-
PDF
- Split View
-
Views
-
Cite
Cite
François Regoli, Angelo Auricchio, The role of invasive mapping in the electrophysiology laboratory, EP Europace, Volume 11, Issue suppl_5, November 2009, Pages v40–v45, https://doi.org/10.1093/europace/eup271
- Share Icon Share
Abstract
The role of invasive mapping in the context of cardiac resynchronization has been essentially confined to improving knowledge of the depolarization processes and spread of ventricular activation. Experimental and limited clinical data obtained from high resolution three-dimensional contact and non-contact mapping have consistently pointed to the heterogeneity and the complexity of ventricular sequential activation in heart failure with conduction disturbance. The present article reviews current knowledge about activation mapping in patients with different types of ventricular conduction disturbance (right and left bundle branch block) putting this in the perspective of selection of most appropriate pacing site in cardiac resynchronization therapy (CRT) patients. Furthermore, recent comparative data of epicardial and endocardial pacing have been discussed. There is little doubt that invasive mapping will continue to contribute in a substantial manner to progresses in CRT especially in the new era of endocardial pacing. Therefore, it is possible to envision that endocardial mapping may serve to selectively target the most adequate positions for the left ventricular lead in order to optimize CRT.
Introduction
The characterization and understanding of the cardiac electrical activation sequence in vitro and in vivo has been the first goal of cardiac physiology and clinical electrophysiology. It also constitutes the foundation for the interpretation of cardiac mechanical events, particularly in patients with ventricular conduction disturbances, and it is relevant in the context of non-pharmacological therapy for heart failure such as cardiac resynchronization therapy (CRT). Although characterization of cardiac electrical activation may be obtained by different non-invasive techniques, catheter-based mapping is still considered the ‘gold standard’.
There is no doubt that routine evaluation of cardiac electrical activation sequence by catheter mapping in CRT patients are time-consuming, cumbersome, and it may carry some morbidity. However, catheter mapping may provide a unique characterization of the electrical substrate of CRT patients and offer insights into the haemodynamic changes conferred by CRT. It is possible that advancement in routine application of endocardial CRT may revitalize, in the near future, the interest in endocardial mapping for guiding pacing site selection. We are briefly reviewing current knowledge about activation mapping in patients with different types of ventricular conduction disturbance [right and left bundle branch block (RBBB and LBBB)] putting this in the perspective of selection of most appropriate pacing site in CRT patients. Furthermore, we will review recent comparative data of epicardial and endocardial pacing.
Mapping techniques
Invasive evaluation of cardiac activation sequence can be obtained either by conventional catheter-based mapping technique or using more sophisticated, high-resolution, three-dimensional (3D) catheter-based mapping systems. Conventional catheter-based mapping in dilated human hearts achieves only a gross estimate of the anatomy of the chambers and of their electrical activation sequence, given the limited number of recording sites that may be collected in a reasonable time and the lack of precision in marking specific anatomical locations. 1 , 2 In contrast, catheter-based 3D non-fluoroscopic contact and non-contact mapping techniques permit in vivo reconstruction of the cardiac anatomy and allow assessment of electrical activation sequence with high spatial and temporal resolution. Contact mapping more frequently uses bipolar signals that represent local changes in electrical events with an associated higher sensitivity to rapidly changing events and a lower sensitivity to slowly changing events. On the other hand, unipolar signals retain, at any given recording site, electrical information across the whole transmural thickness with equal sensitivity to fast and slow conduction. Given these different attributes, unipolar, and bipolar recordings may provide a more complete characterization and localization of electrical events, especially in severely diseased and dilated hearts associated with some degree of altered ventricular conduction. 3–5
Ventricular activation in left bundle branch block
LBBB is the most common ventricular conduction disturbance in heart failure patients and CRT candidates. Impulse propagation in dilated heart with LBBB is characterized by a great degree of complexity and heterogeneity. 3 , 6 , 7 High resolution integrated contact ( Figure 1 ) and non-contact ( Figure 2 ) 3D mapping data in LBBB heart failure patients have identified the earliest ventricular activation at the level of the right ventricle, with right ventricular (RV) breakthrough localized, by contact mapping, in a single or more points at the level of the antero-lateral region of the right ventricle. The activation front then spreads to the apex, septum, outflow tract, and to the basal region around the tricuspid valve. The activation of this chamber is quick due to the intact right-sided Purkinje system and it is usually completed within 60–80 ms. However, when the QRS duration increases, there is a progressive prolongation of RV activation which, in particular cases, may reach up to 130 ms ( Figure 1 ); in these cases, most of the delay occurs at RV outflow tract and at basal portion of right ventricle. Thus, proper position of RV lead in late activated portion of right ventricle (mid-septum or RV outflow tract) may become relevant especially in those patients with QRS duration >150 ms.
