein and advanced to the inferior vena cava (IVC) under fluoroscopic guidance. Following a single transeptal puncture, unfractionated heparin was given to maintain therapeutic activated clotting time (between 300 and 350 s). Pulmonary venography was performed. An irrigated, 3.5 mm tip bipolar mapping catheter (Navistar™, Biosense Webster, CA, USA) was used. In manual procedures, a steerable sheath was used for all cases. An electro-anatomic map of the left atrium was collected using CARTO-XP™ (Biosense Webster). Circumferential pulmonary vein ablation (CPVA) was performed guided by 3D mapping and the ablation catheter was kept still at each ablation point. Radiofrequency energy delivered at each site at 30 W for 60 s in manual ablation and 25 W for 30 s for robotic ablation. For robotic procedures using Intellisense, lesions were delivered using 10–20 g pressure.
Catheter stability measurement
An ablation lesion marker was placed on the electroanatomic map at the start and finish of RF delivery. The distance between markers was calculated for each RF delivery using the CARTO distance measurement tool. We have previously validated the accuracy of this tool.17 Stability was compared between two time points and therefore catheter excursion in between these time-points will not be registered by our method. Operators were instructed to maintain the ablation catheter at the same position and lesions with complete catheter displacement (>1 cm) were excluded. To validate our methodology, we compared distance moved during energy delivery with the corresponding signal attenuation for all lesions, before proceeding to analyse each group individually.
Signal reduction measurement
The measurement of local electrogram voltage reduction has been used in several studies to monitor tissue injury during RF energy delivery10,18–20 and as an endpoint for during circumferential pulmonary vein ablation in humans.21 The intracardiac electrogram recordings were stored on a Bard system (Lab system Pro EP, Bard, Lowell, MA) for offline measurement. The bipolar electrogram recording from the distal electrode of the mapping catheter was measured from peak maximum to peak minimum. Signal amplitude is known to change with respiration and cardiac motion; therefore, averaging the signal amplitude over five cardiac cycles may provide improved accuracy over a single measurement. The measurements were taken from the five atrial electrograms immediately prior to ablation start and immediately following ablation stop (corresponding time points are automatically logged on the Bard system). The percentage voltage change between the mean pre- and mean post-ablation electrogram was calculated for each lesion and compared between robotic and manual modalities. Lesions which demonstrated an increase in voltage were defined as exhibiting catheter micromovement since an increase in voltage can only be due to movement of the catheter and not due to RF delivery. The number of lesions demonstrating catheter micromovement during ablation were also compared between robotic and manual modalities, and excluded from the overall calculation of voltage reduction for each modality.
Evaluation of measurement reliability
To prevent bias that may arise through unblinded signal measurement, we assessed inter-observer variability in determination of voltages using the data from two patients from each of the three groups. The two additional observers were blinded to the findings of the first observer and each other, and to the modality of the procedure. Distance measurements were calculated automatically by the electroanatomic mapping system; therefore, reproducibility testing was not felt to be necessary.
Comparison of ablation parameters for left- and right-sided circumferential ablation
Due to the relative inflexibility of the robotic inner and outer steerable sheaths, difficulty in reaching and maintaining stability at the right lower pulmonary vein has been noted by some operators. We compared signal attenuation during ablation for right- and left-sided veins in both robotic and manual groups to investigate whether there was a difference in ablation efficacy between left- and right-sided ablation.
Statistics
All variables are expressed as mean ± standard deviation. Statistical analysis of continuous variables was by unpaired t-tests. Analysis of categorical variables was by χ2 test. The association between distance moved during ablation and voltage reduction was analysed using Spearman's rank correlation. Inter-observer, signal amplitude measurement reproducibility was determined using Pearson's correlation co-efficient. A P value of <0.05 was considered statistically significant.
Results
Patients were randomized to either manual (n = 10) or robotic ablation (n = 10). A further 10 consecutive patients underwent robotic ablation at the increased lesion duration of 60 s. The patient demographics and procedural details are shown in Table 1. No significant differences in patient demographics or procedural details were noted between groups, with the exception of total RF time which was greater in the R60 group. There were three complications; two groin haematomas (minor) requiring no intervention, one in the manual group, one in the R60 group, and a retroperitoneal bleed (major) in the R30 group, given two units of blood transfusion and settled with no further treatment. These were not on the same side as the robotic 14F sheath, but they were all on the same side as the arterial sheath. Of note, there were no significant differences in patient demographics or procedural outcomes between procedures under general anaesthesia and conscious sedation.
