-
PDF
- Split View
-
Views
-
Cite
Cite
Hung-Fat Tse, Kwong-Kuen Wong, Chung-Wah Siu, Man-Oi Tang, Vella Tsang, Wai-Yin Ho, Chu-Pak Lau, Impacts of ventricular rate regularization pacing at right ventricular apical vs. septal sites on left ventricular function and exercise capacity in patients with permanent atrial fibrillation, EP Europace, Volume 11, Issue 5, May 2009, Pages 594–600, https://doi.org/10.1093/europace/eup087
- Share Icon Share
Abstract
The deleterious effects of right ventricular apex (RVA) pacing may offset the potential benefit of ventricular rate (VR) regularization during atrial fibrillation (AF). Recent studies suggested that right ventricular septal (RVS) pacing may prevent the potential deleterious effects of RVA pacing and enhance the VR regularization (VRR) with ventricular pacing due to closer proximity of the pacing site to the retrograde atrioventricular conduction.
We randomized 24 patients with permanent AF and symptomatic bradycardia to undergo RVA ( n = 12) or RVS ( n = 12) pacing. A VRR algorithm was programmed for all patients at 6-month after implantation. All patients underwent 6 min hall walk (6MHW) to assess exercise capacity at 6, 12, and 24 months, and radionuclide ventriculography to determine left ventricular ejection fraction (LVEF) at 6 and 24 months. Baseline characteristics were comparable in both groups except pacing QRS duration was significantly shorter during RVS pacing than RVA pacing (132 ± 4 vs. 151 ± 6 ms, P = 0.012). In both groups, VRR significantly increased the percentage of ventricular pacing and reduced VR variability ( P < 0.05) without increasing mean VR ( P > 0.05). At 6 months, 6MHW and LVEF were comparable in patients with RVA and RVS pacing ( P > 0.05). At 24 months, patients with RVA pacing had significant decreases in LVEF and 6MHW after VRR pacing ( P < 0.05), whereas RVS pacing with VRR preserved LVEF and improved 6MHW ( P < 0.05).
In patients with permanent AF, VRR pacing at RVS, but not at RVA, preserves LVEF and provides incremental benefit for exercise capacity.
Introduction
Atrial fibrillation (AF) is the most common cardiac arrhythmia and confers substantial mortality and morbidity from stroke, thrombo-embolism, heart failure, and impaired quality of life. 1 Pooled analyses of prospective clinical trials have failed to show any statistically significant differences between rate-control vs. rhythm-control strategies with respect to mortality or rates for major bleeding and thrombo-embolic events. 2 Rate-control is associated with a lesser improvement in exercise capacity than rhythm-control in patients with AF. 3–5 In addition, it is difficult to achieve ventricular rate (VR) control during AF using pharmacological agents alone. In the AFFIRM study, optimal VR during AF was achieved in only 70% of patients in the rate-control arm. 6 On the other hand, the use of rate-control drugs to achieve VR control can frequently blunt the heart rate response during exercise and have no effect on irregularity of the VR. This can contribute to impaired exercise capacity in patients with AF. 7 , 8 Indeed, the optimal VR control during AF is still remain unclear. 9 Pacemaker implantation is also more commonly required if a strict VR control strategy is applied. 9 The hybrid use of permanent pacing in patients with AF has consequently been suggested to provide rate adaptation during exercise, to avoid bradycardia, and to regulate VR. 10
Acute haemodynamic studies have revealed that regulation of VR with ventricular overdrive pacing improves cardiac performance. 11–14 Nevertheless clinical studies that used different ventricular overdrive algorithms demonstrated only modest or no significant improvement in symptoms, quality of life, or functional capacity. 15–17 This may be due to deleterious effects of long-term right ventricular apical (RVA) pacing that offset any potential benefit of VR regularization (VRR). 18 , 19 Recent clinical studies 20–23 suggest that right ventricular septal (RVS) pacing may prevent the long-term adverse effects of RVA pacing on left ventricular (LV) function. The purpose of this prospective, randomized study was to compare the effects of VRR by permanent pacing at RVA vs. RVS on LV function and exercise capacity in patients with permanent AF.
Methods
Patients
The study included 30 consecutive patients, 24 men and 6 women, mean age (±SEM) 74 ± 3 years (range: 45–87 years), with permanent AF and symptomatic bradycardia who were admitted for pacemaker implantation. All patients had intact atrioventricular (AV) conduction with an average heart rate of 60–120 bpm on 24 h Holter recording. Patients with a previous history of coronary artery disease, significant valve disease, congestive heart failure, or left ventricular ejection fraction (LVEF) <50% on baseline screening echocardiography were excluded from the study as the progression of their underlying diseases may affect their LVEF on follow-up. The study protocol was approved by the local Ethics Committee, and all patients gave informed consent.