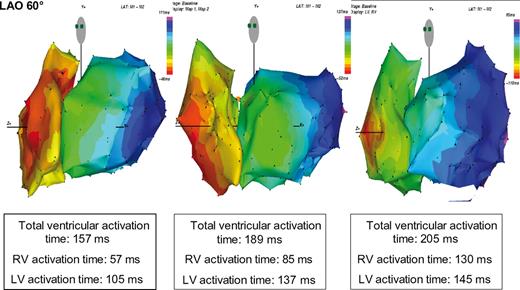
Colour-coded electroanatomical 10 ms isochronal maps (in LAO 60° projection), acquired with contact mapping system, of right ventricle and left ventricular (LV) activation in three patients with left bundle branch block and heart failure. Total ventricular activation time, and both right ventricular (RV) and LV activation times in each patients are reported. With the prolongation of the total duration time, the RV activation time increased proportionally more than the LV activation time.

Colour-coded electroanatomical isochronal maps (left half of the figure), acquired with non-contact mapping system, depicting two distinct patterns of LV activation in two different patients with LBBB, but different QRS durations. The right half of the figure presents the anatomical distribution of the line of block in 20 heart failure patients with left bundle branch, 3 stratified according to QRS duration of these patients, >150 ms and between 120 and 150 ms, respectively.
Although depolarization of the right ventricle takes place, the activation wavefront travels slowly to the left side of the septum (up to 70 ms) after earliest RV activation. Left ventricular (LV) endocardial breakthrough time in patients with LBBB shows a great patient-to-patient variability but aggregated in a bimodal distribution: normal (<20 ms) and abnormal (>40 ms) timing. About one-third of LBBB patients scheduled for CRT exhibit a normal, quick trans -septal time with a septo-basal or anterior endocardial breakthrough, whereas in the remaining patients presents a very slow transeptal breakthrough, localized in the mid/apical septum. A normal LV breakthrough time was noted in a large proportion of patients with a QRS duration between 120 and 150 ms, whereas the vast majority (80%) of patients with abnormal LV breakthrough time had a QRS duration >150 ms. The different detected patterns of septal breakthrough also characterize subsequent sequential activation of the rest of the left ventricle. The information derived from detailed 3D non-contact mapping 3 has shown that sequential LV activation propagates slowly and gradually to end at the level of the basal or lateral/postero-lateral wall, near the mitral valve annulus. The activation pattern shows a typical ‘U-shaped’ sequence turning around a mid-anterior line-of-block ( Figure 2 ). Different group of investigators, all using non-contact mapping technique, have independently confirmed this finding. 3 , 5 , 7 The line-of-block is characterized locally by small, multi-phasic, fragmented unipolar electrograms. The line-of-block showed two additional properties: (i) pacing tends to cause a shift in the line-of-block, suggesting that it is a ‘functional’ rather than ‘anatomical’ block (functional behaviour); (ii) unipolar signals, not local bipolar signals, localize the line, suggesting that conduction delay is localized intramurally rather than subendocardially. A clear association was observed between the localization of the line of block and the duration of QRS complex: when QRS duration was between 120 m and 150 ms, the line, if present, was shorter and located in a latero-basal or infero-basal position; when QRS duration was >150 ms, then the line of block was consistently located in the anterior region of left ventricle ( Figure 2 ). Coronary artery disease in general, and anterior myocardial scar, more specifically, has very little impact on the location of the line-of-block. This observation may have important clinical implication, namely that proper pacing site selection is more crucial in patients with QRS duration between 120 and 150 ms. Although there are several experimental and clinical data possibly supporting the hypothesis, no study has prospectively prospectively investigated the relationship between the site of line-of-block and response to CRT in relationship to pacing site. Helm et al . 8 showed that in a LBBB dog model (QRS duration comparable with human duration of >150 ms) with and without heart failure, there was a large, delayed lateral activation area and contraction. Optimized CRT was achieved over a fairly broad area of LV lateral wall in both non-failing and failing hearts, with modest anterior or posterior deviation still capable of providing effective CRT. Of note, sites selected to achieve the most mechanical synchrony were generally similar to those that most improved global function. Moreover, Fung et al . 7 showed that patients with a U-shaped activation pattern had significantly more mechanical dyssynchrony as detected by tissue-Doppler imaging compared with patients in whom a near normal LV activation pattern was evident.
