-
PDF
- Split View
-
Views
-
Cite
Cite
Rosa D Wouda, S Matthijs Boekholdt, Kay Tee Khaw, Nicholas J Wareham, Martin H de Borst, Ewout J Hoorn, Joris I Rotmans, Liffert Vogt, Sex-specific associations between potassium intake, blood pressure, and cardiovascular outcomes: the EPIC-Norfolk study, European Heart Journal, Volume 43, Issue 30, 7 August 2022, Pages 2867–2875, https://doi.org/10.1093/eurheartj/ehac313
- Share Icon Share
Abstract
A potassium replete diet is associated with lower blood pressure (BP) and lower risk of cardiovascular disease (CVD). Whether these associations differ between men and women and whether they depend on daily sodium intake is unknown.
An analysis was performed in 11 267 men and 13 696 women from the EPIC-Norfolk cohort. Twenty-four hour excretion of sodium and potassium, reflecting intake, was estimated from sodium and potassium concentration in spot urine samples using the Kawasaki formula. Linear and Cox regression were used to explore the association between potassium intake, systolic BP (SBP), and CVD events (defined as hospitalization or death due to CVD). After adjustment for confounders, interaction by sex was found for the association between potassium intake and SBP (P < 0.001). In women, but not in men, the inverse slope between potassium intake and SBP was steeper in those within the highest tertile of sodium intake compared with those within the lowest tertile of sodium intake (P < 0.001 for interaction by sodium intake). Both in men and women, higher potassium intake was associated with a lower risk of CVD events, but the hazard ratio (HR) associated with higher potassium intake was lower in women than in men [highest vs. lowest potassium intake tertile: men: HR 0.93, 95% confidence interval (CI) 0.87–1.00; women: HR 0.89, 95% CI 0.83–0.95, P = 0.033 for interaction by sex].
The association between potassium intake, SBP, and CVD events is sex specific. The data suggest that women with a high sodium intake in particular benefit most from a higher potassium intake with regard to SBP.
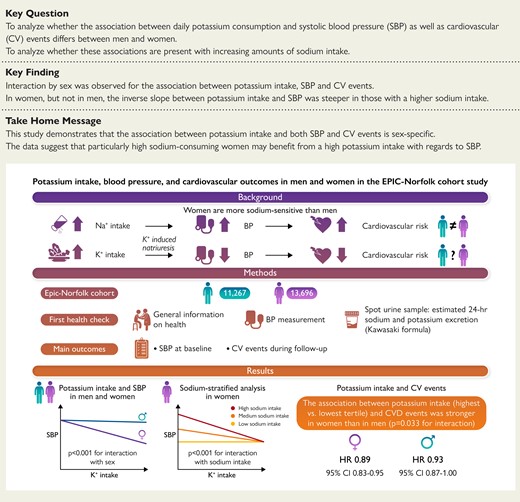
An analysis was performed in 11 267 men and 13 696 women from the EPIC-Norfolk cohort. Twenty-four hour excretion of sodium and potassium, reflecting intake, was estimated from sodium and potassium concentration in spot urine samples using the Kawasaki formula. Linear and Cox regression analyses showed that the association between potassium intake and both SBP and CVD risk is sex specific. Moreover, among women, the association between potassium intake and SBP was modified by sodium intake. EPIC-Norfolk, Norfolk cohort of the European Prospective Investigation into Cancer; CVD, cardiovascular disease; SBP, systolic blood pressure.
See the editorial comment for this article ‘Sutton's law and dietary Na+/K+ intake in cardiovascular disease’, by Franz H. Messerli et al., https://doi.org/10.1093/eurheartj/ehac283.
Introduction
Cardiovascular disease (CVD) is the leading cause of death worldwide.1 The incidence of CVD is high in both men and women, but sex-specific differences exist.2,3 Differences between men and women in cardiovascular risk factors and treatments have been studied extensively,4–6 but little attention has been paid to the sex-specific effects of sodium and potassium consumption with respect to cardiovascular outcomes.
Dietary patterns in the Western world are characterized by a high sodium and low potassium content.7 There is a well-established association between high sodium consumption, blood pressure (BP), and an increased risk of cardiovascular events.8,9 In contrast to the harmful effects of high sodium consumption, a potassium replete diet may have a beneficial effect on cardiovascular health. Multiple cohort studies have shown that high urinary potassium excretion, as proxy for dietary potassium intake, is related to a lower BP and reduced risk of CVD.8,10–13
The cardiovascular protection associated with higher potassium consumption is generally attributed to its BP-lowering effects through promoting natriuresis and normalization of the extracellular fluid status.14–16 These effects seem to depend on the amount of sodium consumption, at least in healthy volunteers, in whom a three-fold higher natriuresis in response to an oral potassium load was observed during a high sodium, as compared with a low sodium diet.17 Furthermore, a large global cohort study including >100 000 subjects demonstrated that the lower BP associated with a higher potassium intake was augmented in persons with higher levels of sodium consumption.8 It is unknown whether sex-specific differences in the potassium intake-associated cardiovascular protection exist. Also, in view of various studies showing that women are more sodium-sensitive—i.e. having a larger change in BP in response to changes in sodium consumption18–20—women with high sodium intake might specifically benefit more from an increase in potassium intake. Sex-specific data on the relationship between potassium intake and long-term cardiovascular outcomes are however lacking. Therefore, the aim of this study was to analyze whether the associations between daily potassium consumption and BP as well as CVD differ between men and women from the EPIC-Norfolk population-based cohort, and whether these associations depend on daily sodium consumption. We hypothesize that (i) the inverse associations between potassium consumption and systolic BP (SBP) as well as CVD is stronger in women than in men and (ii) that these associations are amplified in women with a high daily sodium consumption.