Study protocol
All patients underwent implantation of a single-chamber rate-adaptive pacemaker with specific VRR algorithm (Insignia, Boston Scientific, USA). Patients were randomized using a randomization table at the time of implantation to receive a ventricular lead at either the RVA (passive-fixation lead) or high mid-RVS (active-fixation lead) position as described previously. 20 An ECG using surface limb leads (I, II, III, aVR, aVL, and aVF) and paper speed of 100 mm/s was recorded during the procedure: QRS duration was measured from the earliest to the latest deflection of the QRS complex. All ventricular pacing leads were positioned at a stable position to obtain a satisfactory pacing threshold value and R-wave sensing value.
Following device implantation, all pacemakers were programmed to VVIR mode with a lower rate limit of 60 bpm and VRR algorithm OFF for 6 months to allow lead maturation and optimization of AV nodal blocking agents. During this period, AV nodal blocking agents in all patients were optimized to achieve a mean VR of <90 bpm as determined by the device counter. Doses of AV nodal blocking agents were stable for ≥3 weeks prior to study commencement.
At 6 months follow-up, all patients underwent a 6 min hall walk (6MHW) test 18 to assess functional capacity and radionuclide ventriculography 22 to determine LVEF. Then, VRR algorithm was programmed ON in all patients for a further 18 months. The 6MHW test was repeated at 12 and 24 months follow-up in all patients; radionuclide ventriculography was performed at 24-month follow-up. The 6MHW and radionuclide ventriculography were performed and analysed by investigators who were blinded to the pacing sites of the patients.
Pacemaker diagnostics and pacing algorithm
The VRR algorithm is designed to regularize the VR during AF by ventricular pacing close to the mean VR and following its mean fluctuations over time based on a weighted average of the last 32 sensed and paced RR intervals. 19 At 6, 12, and 24 months follow-up, data were recorded for the percentage of overall paced ventricular beats, average daily VR, and the degree of VR variability as determined by specific counters based on the difference between contiguous RR intervals. The percentage of time with the difference of RR interval >30% (RR < 30) was used to assess the degree of VR variability as described previously. 19
Radionuclide ventriculography
Multiple-gated equilibrium blood pool imaging was performed at rest to determine the global LV systolic and diastolic function at 6- and 18-month follow-up as described previously. 22 In brief, patients’ red blood cells were labelled with 20–30 mCi of technetium-99m, and global LVEF and diastolic function were determined with the commercially available software.
Statistical analysis
Data are expressed as mean ± 1 standard error of the mean (SEM). Categorical data are presented as frequencies and percentages. Comparison of baseline characteristics between groups was performed using Student’s t -test or χ 2 test, when appropriate. Comparisons of variables between baseline and follow-up were performed using repeated ANOVA followed by Bonferroni multiple comparison. Calculations were performed with SPSS software (version 14.0). A P -value of <0.05 was considered statistically significant.
Results
Patient characteristics
During implantation, 15 patients were randomized to receive RVA pacing and 15 patients to receive RVS pacing. There were no significant differences in the age, gender distribution, and baseline LVEF by echocardiography between the two groups (all P > 0.05). No complications or lead dislodgement was observed during the study, and all patients were in permanent AF throughout the study period. Of the 30 patients enrolled, 2 patients died of chest infection and 2 of malignancy (at 1, 3, 7, and 8 months, respectively); 2 patients developed stroke (at 3 and 6 months, respectively) were excluded as no baseline LVEF and functional capacity could be assessed at 6 months.
The final analysis included 24 patients who completed the study protocol, and their baseline clinical characteristics are shown in Table 1 . Twelve patients were randomized to RVA pacing and 12 to RVS pacing. Their overall mean age was 71 ± 2 years (5 men and 19 women). Twelve patients were randomized to RVA pacing and 12 to RVS pacing. There was no significant difference in the age, gender distribution, prevalence of underlying medical illness, rate-control agents, baseline LVEF by echocardiography, VR, blood pressure, or pacing parameter at implant between the two groups. The mean QRS duration was nonetheless significantly shorter during RVS pacing compared with RVA pacing ( Table 1 ).