Finally, Fantoni et al . 9 have suggested that breakthrough timing also influences the type of activation in the antero-septal region: in patients with a normal transeptal time, anteroseptal activation was mostly endocardial to epicardial; in patients with abnormal transeptal time activation, epicardial activation appeared to precede endocardial activation. This observation, however, requires confirmation by other authors and may have implications in light of future endocardial LV pacing.
Ventricular activation in right bundle branch block
Approximately 10% of potential heart failure candidates for CRT present a RBBB QRS morphology. Both conventional catheter mapping and 3D high-resolution electroanatomical catheter mapping techniques have shown in RBBB a mirror image of the activation pattern seen in LBBB patients. 4 Thus, the activation starts at the LV side of the septum and spread nearly in a normal fashion across the left ventricle. In contrast, the entire RV activation starts late (50–70 ms) with a breakthrough at mid portion of the RV septum. Finally, the right free wall and the basal portion of the right ventricle is activated ( Figure 3 ). Recent haemodynamic data collected in a RBBB dog model confirm the finding that in RBBB the right free wall is delayed and pacing at this site significantly improved mechanical ventricular function. However, less mechanical dyssynchrony is induced by RBBB than LBBB in failing hearts, and the corresponding impact of CRT on the former is reduced. Of note, RV-only pacing may be equally efficacious as BiV CRT in hearts with pure right bundle branch conduction delay. 4
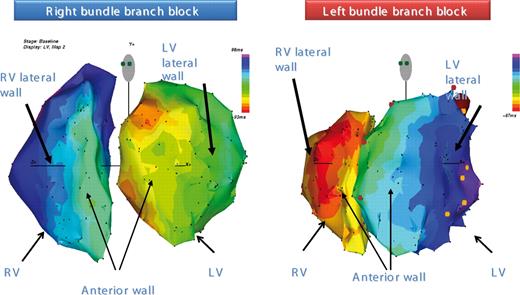
Detailed high resolution colour-coded electroanatomical isochronal maps, acquired with contact mapping system, that show RV and LV activations in two heart failure patients. Activation of the two ventricles is shown, on the left, for a patient with right bundle branch block and, on the right, for a patients with left bundle-branch block.
It is important to note that in the vast majority of CRT patients, the QRS morphology is significantly different from the characteristic RBBB appearing in otherwise structurally normal hearts. Indeed, many of these patients demonstrate a specific ECG pattern defined by Rosenbaum 10 as ‘RBBB masking LBBB’. This ECG pattern is characterized by a broad, slurred, eventually notched R wave on lead I and aVL, a left axis deviation and a notched, broad QRS in V1 and V2. Recent electronanatomical mapping have demonstrated that, in patients with this particular ECG pattern, not only right ventricle is abnormally delayed, but LV activation is also delayed as much as in patients presenting with isolated LBBB. 4 There are some potential clinical consequences for RBBB patients treated by CRT. It is well-known that CRT patients with RBBB show less symptomatic and smaller magnitude of reverse remodelling than CRT patients with LBBB. 11 , 12 Some major important clinical and haemodynamic differences (coronary artery disease is more prevalent in RBBB patient, both RV and LV ejection fraction are usually more depressed in RBBB patients compared with LBBB patients) may account for the difference. However, it may be equally possible that in these patients, RV lead should be placed in a slightly different position from conventional apical or mid RV septum; the RV outflow tract or the free wall of the right ventricle may be more appropriate. Although no clinical data can support this statement, interpretation of 3D-electroanatomical mapping strongly suggest a change in implantation strategy in this selected group of CRT patients.