Characteristic . | Manual (M) . | Robotic 30 (R30) . | Robotic 60 (R60) . | M vs. R30, P value . | M vs. R60, P value . |
---|---|---|---|---|---|
Patient | |||||
Age (years) | 64.6 ± 10.6 | 59.3 ± 9.7 | 60.6 8.3 | 0.76 | 0.89 |
AF duration (months) | 41.8 ± 34.6 | 39.4 ± 29.9 | 59.9 ± 53.8 | 0.86 | 0.18 |
No. of failed AADs | 1.4 ± 0.8 | 1.5 ± 0.7 | 1.33 ± 0.5 | 0.92 | 0.98 |
CAD (%) | 11.1 | 9.1 | 11.1 | 1.0 | 1.0 |
Hypertension (%) | 55.6 | 27.3 | 33.3 | 0.14 | 0.14 |
Diabetes (%) | 22.2 | 0.0 | 11.1 | 0.48 | 0.48 |
LVEF (%) | 52.0 ± 7.5 | 56.2 ± 6.4 | 56.4 ± 7.5 | 0.20 | 0.25 |
LA size (mm) | 38.0 ± 5.8 | 42.7 ± 7.9 | 42.5 ± 6.6 | 0.14 | 0.17 |
Procedural | |||||
Procedure time (min) | 224:48 ± 55:49 | 222:13 ± 26:14 | 233:17 ± 30:21 | 0.92 | 0.73 |
Fluoroscopy time (min) | 55:29 ± 18:24 | 48:25 ± 9:34 | 46:06 ± 8:19 | 0.65 | 0.72 |
General anaesthesia vs. sedation | 5/5 | 6/4 | 7/3 | 0.79 | 0.48 |
No. of lesions in CPVA | 48.7 | 60.2 | 57.0 | 0.13 | 0.19 |
Additional RF to achieve PVI (min) | 17:06 ± 10:33 | 16:30 ± 10:16 | 17:53 ± 10:14 | 0.93 | 0.94 |
Total RF time (min) | 56:05 ± 11:39 | 46:13 ± 15:02 | 79:22 ± 12:44 | 0.19 | *0.003 |
Major complications | 0 | 1 | 0 | 0.32 | 1.0 |
Minor complication | 1 | 0 | 1 | 0.32 | 1.0 |
Freedom from AF (%) | 60 | 50 | 80 | 0.66 | 0.34 |
Characteristic . | Manual (M) . | Robotic 30 (R30) . | Robotic 60 (R60) . | M vs. R30, P value . | M vs. R60, P value . |
---|---|---|---|---|---|
Patient | |||||
Age (years) | 64.6 ± 10.6 | 59.3 ± 9.7 | 60.6 8.3 | 0.76 | 0.89 |
AF duration (months) | 41.8 ± 34.6 | 39.4 ± 29.9 | 59.9 ± 53.8 | 0.86 | 0.18 |
No. of failed AADs | 1.4 ± 0.8 | 1.5 ± 0.7 | 1.33 ± 0.5 | 0.92 | 0.98 |
CAD (%) | 11.1 | 9.1 | 11.1 | 1.0 | 1.0 |
Hypertension (%) | 55.6 | 27.3 | 33.3 | 0.14 | 0.14 |
Diabetes (%) | 22.2 | 0.0 | 11.1 | 0.48 | 0.48 |
LVEF (%) | 52.0 ± 7.5 | 56.2 ± 6.4 | 56.4 ± 7.5 | 0.20 | 0.25 |
LA size (mm) | 38.0 ± 5.8 | 42.7 ± 7.9 | 42.5 ± 6.6 | 0.14 | 0.17 |
Procedural | |||||
Procedure time (min) | 224:48 ± 55:49 | 222:13 ± 26:14 | 233:17 ± 30:21 | 0.92 | 0.73 |
Fluoroscopy time (min) | 55:29 ± 18:24 | 48:25 ± 9:34 | 46:06 ± 8:19 | 0.65 | 0.72 |
General anaesthesia vs. sedation | 5/5 | 6/4 | 7/3 | 0.79 | 0.48 |
No. of lesions in CPVA | 48.7 | 60.2 | 57.0 | 0.13 | 0.19 |
Additional RF to achieve PVI (min) | 17:06 ± 10:33 | 16:30 ± 10:16 | 17:53 ± 10:14 | 0.93 | 0.94 |
Total RF time (min) | 56:05 ± 11:39 | 46:13 ± 15:02 | 79:22 ± 12:44 | 0.19 | *0.003 |
Major complications | 0 | 1 | 0 | 0.32 | 1.0 |
Minor complication | 1 | 0 | 1 | 0.32 | 1.0 |
Freedom from AF (%) | 60 | 50 | 80 | 0.66 | 0.34 |
*P values comparing between groups using T-tests for normally distributed variables and χ2 tests for binary variables.