. | RVA ( n = 12) . | RVS ( n = 12) . | P -value . |
---|---|---|---|
Age (years) | 72.3 ± 3 | 70.3 ± 1 | 0.12 |
Male [ n (%)] | 2 (16) | 3 (25) | 1.0 |
Intrinsic rhythm [ n (%)] | |||
LBBB | 1 (8) | 1 (8) | 1.0 |
RBBB | 1 (8) | 2 (17) | 1.0 |
Narrow QRS (<120 ms) | 10 (83) | 9 (75) | 1.0 |
Other medical illness [ n (%)] | |||
Diabetes | 2 (17) | 2 (17) | 1.0 |
Hyperlipidaemia | 1 (8) | 2 (17) | 1.0 |
Hypertension | 6 (50) | 9 (42) | 1.0 |
Medications [ n (%)] | |||
Digoxin | 4 (33) | 4 (33) | 1.0 |
β-Blocker | 3 (25) | 5 (42) | 1.0 |
Diltiazem | 5 (42) | 4 (33) | 1.0 |
Baseline echocardiography | |||
LVEF (%) | 58 ± 4 | 59 ± 5 | 0.74 |
Mean ventricular rate a (bpm) | 104.3 ± 6.1 | 108.2 ± 4.3 | 0.87 |
Mean systolic blood pressure (mmHg) | 146 ± 8 | 142 ± 6 | 0.45 |
Mean diastolic blood pressure (mmHg) | 87 ± 4 | 85 ± 6 | 0.67 |
Pacing parameters at implant | |||
Ventricular pacing threshold (V/0.5 ms) | 1.2 ± 0.7 | 1.2 ± 0.5 | 0.73 |
R-wave amplitude (mV) | 11.1 ± 2.3 | 9.8 ± 4.2 | 0.60 |
Impedance (Ω) | 620 ± 87 | 720 ± 88 | 0.56 |
Pacing QRS duration (ms) | 152.8 ± 6.6 | 135.2 ± 5.0 | 0.035 |
. | RVA ( n = 12) . | RVS ( n = 12) . | P -value . |
---|---|---|---|
Age (years) | 72.3 ± 3 | 70.3 ± 1 | 0.12 |
Male [ n (%)] | 2 (16) | 3 (25) | 1.0 |
Intrinsic rhythm [ n (%)] | |||
LBBB | 1 (8) | 1 (8) | 1.0 |
RBBB | 1 (8) | 2 (17) | 1.0 |
Narrow QRS (<120 ms) | 10 (83) | 9 (75) | 1.0 |
Other medical illness [ n (%)] | |||
Diabetes | 2 (17) | 2 (17) | 1.0 |
Hyperlipidaemia | 1 (8) | 2 (17) | 1.0 |
Hypertension | 6 (50) | 9 (42) | 1.0 |
Medications [ n (%)] | |||
Digoxin | 4 (33) | 4 (33) | 1.0 |
β-Blocker | 3 (25) | 5 (42) | 1.0 |
Diltiazem | 5 (42) | 4 (33) | 1.0 |
Baseline echocardiography | |||
LVEF (%) | 58 ± 4 | 59 ± 5 | 0.74 |
Mean ventricular rate a (bpm) | 104.3 ± 6.1 | 108.2 ± 4.3 | 0.87 |
Mean systolic blood pressure (mmHg) | 146 ± 8 | 142 ± 6 | 0.45 |
Mean diastolic blood pressure (mmHg) | 87 ± 4 | 85 ± 6 | 0.67 |
Pacing parameters at implant | |||
Ventricular pacing threshold (V/0.5 ms) | 1.2 ± 0.7 | 1.2 ± 0.5 | 0.73 |
R-wave amplitude (mV) | 11.1 ± 2.3 | 9.8 ± 4.2 | 0.60 |
Impedance (Ω) | 620 ± 87 | 720 ± 88 | 0.56 |
Pacing QRS duration (ms) | 152.8 ± 6.6 | 135.2 ± 5.0 | 0.035 |
Data expressed as mean ± SEM. LBBB, left bundle branch block; LVEF, left ventricular ejection fraction; RBBB, right bundle branch block; RVA, right ventricular apex; RVS, right ventricular septum.
a Average ventricular rate from 24 h Holter recording.