Ventricular activation during cardiac resynchronization therapy
Detailed characterization of the electrical activation during atrial synchronous biventricular or LV pacing alone has been investigated mostly in computer simulation model, 13 experimental model, 14 and, to a limited extent, in patients. 5 Translation of observations made in animal model to patients may be difficult due to the inability to reproduce in a dog model substrates similar to what usually both heart failure and chronic ischaemic disease may create in human being. Moreover, there is very little understanding about the complex relationship between electrical activation and mechanical contraction during CRT. Indeed, it is commonly observed that LV pacing alone at the most optimal pacing site may result in very broad QRS duration but provides at least as much improvement in LV contractility as biventricular pacing, which at most optimal atrioventricular (AV) delay usually shows a shorter QRS duration than LV pacing alone.
Detailed epicardial and endocardial characterization of the electrical activation during atrial synchronous LV epicardial single site pacing in an animal model with normal ventricular function has been recently provided. This gives us very useful insights into how electrical synchrony may arise during CRT delivered by LV pacing alone. Faris et al . 15 evaluated the transition from dyssynchronous to synchronous LV activation pattern by constructing activation time maps for both endo- and epicardial surfaces, as a function of lengthening the AV delay. They showed that, at short AV delays during epicardial LV pacing, LV activation pattern was asynchronous in both the endocardial and the epicardial surfaces without possibility of merging with the intrinsic conduction which was travelling through the right bundle branch. In this situation, both inter- and intra-ventricular electrical asynchrony were usually increased. In contrast, at longer AV delays, the electrical activation pattern became more synchronous in both the epicardial and the endocardial surfaces, due to increasing degree of fusion between the wavefront propagating from the LV epicardial pacing site and the intrinsic wavefront originating from the right bundle branch. This activation pattern led to a significant reduction of both inter- and intra-ventricular electrical asynchrony. Finally, at very long AV delays, electrical asynchrony increased again, indicating that the heart was primarily activated from the intrinsic conduction system through the right bundle branch, rather than from the LV stimulus. In this latter situation, activation of both ventricles resembled what is usually observed during intrinsic LBBB, with consequent significant inter- as well as intra-ventricular electrical asynchrony. Interestingly, the transition from dyssynchronous to synchronous activation occurred at similar AV delays in the epicardial and in endocardial surfaces.
Characterization of the ventricular activation sequence during single site LV pacing or during biventricular pacing in humans is considerably limited. Technical limitations in collection of enough mapping points in the same ventricular region and at different AV timing, the potential risk related to lengthy mapping procedures, and anatomical limitation due to coronary vein availability and eventually the limited number of epicardial veins, are all potential issues in precise characterization of ventricular activation sequence of left and right ventricles during pacing. Moreover, scar and myocardial fibre disarray, as frequently observed in patients with coronary artery disease, may influence in an important manner the merging of the two stimulation wavefronts during CRT.
Where to go from here: prospects of endocardial pacing
Until recently, interest in endocardial pacing has been minimal and comparison to epicardial pacing has only been anecdotal in both animal models and patients. Novel endocardial wireless pacing technology have immediately captured interest because of the theoretical possibility to pace the heart in a more physiological manner.