Characteristic . | Manual (M) . | Robotic 30 (R30) . | Robotic 60 (R60) . | M vs. R30, P value . | M vs. R60, P value . |
---|---|---|---|---|---|
Patient | |||||
Age (years) | 64.6 ± 10.6 | 59.3 ± 9.7 | 60.6 8.3 | 0.76 | 0.89 |
AF duration (months) | 41.8 ± 34.6 | 39.4 ± 29.9 | 59.9 ± 53.8 | 0.86 | 0.18 |
No. of failed AADs | 1.4 ± 0.8 | 1.5 ± 0.7 | 1.33 ± 0.5 | 0.92 | 0.98 |
CAD (%) | 11.1 | 9.1 | 11.1 | 1.0 | 1.0 |
Hypertension (%) | 55.6 | 27.3 | 33.3 | 0.14 | 0.14 |
Diabetes (%) | 22.2 | 0.0 | 11.1 | 0.48 | 0.48 |
LVEF (%) | 52.0 ± 7.5 | 56.2 ± 6.4 | 56.4 ± 7.5 | 0.20 | 0.25 |
LA size (mm) | 38.0 ± 5.8 | 42.7 ± 7.9 | 42.5 ± 6.6 | 0.14 | 0.17 |
Procedural | |||||
Procedure time (min) | 224:48 ± 55:49 | 222:13 ± 26:14 | 233:17 ± 30:21 | 0.92 | 0.73 |
Fluoroscopy time (min) | 55:29 ± 18:24 | 48:25 ± 9:34 | 46:06 ± 8:19 | 0.65 | 0.72 |
General anaesthesia vs. sedation | 5/5 | 6/4 | 7/3 | 0.79 | 0.48 |
No. of lesions in CPVA | 48.7 | 60.2 | 57.0 | 0.13 | 0.19 |
Additional RF to achieve PVI (min) | 17:06 ± 10:33 | 16:30 ± 10:16 | 17:53 ± 10:14 | 0.93 | 0.94 |
Total RF time (min) | 56:05 ± 11:39 | 46:13 ± 15:02 | 79:22 ± 12:44 | 0.19 | *0.003 |
Major complications | 0 | 1 | 0 | 0.32 | 1.0 |
Minor complication | 1 | 0 | 1 | 0.32 | 1.0 |
Freedom from AF (%) | 60 | 50 | 80 | 0.66 | 0.34 |
Characteristic . | Manual (M) . | Robotic 30 (R30) . | Robotic 60 (R60) . | M vs. R30, P value . | M vs. R60, P value . |
---|---|---|---|---|---|
Patient | |||||
Age (years) | 64.6 ± 10.6 | 59.3 ± 9.7 | 60.6 8.3 | 0.76 | 0.89 |
AF duration (months) | 41.8 ± 34.6 | 39.4 ± 29.9 | 59.9 ± 53.8 | 0.86 | 0.18 |
No. of failed AADs | 1.4 ± 0.8 | 1.5 ± 0.7 | 1.33 ± 0.5 | 0.92 | 0.98 |
CAD (%) | 11.1 | 9.1 | 11.1 | 1.0 | 1.0 |
Hypertension (%) | 55.6 | 27.3 | 33.3 | 0.14 | 0.14 |
Diabetes (%) | 22.2 | 0.0 | 11.1 | 0.48 | 0.48 |
LVEF (%) | 52.0 ± 7.5 | 56.2 ± 6.4 | 56.4 ± 7.5 | 0.20 | 0.25 |
LA size (mm) | 38.0 ± 5.8 | 42.7 ± 7.9 | 42.5 ± 6.6 | 0.14 | 0.17 |
Procedural | |||||
Procedure time (min) | 224:48 ± 55:49 | 222:13 ± 26:14 | 233:17 ± 30:21 | 0.92 | 0.73 |
Fluoroscopy time (min) | 55:29 ± 18:24 | 48:25 ± 9:34 | 46:06 ± 8:19 | 0.65 | 0.72 |
General anaesthesia vs. sedation | 5/5 | 6/4 | 7/3 | 0.79 | 0.48 |
No. of lesions in CPVA | 48.7 | 60.2 | 57.0 | 0.13 | 0.19 |
Additional RF to achieve PVI (min) | 17:06 ± 10:33 | 16:30 ± 10:16 | 17:53 ± 10:14 | 0.93 | 0.94 |
Total RF time (min) | 56:05 ± 11:39 | 46:13 ± 15:02 | 79:22 ± 12:44 | 0.19 | *0.003 |
Major complications | 0 | 1 | 0 | 0.32 | 1.0 |
Minor complication | 1 | 0 | 1 | 0.32 | 1.0 |