. | RVA ( n = 12) . | RVS ( n = 12) . | P -value . |
---|---|---|---|
Age (years) | 72.3 ± 3 | 70.3 ± 1 | 0.12 |
Male [ n (%)] | 2 (16) | 3 (25) | 1.0 |
Intrinsic rhythm [ n (%)] | |||
LBBB | 1 (8) | 1 (8) | 1.0 |
RBBB | 1 (8) | 2 (17) | 1.0 |
Narrow QRS (<120 ms) | 10 (83) | 9 (75) | 1.0 |
Other medical illness [ n (%)] | |||
Diabetes | 2 (17) | 2 (17) | 1.0 |
Hyperlipidaemia | 1 (8) | 2 (17) | 1.0 |
Hypertension | 6 (50) | 9 (42) | 1.0 |
Medications [ n (%)] | |||
Digoxin | 4 (33) | 4 (33) | 1.0 |
β-Blocker | 3 (25) | 5 (42) | 1.0 |
Diltiazem | 5 (42) | 4 (33) | 1.0 |
Baseline echocardiography | |||
LVEF (%) | 58 ± 4 | 59 ± 5 | 0.74 |
Mean ventricular rate a (bpm) | 104.3 ± 6.1 | 108.2 ± 4.3 | 0.87 |
Mean systolic blood pressure (mmHg) | 146 ± 8 | 142 ± 6 | 0.45 |
Mean diastolic blood pressure (mmHg) | 87 ± 4 | 85 ± 6 | 0.67 |
Pacing parameters at implant | |||
Ventricular pacing threshold (V/0.5 ms) | 1.2 ± 0.7 | 1.2 ± 0.5 | 0.73 |
R-wave amplitude (mV) | 11.1 ± 2.3 | 9.8 ± 4.2 | 0.60 |
Impedance (Ω) | 620 ± 87 | 720 ± 88 | 0.56 |
Pacing QRS duration (ms) | 152.8 ± 6.6 | 135.2 ± 5.0 | 0.035 |
. | RVA ( n = 12) . | RVS ( n = 12) . | P -value . |
---|---|---|---|
Age (years) | 72.3 ± 3 | 70.3 ± 1 | 0.12 |
Male [ n (%)] | 2 (16) | 3 (25) | 1.0 |
Intrinsic rhythm [ n (%)] | |||
LBBB | 1 (8) | 1 (8) | 1.0 |
RBBB | 1 (8) | 2 (17) | 1.0 |
Narrow QRS (<120 ms) | 10 (83) | 9 (75) | 1.0 |
Other medical illness [ n (%)] | |||
Diabetes | 2 (17) | 2 (17) | 1.0 |
Hyperlipidaemia | 1 (8) | 2 (17) | 1.0 |
Hypertension | 6 (50) | 9 (42) | 1.0 |
Medications [ n (%)] | |||
Digoxin | 4 (33) | 4 (33) | 1.0 |
β-Blocker | 3 (25) | 5 (42) | 1.0 |
Diltiazem | 5 (42) | 4 (33) | 1.0 |
Baseline echocardiography | |||
LVEF (%) | 58 ± 4 | 59 ± 5 | 0.74 |
Mean ventricular rate a (bpm) | 104.3 ± 6.1 | 108.2 ± 4.3 | 0.87 |
Mean systolic blood pressure (mmHg) | 146 ± 8 | 142 ± 6 | 0.45 |
Mean diastolic blood pressure (mmHg) | 87 ± 4 | 85 ± 6 | 0.67 |
Pacing parameters at implant | |||
Ventricular pacing threshold (V/0.5 ms) | 1.2 ± 0.7 | 1.2 ± 0.5 | 0.73 |
R-wave amplitude (mV) | 11.1 ± 2.3 | 9.8 ± 4.2 | 0.60 |
Impedance (Ω) | 620 ± 87 | 720 ± 88 | 0.56 |
Pacing QRS duration (ms) | 152.8 ± 6.6 | 135.2 ± 5.0 | 0.035 |
Data expressed as mean ± SEM. LBBB, left bundle branch block; LVEF, left ventricular ejection fraction; RBBB, right bundle branch block; RVA, right ventricular apex; RVS, right ventricular septum.
a Average ventricular rate from 24 h Holter recording.
At 6-month follow-up, there was also no significant difference in rate-control agents, LVEF by radionuclide ventriculogram, VR, blood pressure, or pacing parameter between the two groups. Similarly, the pacing QRS duration remained significantly shorter during RVS pacing compared with RVA pacing ( Table 2 ).