Methods
Study design and participants
We performed an analysis in the Norfolk cohort of the European Prospective Investigation into Cancer (EPIC-Norfolk) study, a prospective population-based cohort study. The design and characteristics of the EPIC-Norfolk study have been described previously.21 A total of 25 639 participants of 40–79 years old were recruited from registries of general practices in Norfolk (UK) between 1993 and 1997. All participants signed informed consent. The EPIC-Norfolk study was approved by the Norwich District Health Authority Ethics Committee.
Study procedures
During the first health check, general information on health and lifestyle was collected using a questionnaire, a physical examination was performed, blood was drawn, and a random spot urine sample was collected. The health checks were carried out by trained study nurses. The health and lifestyle questionnaire contained questions about the participant’s medical history, medication use, alcohol use, and smoking status. The physical examination consisted of measurements of height, weight, and BP. BP was measured twice using an Accutorr sphygmomanometer (Datascope, UK) after the participant had been in a seated position for 5 min. The mean of two measurements was used for statistical analysis. Blood samples were stored at 4°C and assayed at the Department of Clinical Biochemistry, University of Cambridge, Cambridge, UK. Serum creatinine concentration was measured by standard laboratory methods on an Olympus AU640. Spot urine samples were stored, without preservation, at −20°C until further analysis. Between 1998 and 2002, spot urine sodium and potassium concentrations were assayed by flame photometry (IL 943; Instrumentation Lab, Warrington, UK) and creatinine concentrations were determined on a Roche Cobas Mira Plus analyser. Glomerular filtration rate (GFR) was estimated using the Chronic Kidney Disease Epidemiology Collaboration formula.22
To estimate 24-h potassium and sodium excretion from spot urine samples, we used the Kawasaki formula (see Supplementary material online, Item S1).23 Compared with other formulae that estimate 24-h excretion of potassium and sodium from a single spot urine sample (the Tanaka and INTERSALT formula), the highest interclass correlation between estimated and measured 24-h sodium and potassium excretion was observed for the Kawasaki formula.24–26 After application of the Kawasaki formula, we excluded extreme outliers of estimated 24-h potassium and sodium excretion [more than Q3 + 3 × interquartile range (IQR) or less than Q1 – 3 × IQR]. Estimated 24-h urine potassium and sodium excretion were considered to reflect daily intake. Actual daily potassium and sodium consumption are expected to be higher than 24-h urine potassium and sodium excretion, due to excretion of sodium and potassium through sweat and faeces.
Outcomes of interest
The main outcomes were SBP at baseline (i.e. the day of spot urine collection) and the composite of hospitalization or mortality from CVD. Other outcomes of interest were diastolic BP (DBP), mean arterial pressure (MAP), the composite of hospitalization or mortality from ischaemic heart disease (IHD), and the composite of hospitalization or mortality from ischaemic or haemorrhagic stroke. Hospitalization during follow-up was determined by linkage of the participant’s National Health Service number with the East Norfolk Health Authority (ENCORE) database. Vital status was recorded by the UK Office for National Statistics. Cardiovascular disease was defined if the underlying cause of hospitalization or mortality was coded as hypertensive disease, IHD, heart failure, aortic aneurysm, or cerebrovascular disease (ICD-10 codes I10-I79). Outcome events were recorded until 31 March 2016.
Statistical analysis
For the current analysis, we excluded patients with missing data for sex, age, height, weight, and spot urine sodium, potassium, and creatinine concentration. Continuous data are presented as mean with standard deviation or in case of non-normal distribution as median with IQR. The distribution of data was visually inspected using histograms. Categorical data are presented as frequency and percentage. Since potassium and sodium intake, as well as cardiovascular risk2,3 differ for men and women, baseline characteristics were presented for men and women separately. Differences in baseline characteristics between men and women were compared using unpaired t-tests for continuous variables and χ2 tests for categorical variables.
We performed a multivariable linear regression analysis stratified by sex to determine the association between potassium intake (g/day) and SBP. Since SBP is modified by the use of antihypertensive medication, we estimated the untreated SBP by adding a constant to the measured SBP in participants using antihypertensive medication.27 Based on a meta-analysis of the average reduction in BP produced by BP-lowering drugs, 15 mmHg was added to the treated SBP.28 The final model was adjusted for age and sodium intake (tertiles). Considering the imprecision of estimated potassium intake, we repeated the analysis using tertiles of potassium intake. Due to differences in potassium and sodium intake between men and women, sex-specific tertiles of potassium and sodium intake were defined. To explore the association between potassium intake and cardiovascular events, we used a Cox proportional hazards model stratified by sex. For this analysis, the lowest tertile of potassium intake was regarded as the reference group. In the final model, adjustments were made for age, body mass index, sodium intake (tertiles), smoking status (never smoked, former smoker, current smoker), use of lipid-lowering drugs, alcohol use (g/day), a history of diabetes mellitus, and a history of CVD (stroke and/or myocardial infarction). The proportionality of hazards was evaluated using formal statistical tests and plots of the scaled Schoenfeld residuals. Skewed variables were log transformed before entering the regression models.
Before conducting the sex-stratified linear and Cox regression analyses, interaction by sex for the association between potassium intake and SBP, and CVD events was formally tested using an interaction term. Because of the sex-specific estimation of the 24-h urine creatinine in the Kawasaki formula that may influence results, we also tested for interaction by sex for the association between spot urine sodium-to-potassium ratio and SBP, and CVD events. The spot urine sodium-to-potassium ratio does not include urine creatinine and, as such, will not be affected by sex-specific estimation of 24-h creatinine.
To further explore the effect of sodium intake on the association between potassium intake and SBP and CVD events in men and women, we tested for interaction by sodium intake (highest vs. lowest tertile), and performed linear and Cox regression analyses stratified by sodium intake tertile. In order to perform these analyses, we generated six strata for sodium intake (men: tertile 1, tertile 2, and tertile 3; women: tertile 1, tertile 2, and tertile 3).