Freedom from AF (%) | 60 | 50 | 80 | 0.66 | 0.34 |
*P values comparing between groups using T-tests for normally distributed variables and χ2 tests for binary variables.
Methodology validation
Voltage measurements were compared in six patients between the original observer (1) and two further observers (2 and 3). A good correlation was demonstrated between ablation start and stop measurements for observer 1 vs. 2 (R2= 0.862 and 0.799, respectively, P < 0.001) and for observer 1 and 3 (R2= 0.87 and 0.856, respectively, P < 0.001). The voltage measurements at ablation start and ablation stop were not significantly different between observer 1 and 2 (P = 0.97 and 0.79, respectively) or observer 1 and 3 (P = 0.41 and 0.39, respectively). This confirmed that our method was accurate, reproducible, and unbiased.
The importance of catheter stability for effective lesion formation is well established. We measured catheter stability using two time points at the beginning and end of energy delivery. In order to confirm that this technique produces a valid marker of catheter stability, distance moved during RF was correlated with local electrogram reduction for all lesions (Figure 1). A significant negative correlation was identified (Spearman's rho −0.929, P < 0.001). Voltage reduction for stable deliveries (<1 mm moved during RF) was 46.1 ± 30.8% compared with 32.6 ± 32.7% unstable deliveries (>1 mm movement) (P = 0.01). This would suggest that our technique for assessing lesions in vivo is related to catheter stability.
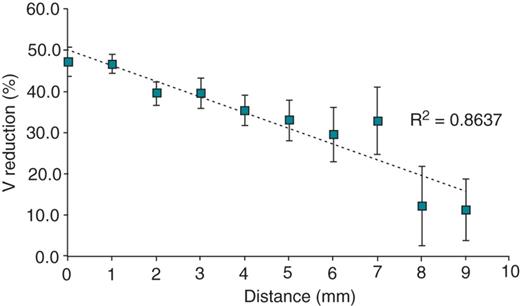
Signal amplitude reduction (mean ± SEM) produced with increasing distance moved during radiofrequency ablation including both robotic and manual ablation modalities, demonstrating that greater catheter stability is associated with greater signal amplitude reduction following ablation.
Lesions which produced an increase in local electrogram voltage were defined as episodes of catheter micromovement. We confirmed this by comparing the average distance moved for lesions demonstrating micromovement with those not demonstrating micromovement (4.5 ± 3.5 vs. 3.1 ± 2.6 mm respectively, P < 0.001).
Catheter stability
Catheter stability was analysed on each completed lesion: manual (M, n = 347), robotic 30 s (R30, n = 406), and robotic 60 s (R60, n = 355). The average distance moved during RF delivery (Figure 2 top panel) was 4.3 ± 3.0 mm (M) vs. 2.9 ± 2.3 mm (R30) vs. 2.6 ± 2.2 mm (R60) and both robotic groups were more stable than manual ablation (P < 0.001).