. | RVA ( n = 12) . | RVS ( n = 12) . | P -value . |
---|---|---|---|
Medications [ n (%)] | |||
Digoxin | 8 (67) | 9 (75) | 1.0 |
β-Blocker | 7 (58) | 6 (50) | 1.0 |
Diltiazem | 8 (67) | 7 (58) | 1.0 |
Radionuclide ventriculogram | |||
LVEF (%) | 59.6 ± 1.9 | 59.5 ± 2.4 | 0.98 |
Mean ventricular rate a (bpm) | 74.6 ± 10.5 | 71.8 ± 3.2 | 0.25 |
Mean systolic blood pressure (mmHg) | 136 ± 8 | 135 ± 6 | 0.87 |
Mean diastolic blood pressure (mmHg) | 85 ± 4 | 83 ± 5 | 0.58 |
Pacing parameters at 6 months | |||
Ventricular pacing threshold (V/0.5 ms) | 1.1 ± 0.5 | 1.3 ± 0.6 | 0.63 |
R-wave amplitude (mV) | 11.5 ± 3.5 | 10.1 ± 3.5 | 0.50 |
Impedance (Ω) | 590 ± 67 | 670 ± 73 | 0.65 |
Pacing QRS duration (ms) | 150.6 ± 5.6 | 132.6 ± 4.0 | 0.012 |
. | RVA ( n = 12) . | RVS ( n = 12) . | P -value . |
---|---|---|---|
Medications [ n (%)] | |||
Digoxin | 8 (67) | 9 (75) | 1.0 |
β-Blocker | 7 (58) | 6 (50) | 1.0 |
Diltiazem | 8 (67) | 7 (58) | 1.0 |
Radionuclide ventriculogram | |||
LVEF (%) | 59.6 ± 1.9 | 59.5 ± 2.4 | 0.98 |
Mean ventricular rate a (bpm) | 74.6 ± 10.5 | 71.8 ± 3.2 | 0.25 |
Mean systolic blood pressure (mmHg) | 136 ± 8 | 135 ± 6 | 0.87 |
Mean diastolic blood pressure (mmHg) | 85 ± 4 | 83 ± 5 | 0.58 |
Pacing parameters at 6 months | |||
Ventricular pacing threshold (V/0.5 ms) | 1.1 ± 0.5 | 1.3 ± 0.6 | 0.63 |
R-wave amplitude (mV) | 11.5 ± 3.5 | 10.1 ± 3.5 | 0.50 |
Impedance (Ω) | 590 ± 67 | 670 ± 73 | 0.65 |
Pacing QRS duration (ms) | 150.6 ± 5.6 | 132.6 ± 4.0 | 0.012 |
Data expressed as mean ± SEM. Abbreviation as in Table 1 .
a Average ventricular rate from device telemetry recording.
. | RVA ( n = 12) . | RVS ( n = 12) . | P -value . |
---|---|---|---|
Medications [ n (%)] | |||
Digoxin | 8 (67) | 9 (75) | 1.0 |
β-Blocker | 7 (58) | 6 (50) | 1.0 |
Diltiazem | 8 (67) | 7 (58) | 1.0 |
Radionuclide ventriculogram | |||
LVEF (%) | 59.6 ± 1.9 | 59.5 ± 2.4 | 0.98 |
Mean ventricular rate a (bpm) | 74.6 ± 10.5 | 71.8 ± 3.2 | 0.25 |
Mean systolic blood pressure (mmHg) | 136 ± 8 | 135 ± 6 | 0.87 |
Mean diastolic blood pressure (mmHg) | 85 ± 4 | 83 ± 5 | 0.58 |
Pacing parameters at 6 months | |||
Ventricular pacing threshold (V/0.5 ms) | 1.1 ± 0.5 | 1.3 ± 0.6 | 0.63 |
R-wave amplitude (mV) | 11.5 ± 3.5 | 10.1 ± 3.5 | 0.50 |
Impedance (Ω) | 590 ± 67 | 670 ± 73 | 0.65 |
Pacing QRS duration (ms) | 150.6 ± 5.6 | 132.6 ± 4.0 | 0.012 |
. | RVA ( n = 12) . | RVS ( n = 12) . | P -value . |
---|---|---|---|
Medications [ n (%)] | |||
Digoxin | 8 (67) | 9 (75) | 1.0 |
β-Blocker | 7 (58) | 6 (50) | 1.0 |
Diltiazem | 8 (67) | 7 (58) | 1.0 |
Radionuclide ventriculogram | |||
LVEF (%) | 59.6 ± 1.9 | 59.5 ± 2.4 | 0.98 |
Mean ventricular rate a (bpm) | 74.6 ± 10.5 | 71.8 ± 3.2 | 0.25 |
Mean systolic blood pressure (mmHg) | 136 ± 8 | 135 ± 6 | 0.87 |
Mean diastolic blood pressure (mmHg) | 85 ± 4 | 83 ± 5 | 0.58 |
Pacing parameters at 6 months | |||
Ventricular pacing threshold (V/0.5 ms) | 1.1 ± 0.5 | 1.3 ± 0.6 | 0.63 |
R-wave amplitude (mV) | 11.5 ± 3.5 | 10.1 ± 3.5 | 0.50 |
Impedance (Ω) | 590 ± 67 | 670 ± 73 | 0.65 |
Pacing QRS duration (ms) | 150.6 ± 5.6 | 132.6 ± 4.0 | 0.012 |
Data expressed as mean ± SEM. Abbreviation as in Table 1 .
a Average ventricular rate from device telemetry recording.