Separate sensitivity and subgroup analyses were conducted by excluding participants with and without hypertension (defined as mean BP > 140/90 mmHg and/or use of antihypertensive medication at baseline), chronic kidney disease (CKD, defined as eGFR < 60 mL/min/1.73 m2 at baseline), prevalent CVD (i.e. a history myocardial infarction or stroke), and prevalent diabetes mellitus, respectively. Moreover, to assess the influence of hormone replacement therapy (HRT), sensitivity analyses were performed in women not using HRT. Lastly, to explore whether associations remained present when using another formula for estimating 24-h excretion of potassium and sodium, linear and Cox regression analyses were repeated with estimated 24-h potassium and sodium excretion based on the Tanaka formula.26
Regression coefficients and hazard ratios (HRs) are presented with a 95% confidence interval (CI). Statistical analyses were conducted in R (version 3.6.1) with the addition of the survival package (3.2–3). Figures were made in R and Graphpad Prism (version 8). A P-value of <0.05 was considered statistically significant.
Results
Baseline characteristics
We included a cohort of 24 963 participants, 11 267 men and 13 696 women (see Supplementary material online, Figure S1). The mean age was 59 ± 9 years for men and 58 ± 9 years for women. 18.5% of men and 18.6% of women used antihypertensive medication. For men, estimated potassium intake was 71 ± 16 mmol/day (2.8 ± 0.6 g/day) and estimated sodium intake 214 ± 62 mmol/day (4.9 ± 1.4 g/day), whereas for women estimated potassium intake was 66 ± 16 mmol/day (2.6 ± 0.6 g/day) and estimated sodium intake 183 ± 61 mmol/day (4.2 ± 1.4 g/day) (Table 1). Mean daily sodium and potassium intake within each tertile are presented in Table 1. Men and women within the highest tertile of potassium intake were on average younger, had a higher BMI, lower SBP, and higher sodium intake compared with men and women within the lowest tertile of potassium intake (see Supplementary material online, Table S1). Spot urine sodium-to-potassium ratio had a skewed distribution (see Supplementary material online, Figure S2). The median spot urine sodium-to-potassium concentration was 1.7 ± 1.3 in men and 1.5 ± 1.2 in women. Both in men and in women a highly significant correlation between potassium and sodium intake was observed (R = 0.44, P < 0.001; R = 0.48, P < 0.001, respectively) (see Supplementary material online, Figure S3).
Characteristic . | Men (n = 11 267) . | Women (n = 13 696) . | P-value . |
---|---|---|---|
European descent | 11 165 (99.6) | 13 607 (99.7) | 0.037 |
Age at baseline (years) | 59 ± 9 | 58 ± 9 | <0.001 |
BMI (kg/m2) | 26.5 ± 3.3 | 26.2 ± 4.3 | <0.001 |
Blood pressure (mmHg) | |||
ȃSystolic | 137 ± 18 | 134 ± 19 | <0.001 |
ȃDiastolic | 84 ± 11 | 81 ± 11 | <0.001 |
Spot urine sodium (mmol/L)b | 90 ± 71 | 64 ± 61 | <0.001 |
Spot urine potassium (mmol/L)b | 55 ± 45 | 46 ± 45 | <0.001 |
Sodium-to-potassium ratiob | 1.7 ± 1.3 | 1.5 ± 1.2 | <0.001 |
Sodium intake [mmol/day (g/day)]a | 214 ± 62 (4.9 ± 1.4) | 183 ± 61 (4.2 ± 1.4) | <0.001 |
ȃTertile 1 | 150 ± 28 (3.5 ± 0.7) | 121 ± 26 (2.8 ± 0.6) | <0.001 |
ȃTertile 2 | 211 ± 14 (4.8 ± 0.3) | 179 ± 14 (4.1 ± 0.3) | <0.001 |
ȃTertile 3 | 283 ± 41 (6.5 ± 0.9) | 251 ± 43 (5.8 ± 1.0) | <0.001 |
Potassium intake [mmol/day (g/day)]a | 71 ± 16 (2.8 ± 0.6) | 66 ± 16 (2.6 ± 0.6) | <0.001 |
ȃTertile 1 | 55 ± 6 (2.2 ± 0.2) | 49 ± 6 (1.9 ± 0.2) | <0.001 |
ȃTertile 2 | 70 ± 4 (2.7 ± 0.1) | 64 ± 4 (2.5 ± 0.1) | <0.001 |
ȃTertile 3 | 89 ± 11 (3.5 ± 0.4) | 84 ± 12 (3.3 ± 0.5) | <0.001 |
eGFR CKD-EPI (mL/min/1.73 m2) | 77 ± 16 | 72 ± 16 | <0.001 |
Self-reported diabetes | 347 (3.1) | 210 (1.5) | <0.001 |
Self-reported myocardial infarction | 591 (5.3) | 180 (1.3) | <0.001 |
Self-reported stroke | 193 (1.7) | 139 (1.0) | <0.001 |