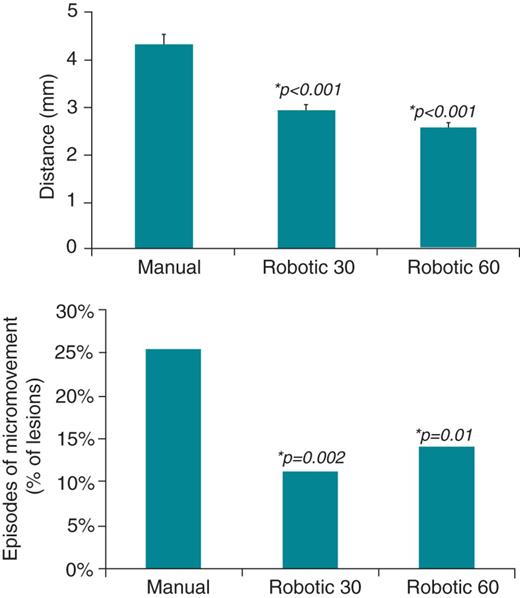
(Top panel) Distance (mean ± SEM) moved by catheter tip measured during robotic and manual radiofrequency ablation, demonstrating greater catheter stability with robotic ablation. (Bottom panel) Episodes of micromovement (defined as an increase in local electrogram voltage during ablation) compared between robotic and manual ablation modalities. *P values comparing each of the robotic groups with manual using Student's T-tests.
The episodes of micromovement were 88 out of 347 (25%) in the manual group, 46 out of 406 (11%) in robotic 30 s, and 50 out of 355 (14%) in the robotic 60 s groups (Figure 2 bottom panel). Again both robotic groups were more stable than manual (P = 0.002 and P = 0.01, for R30 and R60, respectively).
The assertion that robotic catheter positioning is more stable than manual appears to be correct during CPVA in the left atrium and the duration of the RF delivery does not appear to influence this.
Signal amplitude reduction
The average voltage reduction was no different in the manual group 47.7 ± 25.4% compared with the R30 group 45.9 ± 21.3%, P = 0.38. However, in the patients who underwent robotic ablation with increased RF duration (R60), there was a statistically greater voltage reduction 52.4 ± 19.4% compared with manual ablation (P = 0.012) and compared with R30 (P < 0.001) (Figure 3).
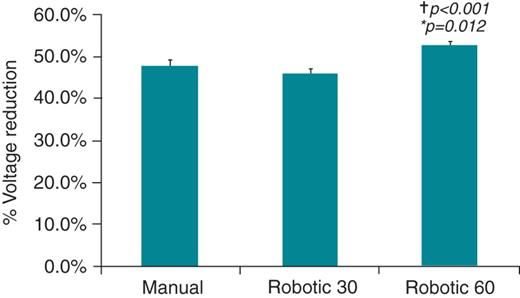
Significantly greater signal amplitude reduction (mean ± SEM) with robotic ablation for 60 s compared with manual (60 s) and robotic (30 s) ablation at standard settings. *P values comparing between manual and robotic 60 s group using Student's T-tests. †P values comparing between robotic 30 s and robotic 60 s groups using Student's T-tests.
This confirms that increased catheter stability conferred by robotic navigation leads to greater signal attenuation compared with manual ablation. If both power and duration of RF energy are reduced (R30) then the benefits of robotic navigation can be lost.
The effect of general anaesthesia on catheter stability
It has been suggested that the use of general anaesthesia can improve procedural outcomes and lead to lower rates of pulmonary vein reconnection.22 We studied the effect of GA on all procedures. Measures of catheter stability were better in procedures under GA compared with conscious sedation (distance moved; 2.8 ± 2.3 vs. 4.1 ± 3.3 mm, P < 0.001 and episodes of micromovement; 12 vs. 18%, respectively, P < 0.001) as were markers of ablation efficacy (signal attenuation 50.5 ± 21.8 vs. 46.8 ± 22.4%, respectively, P = 0.03).