Pacemaker diagnostics
At 6 months, the overall mean percentage of paced ventricular beats, average daily VR, and percentage time with RR <30 was 56.0 ± 4.2%, 73.1 ± 5.6 bpm, and 19.3 ± 1.8%, respectively. The mean percentage of overall paced ventricular beats was significantly increased at 12 months (74.3 ± 6.5%, P = 0.01) and at 24 months (77.0 ± 9.4%, P = 0.008) compared with 6 months ( Figure 1 ). Furthermore, the mean percentage time with RR < 30 was significantly decreased at 12 months (7.9 ± 2.0%, P < 0.001) and 24 months (6.3 ± 1.6%, P < 0.001) compared with 6 months ( Figure 1 ). However, the overall average daily VR at 12 months (65.3 ± 3.4 bpm, P = 0.12) and at 24 months (66.9 ± 3.2 bpm, P = 0.24) was similar to those at 6 months ( Figure 1 ).
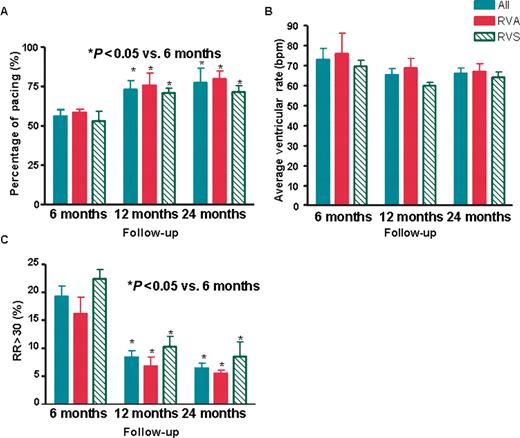
Changes in ( A ) percentage of pacing; ( B ) average ventricular rate; and ( C ) the percentage of time with the difference of RR interval >30% (RR30) in all patients ( n = 24), and in patients with right ventricular apical (RVA, n = 12) and right ventricular septal (RVS, n = 12) pacing at 6, 12, and 24 months of follow-up. Data are expressed as mean ± SEM. * P -values determined by ANOVA with Bonferroni multiple comparison tests.
At 6 months, there were no significant differences in the mean percentage of paced ventricular beats (59.3 ± 2.7 vs. 52.7 ± 7.6%, P = 0.18), average daily VR (74.6 ± 10.5 vs. 71.8 ± 3.2 bpm, P = 0.25), and percentage time with RR < 30 (16.5 ± 4.3 vs. 22.5 ± 2.4%, P = 0.34 ) between patients with RVA and RVS pacing ( Figure 1 ). In both groups, the mean percentage of paced ventricular beats increased and the mean percentage time with RR < 30 decreased at 12 and 24 months when compared with 6 months ( Figure 1 ). However, there were no significant changes in the average daily VR in both groups at 12 and 24 months compared with 6 months ( Figure 1 ).
The mean percentage of paced ventricular beats at 12 months (75.2 ± 9.8 vs. 73.6 ± 4.3%, P = 0.56) and at 24 months (79.3 ± 6.4 vs. 74.5 ± 4.5%, P = 0.12); the mean percentage time with RR < 30 at 12 months (6.1 ± 2.1 vs. 10.2 ± 3.2%, P = 0.21) and at 24 months (5.1 ± 1.2 vs. 7.4 ± 2.9%, P = 0.34); and the average daily VR at 12 months (69.2 ± 4.5 vs. 61.1 ± 2.1 bpm, P = 0.11) and at 24 months (64.2 ± 1.8 vs. 69.3 ± 1.6 bpm, P = 0.20) were similar between patients with RVA and RVS pacing, respectively.
Radionuclide ventriculography
There were no significant differences between patients with RVA and RVS pacing in the global LVEF (59.6 ± 1.9 vs. 59.5 ± 2.4%, P = 0.98) and the peak ventricular filling rate (2.80 ± 0.15 vs. 2.48 ± 0.13 s −1 , P = 0.12) as measured by the radionuclide ventriculogram at 6 months ( Figure 2 ).