Use of antihypertensive medication | 2086 (18.5) | 2542 (18.6) | 0.939 |
Use of lipid-lowering drugs | 170 (1.5) | 200 (1.5) | 0.792 |
Alcohol use (g/day)b | 6.8 (13.4) | 2.0 (7.7) | <0.001 |
Smoking status—no. (%) | <0.001 | ||
ȃNever smoked | 3747 (33.5) | 7647 (56.4) | |
ȃFormer smoker | 6089 (54.4) | 4366 (32.2) | |
ȃCurrent smoker | 1352 (12.1) | 1547 (11.4) |
Characteristic . | Men (n = 11 267) . | Women (n = 13 696) . | P-value . |
---|---|---|---|
European descent | 11 165 (99.6) | 13 607 (99.7) | 0.037 |
Age at baseline (years) | 59 ± 9 | 58 ± 9 | <0.001 |
BMI (kg/m2) | 26.5 ± 3.3 | 26.2 ± 4.3 | <0.001 |
Blood pressure (mmHg) | |||
ȃSystolic | 137 ± 18 | 134 ± 19 | <0.001 |
ȃDiastolic | 84 ± 11 | 81 ± 11 | <0.001 |
Spot urine sodium (mmol/L)b | 90 ± 71 | 64 ± 61 | <0.001 |
Spot urine potassium (mmol/L)b | 55 ± 45 | 46 ± 45 | <0.001 |
Sodium-to-potassium ratiob | 1.7 ± 1.3 | 1.5 ± 1.2 | <0.001 |
Sodium intake [mmol/day (g/day)]a | 214 ± 62 (4.9 ± 1.4) | 183 ± 61 (4.2 ± 1.4) | <0.001 |
ȃTertile 1 | 150 ± 28 (3.5 ± 0.7) | 121 ± 26 (2.8 ± 0.6) | <0.001 |
ȃTertile 2 | 211 ± 14 (4.8 ± 0.3) | 179 ± 14 (4.1 ± 0.3) | <0.001 |
ȃTertile 3 | 283 ± 41 (6.5 ± 0.9) | 251 ± 43 (5.8 ± 1.0) | <0.001 |
Potassium intake [mmol/day (g/day)]a | 71 ± 16 (2.8 ± 0.6) | 66 ± 16 (2.6 ± 0.6) | <0.001 |
ȃTertile 1 | 55 ± 6 (2.2 ± 0.2) | 49 ± 6 (1.9 ± 0.2) | <0.001 |
ȃTertile 2 | 70 ± 4 (2.7 ± 0.1) | 64 ± 4 (2.5 ± 0.1) | <0.001 |
ȃTertile 3 | 89 ± 11 (3.5 ± 0.4) | 84 ± 12 (3.3 ± 0.5) | <0.001 |
eGFR CKD-EPI (mL/min/1.73 m2) | 77 ± 16 | 72 ± 16 | <0.001 |
Self-reported diabetes | 347 (3.1) | 210 (1.5) | <0.001 |
Self-reported myocardial infarction | 591 (5.3) | 180 (1.3) | <0.001 |
Self-reported stroke | 193 (1.7) | 139 (1.0) | <0.001 |
Use of antihypertensive medication | 2086 (18.5) | 2542 (18.6) | 0.939 |
Use of lipid-lowering drugs | 170 (1.5) | 200 (1.5) | 0.792 |
Alcohol use (g/day)b | 6.8 (13.4) | 2.0 (7.7) | <0.001 |
Smoking status—no. (%) | <0.001 | ||
ȃNever smoked | 3747 (33.5) | 7647 (56.4) | |
ȃFormer smoker | 6089 (54.4) | 4366 (32.2) | |
ȃCurrent smoker | 1352 (12.1) | 1547 (11.4) |
Differences in baseline characteristics between men and women were compared by χ2 test for categorical variables and unpaired t-test for continuous variables (after log transformation in case of a skewed distribution). BMI, body mass index; eGFR, estimated glomerular filtration rate. Data are given as n (%) and mean ± SD.
Sodium and potassium intake were estimated from a single urine sample on basis of the Kawasaki formula.
Data are given as median ± IQR.
Characteristic . | Men (n = 11 267) . | Women (n = 13 696) . | P-value . |
---|---|---|---|
European descent | 11 165 (99.6) | 13 607 (99.7) | 0.037 |
Age at baseline (years) | 59 ± 9 | 58 ± 9 | <0.001 |
BMI (kg/m2) | 26.5 ± 3.3 | 26.2 ± 4.3 | <0.001 |
Blood pressure (mmHg) | |||
ȃSystolic | 137 ± 18 | 134 ± 19 | <0.001 |
ȃDiastolic | 84 ± 11 | 81 ± 11 | <0.001 |
Spot urine sodium (mmol/L)b | 90 ± 71 | 64 ± 61 | <0.001 |
Spot urine potassium (mmol/L)b | 55 ± 45 | 46 ± 45 | <0.001 |
Sodium-to-potassium ratiob | 1.7 ± 1.3 | 1.5 ± 1.2 | <0.001 |
Sodium intake [mmol/day (g/day)]a | 214 ± 62 (4.9 ± 1.4) | 183 ± 61 (4.2 ± 1.4) | <0.001 |
ȃTertile 1 | 150 ± 28 (3.5 ± 0.7) | 121 ± 26 (2.8 ± 0.6) | <0.001 |
ȃTertile 2 | 211 ± 14 (4.8 ± 0.3) | 179 ± 14 (4.1 ± 0.3) | <0.001 |
ȃTertile 3 | 283 ± 41 (6.5 ± 0.9) | 251 ± 43 (5.8 ± 1.0) | <0.001 |
Potassium intake [mmol/day (g/day)]a | 71 ± 16 (2.8 ± 0.6) | 66 ± 16 (2.6 ± 0.6) | <0.001 |
ȃTertile 1 | 55 ± 6 (2.2 ± 0.2) | 49 ± 6 (1.9 ± 0.2) | <0.001 |
ȃTertile 2 | 70 ± 4 (2.7 ± 0.1) | 64 ± 4 (2.5 ± 0.1) | <0.001 |
ȃTertile 3 | 89 ± 11 (3.5 ± 0.4) | 84 ± 12 (3.3 ± 0.5) | <0.001 |
eGFR CKD-EPI (mL/min/1.73 m2) | 77 ± 16 | 72 ± 16 | <0.001 |
Self-reported diabetes | 347 (3.1) | 210 (1.5) | <0.001 |
Self-reported myocardial infarction | 591 (5.3) | 180 (1.3) | <0.001 |