When procedures under GA were compared with procedures under conscious sedation in R60, R30, and manual the differences did not reach statistical significance (Figure 4). Patient numbers may have been too small to identify this difference. When procedures under GA alone were compared between groups, there remained a greater signal amplitude reduction in the R60 group compared with manual (53.2 ± 19.6 vs. 48.2 ± 26.1%, P = 0.02) and R30 (46.8 ± 20.8%, P < 0.001). Procedures under conscious sedation in the R60 group demonstrated greater signal attenuation compared with the R30 group, but did not reach statistical significance compared with the manual group (Figure 4). Catheter movement was less in the R60 group and R30 group compared with the manual group in both procedures under GA (2.5 ± 2.1 and 2.8 ± 2.1 vs. 4.1 ± 3.3 mm, P < 0.001 for both robotic groups) and procedures under conscious sedation (3.1 ± 2.1 and 3.2 ± 2.6 vs. 5.3 ± 3.7 mm) (Figure 5). Episodes of micromovement under GA were fewer in both the R60 and R30 group compared with manual (11 and 9 vs. 18%, P = 0.02 0.001, respectively). These results suggest that while procedures under GA benefit from improved catheter stability and greater signal attenuation, there is an additional effect on catheter stability and signal attenuation seen with robotic ablation compared with manual ablation in procedures performed under GA.
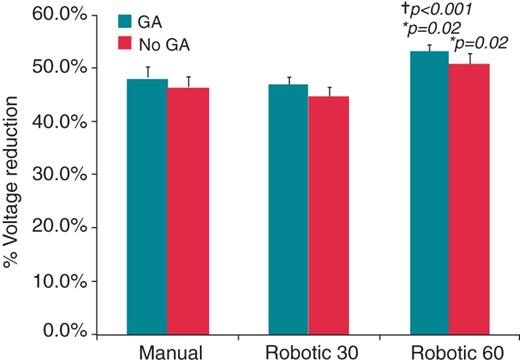
Local signal attenuation (mean ± SEM) compared for procedures conducted with and without general anaesthesia, further separated into manual and robotic 30 and robotic 60 groups. Significantly greater signal attenuation is seen in the robotic 60 group with general anaesthesia compared with both manual and robotic 30 groups with general anaesthesia. *P values comparing between manual and robotic 60 s group using Student's T-tests. †P values comparing between robotic 30 s and robotic 60 s groups using Student's T-tests.
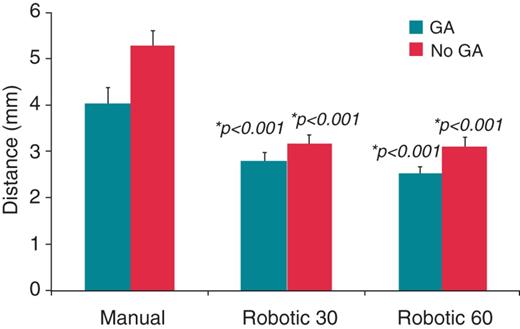
Distance (mean ± SEM) moved by the catheter tip during radiofrequency delivery compared for procedures conducted with and without general anaesthetic, further separated into manual and robotic 30 s and robotic 60 s groups. Significantly greater distances are seen with manual ablation compared with both robotic groups, in procedures with and without general anaesthesia. *P values comparing the robotic 30 s group with manual ablation and the robotic 60 s group with manual ablation. This was compared separately for general anaesthesia and non-general anaesthesia procedures.
Robotic navigation and location of circumferential pulmonary vein ablation
We analysed each case by right- or left-sided circumferential ablation. The average signal amplitude reduction for the right vs. left CPVA was 46.5 ± 25.3 vs. 48.8 ± 25.5% for Manual (P = ns), 48.7 ± 21.9 vs. 44.1 ± 24.7% for R30 (P= ns) and 52.7 ± 19.5 vs. 52.3 ± 19.5% for R60 (P= ns), no difference in ablation efficacy between right and left CPVA for each modality.
Outcomes
Our study was not powered to identify differences in outcome between robotic and manual ablation. However, a 12 month-follow-up data (mean follow-up 22.2 ± 9.3 months) is available for the 30 study patients and may provide preliminary insights into whether robotic ablation at higher powers has an effect on medium term outcomes. After an initial blanking period of 6 weeks, freedom from AF symptoms off all anti-arrhythmic drugs and 24 h Holter monitor with <30 s of atrial arrhythmia, was used to define procedural success. Six of 10 (60%) patients are free from AF recurrence at 12 months in the manual group, 5 of 10 (50%) patients in the robotic 30 group, whereas 8 of 10 patients (80%) in the robotic 60 group are free from AF recurrence at 12 months.
Discussion
Our study confirms there is greater stability with robotically assisted catheters during RF ablation compared with manual. However, at reduced power and duration of RF energy delivery (R30 group – our standard for robotic procedures), the signal amplitude reduction was not significantly different to manual ablation. This suggests we may have lost the benefit of improved catheter stability of robotic ablation by reducing RF power and duration. Increasing the duration of robotic ablation to 60 s significantly increased signal attenuation.