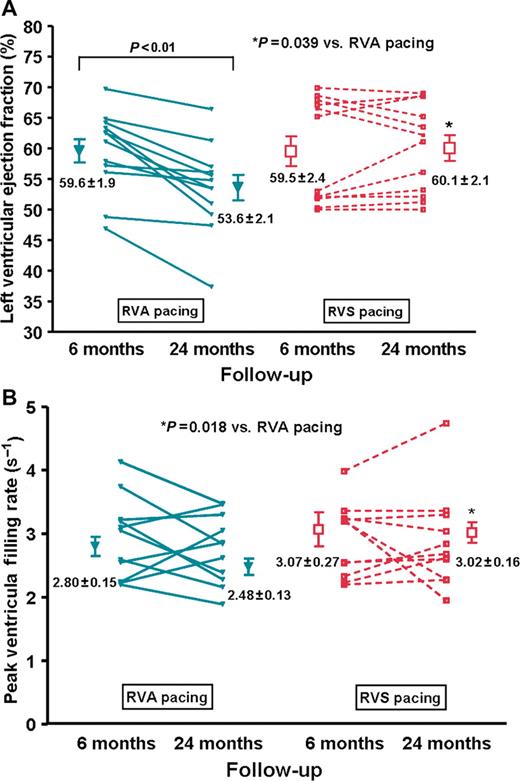
Changes in ( A ) global left ventricular ejection fraction (LVEF) and ( B ) left ventricular diastolic function after 6 and 24 months of right ventricular apical (RVA) and right ventricular septal (RVS) pacing. Data are expressed as mean ± SEM.
In patients with RVA pacing, there was significant interval decrease in the global LVEF at 24 months compared with 6 months data ( Figure 2 A , P = 0.0026). There was no interval change in the global LVEF in patients with RVS pacing ( P > 0.05, Figure 2 A ). Although there was no significant interval change in the peak ventricular filling rate in both groups between 6 and 24 months ( P > 0.05), the peak ventricular filling rate was significantly lower at 24 months in patients with RVA pacing than those with RVS pacing ( Figure 2 B , P = 0.018).
Functional capacity
At 6 months, there was no significant difference in the 6MHW distance between patients with RVA pacing and those with RVS pacing (368.6 ± 12.3 vs. 343.3 ± 16.5 m, P = 0.23, Figure 3 ). In patients with RVA pacing, there was significant interval decrease in 6MHW distance at 24 months compared with 6 months ( Figure 3 , P < 0.05). In contrast, there was a significant increase in 6MHW distance at 24 months compared with 6 months in patients with RVS pacing ( Figure 3 , P < 0.001). Nevertheless, there was no significant correlation between changes in LVEF and 6MWH from baseline to 24 months ( r = 0.26, P = 0.45).
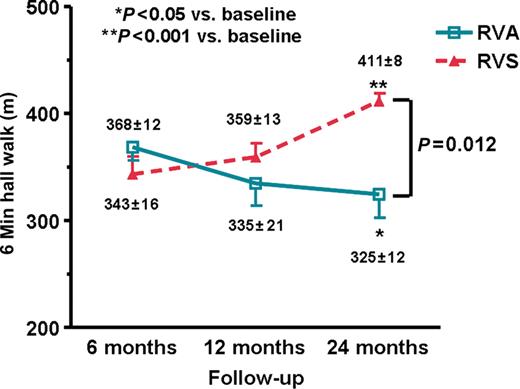
Changes in the 6 min hall walk distance in patients with right ventricular apical (RVA, n = 12) and right ventricular septal (RVS, n = 12) pacing at 6, 12, and 24 months of follow-up. Data are expressed as mean ± SEM. * P -values determined by ANOVA with Bonferroni multiple comparison tests.
Discussion
Main findings
The present study examines the impact of VRR with different RV pacing sites in patients with permanent AF. In this study, a dynamic ventricular overdrive pacing algorithm was used to provide more effective VRR in ambulatory patients due to constant change of physiological conditions and, consequently, the mean VR during AF. 17 In patients with permanent AF and bradycardia who require pacemaker implantation, the use of a VRR algorithm with RVS pacing, but not RVA pacing, improved exercise capacity and preserved LVEF during follow-up when compared with baseline.
Our findings confirm that the VRR algorithm significantly reduced beat-to-beat VR irregularity by increasing the percentage of ventricular pacing without any significant changes in the mean VR in patients with permanent AF. The efficacy of VRR algorithm was similar in patients with RVA pacing and RVS pacing. The long-term use of the VRR algorithm in patients with RVA pacing was nonetheless associated with progressive deterioration in global LV function and functional capacity. In contrast, long-term RVS pacing preserved global LV systolic and diastolic function and improved exercise capacity during follow-up.