Self-reported stroke | 193 (1.7) | 139 (1.0) | <0.001 |
Use of antihypertensive medication | 2086 (18.5) | 2542 (18.6) | 0.939 |
Use of lipid-lowering drugs | 170 (1.5) | 200 (1.5) | 0.792 |
Alcohol use (g/day)b | 6.8 (13.4) | 2.0 (7.7) | <0.001 |
Smoking status—no. (%) | <0.001 | ||
ȃNever smoked | 3747 (33.5) | 7647 (56.4) | |
ȃFormer smoker | 6089 (54.4) | 4366 (32.2) | |
ȃCurrent smoker | 1352 (12.1) | 1547 (11.4) |
Characteristic . | Men (n = 11 267) . | Women (n = 13 696) . | P-value . |
---|---|---|---|
European descent | 11 165 (99.6) | 13 607 (99.7) | 0.037 |
Age at baseline (years) | 59 ± 9 | 58 ± 9 | <0.001 |
BMI (kg/m2) | 26.5 ± 3.3 | 26.2 ± 4.3 | <0.001 |
Blood pressure (mmHg) | |||
ȃSystolic | 137 ± 18 | 134 ± 19 | <0.001 |
ȃDiastolic | 84 ± 11 | 81 ± 11 | <0.001 |
Spot urine sodium (mmol/L)b | 90 ± 71 | 64 ± 61 | <0.001 |
Spot urine potassium (mmol/L)b | 55 ± 45 | 46 ± 45 | <0.001 |
Sodium-to-potassium ratiob | 1.7 ± 1.3 | 1.5 ± 1.2 | <0.001 |
Sodium intake [mmol/day (g/day)]a | 214 ± 62 (4.9 ± 1.4) | 183 ± 61 (4.2 ± 1.4) | <0.001 |
ȃTertile 1 | 150 ± 28 (3.5 ± 0.7) | 121 ± 26 (2.8 ± 0.6) | <0.001 |
ȃTertile 2 | 211 ± 14 (4.8 ± 0.3) | 179 ± 14 (4.1 ± 0.3) | <0.001 |
ȃTertile 3 | 283 ± 41 (6.5 ± 0.9) | 251 ± 43 (5.8 ± 1.0) | <0.001 |
Potassium intake [mmol/day (g/day)]a | 71 ± 16 (2.8 ± 0.6) | 66 ± 16 (2.6 ± 0.6) | <0.001 |
ȃTertile 1 | 55 ± 6 (2.2 ± 0.2) | 49 ± 6 (1.9 ± 0.2) | <0.001 |
ȃTertile 2 | 70 ± 4 (2.7 ± 0.1) | 64 ± 4 (2.5 ± 0.1) | <0.001 |
ȃTertile 3 | 89 ± 11 (3.5 ± 0.4) | 84 ± 12 (3.3 ± 0.5) | <0.001 |
eGFR CKD-EPI (mL/min/1.73 m2) | 77 ± 16 | 72 ± 16 | <0.001 |
Self-reported diabetes | 347 (3.1) | 210 (1.5) | <0.001 |
Self-reported myocardial infarction | 591 (5.3) | 180 (1.3) | <0.001 |
Self-reported stroke | 193 (1.7) | 139 (1.0) | <0.001 |
Use of antihypertensive medication | 2086 (18.5) | 2542 (18.6) | 0.939 |
Use of lipid-lowering drugs | 170 (1.5) | 200 (1.5) | 0.792 |
Alcohol use (g/day)b | 6.8 (13.4) | 2.0 (7.7) | <0.001 |
Smoking status—no. (%) | <0.001 | ||
ȃNever smoked | 3747 (33.5) | 7647 (56.4) | |
ȃFormer smoker | 6089 (54.4) | 4366 (32.2) | |
ȃCurrent smoker | 1352 (12.1) | 1547 (11.4) |
Differences in baseline characteristics between men and women were compared by χ2 test for categorical variables and unpaired t-test for continuous variables (after log transformation in case of a skewed distribution). BMI, body mass index; eGFR, estimated glomerular filtration rate. Data are given as n (%) and mean ± SD.
Sodium and potassium intake were estimated from a single urine sample on basis of the Kawasaki formula.
Data are given as median ± IQR.
Systolic blood pressure
After adjustments for sex, age and sodium intake (tertiles), potassium intake was significantly associated with SBP (β = −0.8, P < 0.001), and we found significant interaction by sex (P < 0.001; Table 2 and Figure 1). Consequently, the relation between potassium intake and SBP differs between men and women. Likewise, interaction by sex was present for the associations between potassium intake and DBP, and MAP (see Supplementary material online, Tables S2 and S3). Interaction between log spot urine sodium-to-potassium ratio and sex was significant as well for the outcome SBP (P = 0.019) (see Supplementary material online, Figure S4).
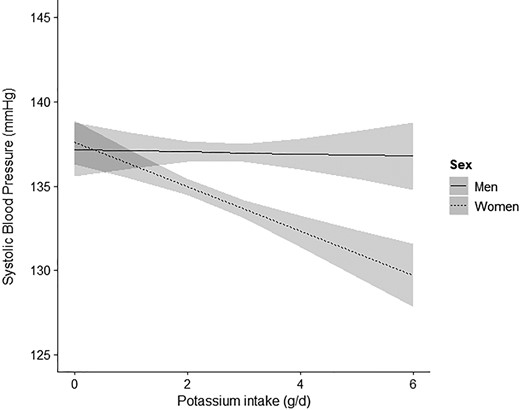
Interaction by sex for the association between potassium intake (g/day) and systolic blood pressure. Linear regression analysis for the association between potassium intake (g/day) and SBP with interaction by sex, adjusted for age, and sodium intake (tertiles). Interaction by sex was significant (P < 0.001). In men no association was observed between potassium intake (g/day) and SBP (P = 0.336), whereas in women this association was highly significant (P < 0.001). SBP, systolic blood pressure. The grey area represents the 95% confidence interval.