Recently, van Deursen et al . 14 and Rademaker et al . 16 have shown in an LBBB animal model with and without chronic myocardial infarction, respectively, that compared with conventional LV epicardial pacing-based CRT, endocardial CRT produces more homogeneous and rapid ventricular electrical depolarization and repolarization, and additional improvement in systolic LV pump function. With LV endocardial pacing, the beneficial effects of CRT with single site LV pacing are less dependent on the timing and position of LV pacing than with an epicardial LV electrode. As compared with epicardial CRT, endocardial CRT provides less transmural dispersion of repolarization. These authors postulated that the crucial parameter underlying the acute haemodynamic benefit of endocardial CRT over conventional epicardial CRT was most likely attributable to electrical resynchronization. Intuitively, the path length for the depolarization wave to reach all regions of the ventricles is smaller, because LV endocardial sites are more centrally located than LV epicardial sites. Moreover earlier studies indicated that subendocardial non-Purkinje fibres conduct impulses faster, especially in the longitudinal direction. A further factor contributing to faster activation during endocardial LV pacing is the more rapid transmural conduction from endocardium to epicardium than in the opposite direction. Consequently, the activation waves originating from the LV and RV electrode coalesce more rapidly during endocardial biventricular pacing than during conventional epicardial biventricular pacing. More rapid impulse conduction, resulting in broader activation wavefronts, facilitates the achievement of sufficient fusion between intrinsic conduction and/or RV pacing and LV pacing derived wavefronts. Broader activation wavefronts during endocardial LV pacing is a likely explanation of why resynchronization was less dependent on the exact site of LV pacing. Epicardial biventricular pacing did not resynchronize significantly when pacing at basal anterior or posterior or the midventricular posterior sites, whereas all endocardial pacing sites resulted in significant resynchronization.
High resolution 3D mapping studies 3 , 5 in HF patients have allowed to further delineate conductive properties of the LV sub-endocardial stratum in the diseased heart with LBBB. Auricchio et al . 3 described a ‘U-shaped’ activation propagation pattern during LBBB which was appreciated through non-contact mapping and not contact mapping, thus suggesting the presence of intra-mural, rather than sub-endocardial conduction delay. Therefore, it is assumed that the impulse may propagate more easily along the sub-endocardial surface.
There is very limited comparative data about endocardial and epicardial LV or biventricular pacing in the same patients. Garrigue et al ., 17 using a trans -septal approach for positioning the endocardial LV lead, has reported the chronic effects of endocardial delivered biventricular pacing compared with epicardial. Although both pacing modalities yielded symptomatic benefits in the follow-up, endocardial biventricular pacing determined more homogenous intraventricular resynchronization and was associated with significantly better LV filling and systolic performance. More recently, Lambiase et al . 5 described areas of slow conduction along the endocardial surface (prevalent amongst patients with ischaemic etiology), but endocardial points where conduction was better would yield important improvements of cardiac output. In fact, largest haemodynamic improvement, shortest LV endocardial activation time, and narrowest QRS complex were obtained when pacing simultaneously at RV and LV endocardium.
Conclusions
Taken together, detailed high resolution electroanatomic invasive mapping shows how, despite ECG morphological feature may be homogenous and similar in patients with either LBBB or RBBB, electrical sequential LV activation is complex and heterogeneous. Certainly, these novel notions on activation sequence of both right and left ventricle may carry a number of implications for CRT.
Invasive mapping has played a key role for the technical advancement of CRT. On one hand, such studies have enhanced our knowledge about electrical disarrangement and eventually how resynchronization of a diseased substrate may occur. There is little doubt that invasive mapping will continue to contribute in a substantial manner to progresses in CRT especially in the new era of endocardial pacing. Therefore, it is possible to envision that endocardial mapping may serve to selectively target the most adequate positions for the LV lead in order to optimize CRT.
Conflict of interest: A.A. received speaker's fees from Biotronik, Medtronic, Sorin, GE Healthcare, and Philips. He is a consultant for Medtronic, Biotronik, Sorin, EBR, and Proteus. Both A.A. and F.R. report that their institution has research contracts with Medtronic, Sorin, Biotronik, and St. Jude.