Effects of ventricular rate regularization during atrial fibrillation
In patients with AF, both the rate and the irregularity of ventricular response contribute to symptoms and lead to impaired exercise capacity. Prior experimental 11 and clinical studies 12 have shown that an irregular ventricular response, independent of rate, impairs cardiac haemodynamic performance. In this study, the use of the VRR algorithm to increase the percentage of ventricular pacing significantly reduced the VR variability as measured by device diagnostic counter. Consistent with the result of recent studies, 24 pacing at RVA or at a site closer proximity to the AV node had a similar effect on VRR. This finding suggests that the transit time of antrograde and retrograde impulse during ventricular pacing did not have major impact on the VR stabilization effect during AF.
Although acute haemodynamic studies show that ventricular overdrive pacing improves cardiac performance in patients with AF, 11–14 clinical studies with different ventricular overdrive algorithms in implantable pacemaker demonstrate only modest or no significant improvement in symptoms, quality of life, and functional capacity. 15–17 Similarly, VR control and regularization by AV nodal ablation and pacemaker implantation also fails to improve exercise capacity and quality of life in patients with AF compared with rate-control only with AV nodal blocking agents. 25 It is possible that the potential benefit of VRR by pacing may be masked by the deleterious effect of permanent RVA pacing. 18–20 , 26 The results of this study demonstrate no beneficial effects of VRR algorithm for VRR on exercise capacity in patients with RVA pacing.
Impact of ventricular pacing sites
Emerging evidence shows that RVA pacing leads to abnormalities of ventricular activation and contraction, and impairs myocardial perfusion with adverse LV remodelling and consequent increased risk of cardiac morbidity and mortality. 18 , 19 , 27 There is thus growing interest to minimize unnecessary RVA pacing and to preserve normal ventricular activation with alternative ventricular pacing sites. 28 , 29 As shown in this study, the use of a ventricular overdrive pacing algorithm during AF can significantly increase the percentage of ventricular pacing by 38%. Although the use of a VRR algorithm did not significantly increase the overall mean VR, the peak exercise heart rate was significantly increased due to the overdrive pacing of shorter intrinsic RR interval during exercise. This increase in the percentage of RVA pacing might offset any potential benefit of VRR during AF with VRR algorithm.
Experimental 30 , 31 and clinical studies 20–23 suggest that RVS pacing may lead to a lesser degree of abnormal LV electrical and mechanical activation, and can potentially avoid the adverse effects on LV function related to permanent pacing as observed with RVA pacing. In this study, a significant reduction in pacing QRS duration was achieved during RVS pacing by positioning the lead to that particular site on the interventricular septum compared with RVA pacing, confirming the results of previous reports. 20 , 21 After 6 months of backup pacing, there were no significant differences in the global LV systolic function and exercise capacity between RVA and RVS pacing. However, RVS pacing with VRR algorithm in patients with AF preserved global LVEF and improved exercise capacity as determined by 6MWH after 18 months of follow-up. In contrast, there was significant interval decrease in global LVEF (∼10%) and exercise capacity (∼12%) during RVA pacing with VRR algorithm after 18 months. These data confirm our previous findings 20 that decreased asynchronous ventricular activation with RVS pacing preserves global LV systolic and diastolic function during permanent pacing and provides a synergistic effect with VRR using dynamic ventricular overdrive pacing during AF to improve exercise capacity.
In this study, there was only a small difference in LVEF as measured by radionuclide ventriculogram between RVA and RVS group at 24 months. However, the difference in 6MHW between the two groups was much larger. Furthermore, there was no significant correlation between changes in LVEF and 6MHW. Although the reason for this discrepancy remains unclear, it is possible that the impact of different sites of RV pacing on cardiac performance may be greater during exercise as the percentage of ventricular pacing increases with VRR algorithm. Therefore, the potential functional benefit of RVS pacing over RVA pacing is greater during exercise than at rest.
Study limitations
First, patients with significant structural heart disease and/or impaired LVEF at baseline were excluded in this study as ventricular overdrive pacing can be potentially harmful in such patients. Whether the findings of this study are applicable to these patients thus remains unclear. Second, the number of patients in this study was small. This was due to the strict screening criteria applied in order to exclude other factors that might affect LV function. Furthermore, three patients from each group were excluded from the analysis as they could not complete the initial assessment of LVEF and functional capacity before VRR ON. Therefore, the results of this study should be interpreted with cautions. Third, the potential benefit of RVS pacing without VRR was not addressed in this study.
Conclusions
The results of this study provide useful information regarding the selection of ventricular pacing site to optimize VR control and regularization in patients with permanent AF. The use of VRR at RVS, but not at RVA, to regularize VR during AF preserved LVEF and provides incremental benefit for exercise capacity in patients with permanent AF in whom satisfactory VR control was achieved with AV nodal blocking agents.
Conflict of interest: none declared.