. | Systolic blood pressure . | |||
---|---|---|---|---|
Potassium intake (g/day) . | β . | 95% . | CI . | P-value . |
A Total cohort | ||||
Model 1 | −0.4 | −0.8 | 0.0 | 0.034 |
Model 2 | 1.0 | 0.6 | 1.3 | <0.001 |
Model 3 | −0.8 | −1.2 | −0.4 | <0.001 |
Model 4 | −1.3 | −1.8 | −0.8 | <0.001 |
B Men | ||||
Model 1 | −1.0 | −1.6 | −0.4 | <0.001 |
Model 2 | 1.2 | 0.7 | 1.8 | <0.001 |
Model 3 | −0.3 | −0.9 | 0.3 | 0.336 |
Model 4 | −0.3 | −1.4 | 0.8 | 0.634 |
C Women | ||||
Model 1 | −0.9 | −1.4 | −0.3 | 0.002 |
Model 2 | 0.6 | 0.1 | 1.1 | 0.012 |
Model 3 | −1.3 | −1.8 | −0.7 | <0.001 |
Model 4 | 0.3 | −0.7 | 1.4 | 0.535 |
. | Systolic blood pressure . | |||
---|---|---|---|---|
Potassium intake (g/day) . | β . | 95% . | CI . | P-value . |
A Total cohort | ||||
Model 1 | −0.4 | −0.8 | 0.0 | 0.034 |
Model 2 | 1.0 | 0.6 | 1.3 | <0.001 |
Model 3 | −0.8 | −1.2 | −0.4 | <0.001 |
Model 4 | −1.3 | −1.8 | −0.8 | <0.001 |
B Men | ||||
Model 1 | −1.0 | −1.6 | −0.4 | <0.001 |
Model 2 | 1.2 | 0.7 | 1.8 | <0.001 |
Model 3 | −0.3 | −0.9 | 0.3 | 0.336 |
Model 4 | −0.3 | −1.4 | 0.8 | 0.634 |
C Women | ||||
Model 1 | −0.9 | −1.4 | −0.3 | 0.002 |
Model 2 | 0.6 | 0.1 | 1.1 | 0.012 |
Model 3 | −1.3 | −1.8 | −0.7 | <0.001 |
Model 4 | 0.3 | −0.7 | 1.4 | 0.535 |
Linear regression model. A Model 1: unadjusted analysis. Model 2: adjusted for sex and age. Model 3: adjusted for sex, age, and sodium intake (tertiles). Model 4: Model 3 including interaction by sex. In the total cohort, interaction by sex for the association between potassium intake and SBP was significant (model: P < 0.001). B and C Model 1: unadjusted analysis. Model 2: adjusted for age. Model 3: adjusted for age and sodium intake (tertiles). Model 4: Model 3 including interaction by sodium intake. In men, no interaction by sodium intake (highest vs. lowest tertile) for the association between potassium intake and SBP was present (P = 0.481). In women, interaction by sodium intake (highest vs. lowest tertile) for the association between potassium intake and SBP was highly significant (P < 0.001). SBP, systolic blood pressure; CI, confidence interval.
. | Systolic blood pressure . | |||
---|---|---|---|---|
Potassium intake (g/day) . | β . | 95% . | CI . | P-value . |
A Total cohort | ||||
Model 1 | −0.4 | −0.8 | 0.0 | 0.034 |
Model 2 | 1.0 | 0.6 | 1.3 | <0.001 |
Model 3 | −0.8 | −1.2 | −0.4 | <0.001 |
Model 4 | −1.3 | −1.8 | −0.8 | <0.001 |
B Men | ||||
Model 1 | −1.0 | −1.6 | −0.4 | <0.001 |
Model 2 | 1.2 | 0.7 | 1.8 | <0.001 |
Model 3 | −0.3 | −0.9 | 0.3 | 0.336 |
Model 4 | −0.3 | −1.4 | 0.8 | 0.634 |
C Women | ||||
Model 1 | −0.9 | −1.4 | −0.3 | 0.002 |
Model 2 | 0.6 | 0.1 | 1.1 | 0.012 |
Model 3 | −1.3 | −1.8 | −0.7 | <0.001 |
Model 4 | 0.3 | −0.7 | 1.4 | 0.535 |
. | Systolic blood pressure . | |||
---|---|---|---|---|
Potassium intake (g/day) . | β . | 95% . | CI . | P-value . |
A Total cohort | ||||
Model 1 | −0.4 | −0.8 | 0.0 | 0.034 |
Model 2 | 1.0 | 0.6 | 1.3 | <0.001 |
Model 3 | −0.8 | −1.2 | −0.4 | <0.001 |
Model 4 | −1.3 | −1.8 | −0.8 | <0.001 |
B Men | ||||
Model 1 | −1.0 | −1.6 | −0.4 | <0.001 |
Model 2 | 1.2 | 0.7 | 1.8 | <0.001 |
Model 3 | −0.3 | −0.9 | 0.3 | 0.336 |
Model 4 | −0.3 | −1.4 | 0.8 | 0.634 |
C Women | ||||
Model 1 | −0.9 | −1.4 | −0.3 | 0.002 |
Model 2 | 0.6 | 0.1 | 1.1 | 0.012 |
Model 3 | −1.3 | −1.8 | −0.7 | <0.001 |
Model 4 | 0.3 | −0.7 | 1.4 | 0.535 |
Linear regression model. A Model 1: unadjusted analysis. Model 2: adjusted for sex and age. Model 3: adjusted for sex, age, and sodium intake (tertiles). Model 4: Model 3 including interaction by sex. In the total cohort, interaction by sex for the association between potassium intake and SBP was significant (model: P < 0.001). B and C Model 1: unadjusted analysis. Model 2: adjusted for age. Model 3: adjusted for age and sodium intake (tertiles). Model 4: Model 3 including interaction by sodium intake. In men, no interaction by sodium intake (highest vs. lowest tertile) for the association between potassium intake and SBP was present (P = 0.481). In women, interaction by sodium intake (highest vs. lowest tertile) for the association between potassium intake and SBP was highly significant (P < 0.001). SBP, systolic blood pressure; CI, confidence interval.