Previous studies comparing robotic and manual ablation have shown no significant difference in clinical outcomes. Although the primary aim of these studies was to prove safety and feasibility, it was generally anticipated that increased catheter precision and stability would lead to better clinical outcomes. While these studies used the same settings for both robotic and manual ablation (either 45 W for 20 s11 or 20–25 W for 60 s4) lesions, the reports comment on robotic ablation requiring shorter RF duration, implying that the operators adjusted power and time according to anatomical location and local signal attenuation. This would be considered standard practice at many centres where a target reduction in local electrogram of >80% is often quoted.21,23,24 However, we would argue that if a standardized change in local electrogram is used as an endpoint then it is likely that lesion quality will remain similar between manual or robotic approaches. Therefore, it may not be surprising that clinical outcomes remain similar. Furthermore, adjustment of RF parameters to achieve a desired level of local signal attenuation may not imply transmurality or the presence of a permanent lesion. Even when adjunctive mapping is used to confirm conduction block in the CPVA, there remains a significant PV reconnection rate even though these pre-specified signal attenuation targets have resulted in conduction block that presumably signifies a transmural effect. Therefore, our assumption is that the degree of signal attenuation needs to be increased to achieve permanent lesions. This approach would need to be balanced against the potential increased risk of cardiac perforation, atrio-oesophageal fistula formation, and phrenic nerve injury.
We have previously demonstrated in animal studies that at equivalent RF settings (25 W for 60 s, target pressure 20 g), robotic ablation was superior to manual ablation. One in vitro study looking at lesion transmurality and char formation with different RF powers, found that using 45 W at 20–30 g for 40 s, 83% of lesions were transmural and 33% of lesions were associated with char formation. Using lower RF settings of 30 W and 20–30 g pressure, no lesions were associated with char formation; however, only 16% were transmural.25 These studies suggest that 25 W for 60 s should be a safe power and duration for robotic ablation. Following the preliminary results from our randomized series that our current settings were not producing statistically significant differences in signal attenuation, we felt that increasing RF duration to 60 s would be a safe approach. Furthermore our previously published animal series suggested that these settings may be sufficient to produce transmural lesions.10
The current study also suggested a critical additive effect using general anaesthesia. Greater catheter stability and electrogram attenuation was seen in procedures conducted under general anaesthesia compared with those under conscious sedation. Furthermore, in procedures conducted under general anaesthesia statistically significant changes in signal attenuation were also seen with robotic ablation compared with manual ablation. On the basis of this pilot data, we would suggest that for any randomized comparison with manual ablation, the robotic ablation parameters should be 25 W for 60 s at 20 g pressure under general anaesthesia.
Limitations
Signal amplitude reduction has several limitations due to problems of combined local and far field signals. Catheter movement can produce both an increase and decrease in signal amplitude, and loss of local electrogram occurs with both effective ablation and poor tissue contact. Effective ablation causing local tissue oedema may also lead to catheter movement and an increase in local electrogram voltage. Our methods do not enable us to differentiate between causes for local electrogram increase and catheter displacement.
Furthermore, signal attenuation is simply a surrogate marker for ablation efficacy. While prior animal work has shown that greater signal attenuation is associated with more consistently transmural lesions, there is currently no evidence that greater signal attenuation leads to greater lesion transmurality in humans. A target signal attenuation of 80% has been quoted in the literature; however, there has been no study to date relating signal attenuation achievement and AF recurrence following ablation. This was a pilot study only and not designed to power for clinical outcomes.
Our method for stability measurement is limited since it only measures the distance between the start and finish catheter locations. However, these limitations affect both manual and robotic ablation modalities equally. Ideally, catheter excursion throughout RF delivery would be used to measure catheter stability.
Conclusions
Robotically assisted ablation using the Hansen system has the capability to produce greater local electrogram attenuation than manual ablation with appropriate RF parameter selection. The use of general anaesthesia appears to help achieve this benefit and randomized studies are required to assess clinical outcomes.
Conflict of interest: P.K. has received research grants and consulting fees from Hansen Medical. D.W.D. has received consulting fees from Hansen Medical.