-
PDF
- Split View
-
Views
-
Cite
Cite
Maziar Arfaee, Annemijn Vis, Jolanda Kluin, Future technologies in total artificial heart development: can a robot become as good as a donor heart?, European Heart Journal, Volume 43, Issue 48, 21 December 2022, Pages 4970–4972, https://doi.org/10.1093/eurheartj/ehac512
- Share Icon Share
How soft total artificial hearts may overcome the limitations of their rigid counterparts?
Heart failure is a growing cardiovascular disease epidemic worldwide. Despite improvements in treating patients with heart failure, still many patients develop end-stage heart disease and require hospitalization, treatments with high complication rates and risk a premature death. Heart transplantation is the preferred treatment of end-stage heart failure, but there is a significant shortage of donor hearts. For this reason, researchers have been trying for decades to find an implantable mechanical pump that can take over the function of the human heart; a total artificial heart (TAH).1
Typically, a TAH is a rigid mechanical device that has two blood chambers and is actuated by a moving membrane that pushes out the blood. In 1969, the first TAH implantation in humans was performed by Denton Cooley (Texas Heart Institute, Houston, TX, USA). The TAH supported the patient for 64 h until a donor heart was available. The patient died 32 h later of sepsis, haemolysis and renal impairment caused by the poor biocompatibility of the TAH.2 Since the first implantation of a TAH in man, a lot has been improved. Nowadays, there are two membrane type TAHs available for human implantation (SynCardia and Carmat).3,4 The SynCardia TAH has been implanted in over 1700 patients worldwide.3 Also, other experimental approaches are currently being Investigated; such as the development of continuous flow TAHs. However, after almost seven decades of research, no suitable TAH has been found to truly overcome the shortage of donor hearts.1 This raises the question whether it would be possible at all, to develop a TAH that solves the current issues related to poor biocompatibility, high complication rates and low quality of life, in order to become a destination therapy for patients with end-stage heart failure.
Biocompatibility of current TAH devices remains a major challenge. Biocompatibility not only refers to the inner surface of the TAH that is in direct contact with blood. The fact that the blood is propelled in a ‘rigid’, non-physiological way is probably an even more important aspect of the low biocompatibility of current devices. Not surprisingly, thromboembolic complications are amongst the most frequently reported causes of death of TAH recipients. Furthermore, the percutaneous hoses that current TAHs need, increase the risk for infections and lower the quality of life.
Soft robotics is an emerging field of research that aims to develop robots with high adaptability to the environment and to humans. Their benefits include low manufacturing cost, safe human-machine interaction,5 light-weight, and ease of process. Soft robots are also favourable for resembling biological systems because of their softness, compliance, flexibility, and high adaptability to the environment. In fact, many soft actuators are inspired by natural organs and their motions, which makes them more adaptive, safer, and more desirable in situations in which the robot interacts with humans.6,7 However, the development of soft robots is still in its infancy, and it is not known if soft robots are indeed safe and durable. In comparison to their rigid counterparts for example, soft robots are still unable to perform very precise tasks and their durability is still limited.8
Although, we hypothesize that a soft robotic TAH can mimic the natural behaviour of the human heart in many aspects, promoting a level of biocompatibility that cannot be obtained with rigid TAHs.
The properties of the elastomeric materials typically used for soft robotic devices, enable us to resemble native heart behaviour in a more natural way. The first application of a soft robotic device used as cardiac assists device was published by Roche et al.9 The device is a soft robotic sleeve that consists of a configuration of soft pneumatic actuators that are placed in a sock and wrapped around the failing heart. This sleeve is able to assist the heart in both the contraction and relaxation phase, a unique feature that could be beneficial to TAH application as well.
A key feature of the human heart is its compliance that affects and improves its performance. Considering the compliance of soft materials, we can design embodied-intelligent structures to overcome the challenges that are currently faced in TAH development, such as a preload dependent cardiac output and maintenance of balanced outputs to the systemic and pulmonary circulation.
Regarding the actuation of a soft robotic TAH, Van Laake et al.10 recently developed a control system for fluidic-driven soft actuators using a soft valve that converts continuous flow to pulsatile flow. It can be used to programme and actuate any soft robot that needs sequential actuation such as a walking robot, but also a soft TAH. This showcases the feasibility of developing soft robots that operate autonomously by using almost no electronics.
Although the soft robotic cardiac sleeve9 and the soft robotic heart11 are still in early developmental stages, these new technologies pave the way for further development of soft robotic TAHs. The field of soft robotics is rapidly evolving and the design of smart soft materials will enhance the future development of soft robotic hearts that can morphologically adapt to physiological changes and can contribute in both the relaxation as well as the actuation phase of the heart.
Upcoming solutions to further enhance biocompatibility can be found in biomaterial science and tissue engineering. The key difference with tissue engineering an entire heart is that in a soft robotic TAH, only one layer of endothelium is required. The ‘muscle’ power is generated by the soft robotic actuators.
To answer the question from the beginning: can a robot become as good as a donor heart? We are not there yet, but the future has a lot in mind. We can speculate that the next generation of TAHs may be soft, mimic the natural motion and have living tissue on the valves and ventricles. To think another step further, recent improvements in battery technologies have boosted the research on transcutaneous energy transfer systems, that can power the TAH across the skin without the need for percutaneous drivelines. Given these developments, a soft robotic TAH as destination therapy might become reality (Figure 1).
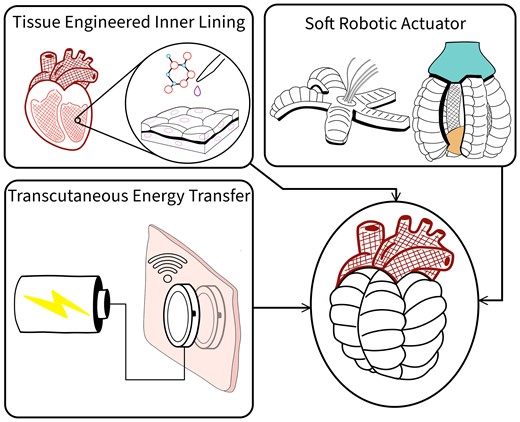
The future of TAHs: A TAH made of soft robotic actuators and biocompatible materials with tissue engineered inner lining, powered by a transcutaneous energy transfer system.
Funding
This work is funded by the European Union Horizon 2020 research and innovation programme under grant agreement no. 767195.
Data availability
Data sharing not applicable to this article as no datasets were generated or analysed during the current study.
References
Author notes
Conflict of interest: All authors are part of the HybridHeart consortium and declare no competing interests.