In men, neither an inverse association between potassium intake and SBP was present (β = −0.3, P = 0.336), nor interaction by sodium intake (highest vs. lowest tertile) (P = 0.481) (Table 2). Conversely, in women, a highly significant interaction by sodium intake (highest vs. lowest tertile) for the association between potassium intake and SBP was present (P < 0.001) (Table 2). Sodium-stratified analyses indicated that an inverse association between potassium intake and SBP was only present in the highest tertile of sodium intake (tertile 3: β = −2.4, P < 0.001) (Figure 2). Following from this, in women within the highest tertile of sodium intake, every 1 g increase in daily potassium intake was associated with a 2.4 mmHg lower SBP.

Association between potassium intake (g/day) and systolic blood pressure in men and women within every tertile of sodium intake. Sodium-stratified linear regression analyses for the association between potassium intake (g/day) and SBP in men and women, adjusted for age. Interaction by sodium intake (highest vs. lowest tertile) was significant in women (P < 0.001), but not in men (P = 0.481). In women within the highest tertile of sodium intake a strong inverse association between potassium intake and SBP was present (β = −2.4, P < 0.001). SBP, systolic blood pressure; CI, confidence interval.
The interaction by sodium intake (highest vs. lowest tertile) for the association between potassium intake (highest vs. lowest tertile) and SBP was also observed in women when potassium intake was analyzed in tertiles (P = 0.004). Similarly, the association between potassium intake (highest vs. lowest tertile) was stronger in women within the highest tertile of sodium intake compared with those within the lowest tertile of sodium intake (see Supplementary material online, Figure S5).
Sensitivity analyses after exclusion of people with a history of diabetes mellitus or CVD, people using antihypertensive medication (see Supplementary material online, Table S4), and women using HRT, showed essentially similar results. Moreover, when adjusting for the use of antihypertensive medication, instead of adding a constant to the treated SBP, and when adjusting for alcohol use associations remained present (see Supplementary material online, Tables S5 and S6). Also, when estimates of potassium and sodium intake were based on the Tanaka formula a significant interaction by sodium intake (highest vs. lowest tertile) for the association between potassium intake and SBP was present in women, but not in men (data not shown). Yet, after exclusion of women with either hypertension or CKD at baseline, interaction between potassium and sodium intake (highest vs. lowest tertile) with regard to SBP was attenuated and no longer statistically significant (women without hypertension: P = 0.094 for interaction between potassium and sodium intake; women without CKD: P = 0.077 for interaction between potassium and sodium intake). Conversely, in women with hypertension, interaction by sodium tertile (highest vs. lowest tertile) for the association between potassium and SBP was significant (P = 0.004 for interaction between potassium and sodium intake). In women with CKD, the slope of the association between potassium intake and SBP was highly heterogeneous and no significant interaction was present between potassium and sodium intake (P = 0.096 for interaction) (see Supplementary material online, Figure S6).
Cardiovascular outcomes
During a median follow-up time of 19.5 years, CVD events occurred in 13 596 (54.5%) participants, including 6705 (59.5%) CVD events in men and 6891 (50.3%) CVD events in women. In the fully adjusted model, people within the highest tertile of potassium intake had a lower risk of CVD events (HR 0.87, 95% CI 0.82–0.93) compared with those within the lowest tertile of potassium intake. Moreover, we found significant interaction by sex for the association between potassium intake (highest vs. lowest tertile) and CVD events (P = 0.033) (Table 3). Similarly, interaction by sex was observed for the association between potassium intake (g/day) and CVD events (P = 0.012, see Supplementary material online, Figure S7), and spot urine sodium-to-potassium ratio and CVD events (P = 0.022) (data not shown). Interaction by sex was present as well for the association between potassium intake (highest vs. lowest tertile) and IHD events (P = 0.016, see Supplementary material online, Table S7). For ischaemic stroke events, interaction between potassium intake (intermediate vs. lowest tertile) and sex was significant (P = 0.027), but not for haemorrhagic stroke events (see Supplementary material online, Table S8).
. | CVD events . | ||||
---|---|---|---|---|---|
. | HR . | HR (95% CI) . | P-value . | HR (95% CI) . | P-value . |
Potassium intake . | Tertile 1 . | Tertile 2 . | Tertile 3 . | ||
A Total cohort | |||||
CVD events, n (%) | 4760 (57.2) | 4633 (55.7) | 4203 (50.5) | ||
Model 1 | 1.00 | 0.91 (0.87–0.95) | <0.001 | 0.79 (0.75–0.82) | <0.001 |
Model 2 | 1.00 | 0.98 (0.94–1.02) | 0.232 | 0.95 (0.91–0.99) | 0.008 |
Model 3 | 1.00 | 0.95 (0.91–1.00) | 0.031 | 0.91 (0.87–0.96) | <0.001 |
Model 4 | 1.00 | 0.95 (0.90–1.01) | 0.093 | 0.87 (0.82–0.93) | <0.001 |
B Men | |||||
CVD events, n (%) | 2367 (63.0) | 2266 (60.3) | 2072 (55.2) | ||
Model 1 | 1.00 | 0.87 (0.82–0.92) | <0.001 | 0.76 (0.71–0.80) | <0.001 |
Model 2 | 1.00 | 0.98 (0.93–1.04) | 0.500 | 0.98 (0.92–1.04) | 0.435 |
Model 3 | 1.00 | 0.95 (0.89–1.01) | 0.097 | 0.93 (0.87–1.00) | 0.044 |
C Women | |||||
CVD events, n (%) | 2393 (52.4) | 2367 (51.9) | 2131 (46.7) | ||
Model 1 | 1.00 | 0.94 (0.89–1.00) | 0.034 | 0.81 (0.76–0.86) | <0.001 |
Model 2 | 1.00 | 0.97 (0.92–1.03) | 0.288 | 0.91 (0.86–0.97) | 0.002 |
Model 3 | 1.00 | 0.96 (0.90–1.02) | 0.141 | 0.89 (0.83–0.95) | <0.001 |
. | CVD events . | ||||
---|---|---|---|---|---|
. | HR . | HR (95% CI) . | P-value . | HR (95% CI) . | P-value . |
Potassium intake . | Tertile 1 . | Tertile 2 . | Tertile 3 . | ||
A Total cohort | |||||
CVD events, n (%) | 4760 (57.2) | 4633 (55.7) | 4203 (50.5) | ||
Model 1 | 1.00 | 0.91 (0.87–0.95) | <0.001 | 0.79 (0.75–0.82) | <0.001 |
Model 2 | 1.00 | 0.98 (0.94–1.02) | 0.232 | 0.95 (0.91–0.99) | 0.008 |
Model 3 | 1.00 | 0.95 (0.91–1.00) | 0.031 | 0.91 (0.87–0.96) | <0.001 |
Model 4 | 1.00 | 0.95 (0.90–1.01) | 0.093 | 0.87 (0.82–0.93) | <0.001 |
B Men | |||||
CVD events, n (%) | 2367 (63.0) | 2266 (60.3) | 2072 (55.2) | ||
Model 1 | 1.00 | 0.87 (0.82–0.92) | <0.001 | 0.76 (0.71–0.80) | <0.001 |
Model 2 | 1.00 | 0.98 (0.93–1.04) | 0.500 | 0.98 (0.92–1.04) | 0.435 |
Model 3 | 1.00 | 0.95 (0.89–1.01) | 0.097 | 0.93 (0.87–1.00) | 0.044 |
C Women | |||||
CVD events, n (%) | 2393 (52.4) | 2367 (51.9) | 2131 (46.7) | ||
Model 1 | 1.00 | 0.94 (0.89–1.00) | 0.034 | 0.81 (0.76–0.86) | <0.001 |
Model 2 | 1.00 | 0.97 (0.92–1.03) | 0.288 | 0.91 (0.86–0.97) | 0.002 |
Model 3 | 1.00 | 0.96 (0.90–1.02) | 0.141 | 0.89 (0.83–0.95) | <0.001 |
Cox proportional hazard model. A Model 1: unadjusted analysis. Model 2: adjusted for age and sex. Model 3: adjusted for age, sex, BMI, sodium intake (tertiles), use of lipid-lowering drugs, smoking status, alcohol use, diabetes mellitus, and history of CVD. Model 4: Model 3 including interaction by sex. The lowest tertile of potassium intake was regarded as reference. In the total cohort, interaction by sex for the association between potassium intake (highest vs. lowest tertile) and CVD events was significant (P = 0.033). B and C Model 1: unadjusted analysis. Model 2: adjusted for age. Model 3: adjusted for age, BMI, sodium intake (tertiles), use of lipid-lowering drugs, smoking status, alcohol use, diabetes mellitus, and history of CVD. In both men and women, no interaction by sodium intake (highest vs. lowest tertile) for the association between potassium intake (highest vs. lowest tertile) and CVD events was present (men: P = 0.611; women: P = 0.527). CVD, cardiovascular disease; CI, confidence interval.
. | CVD events . | ||||
---|---|---|---|---|---|
. | HR . | HR (95% CI) . | P-value . | HR (95% CI) . | P-value . |
Potassium intake . | Tertile 1 . | Tertile 2 . | Tertile 3 . | ||
A Total cohort | |||||
CVD events, n (%) | 4760 (57.2) | 4633 (55.7) | 4203 (50.5) | ||
Model 1 | 1.00 | 0.91 (0.87–0.95) | <0.001 | 0.79 (0.75–0.82) | <0.001 |
Model 2 | 1.00 | 0.98 (0.94–1.02) | 0.232 | 0.95 (0.91–0.99) | 0.008 |
Model 3 | 1.00 | 0.95 (0.91–1.00) | 0.031 | 0.91 (0.87–0.96) | <0.001 |
Model 4 | 1.00 | 0.95 (0.90–1.01) | 0.093 | 0.87 (0.82–0.93) | <0.001 |
B Men | |||||
CVD events, n (%) | 2367 (63.0) | 2266 (60.3) | 2072 (55.2) | ||
Model 1 | 1.00 | 0.87 (0.82–0.92) | <0.001 | 0.76 (0.71–0.80) | <0.001 |
Model 2 | 1.00 | 0.98 (0.93–1.04) | 0.500 | 0.98 (0.92–1.04) | 0.435 |
Model 3 | 1.00 | 0.95 (0.89–1.01) | 0.097 | 0.93 (0.87–1.00) | 0.044 |
C Women | |||||
CVD events, n (%) | 2393 (52.4) | 2367 (51.9) | 2131 (46.7) | ||
Model 1 | 1.00 | 0.94 (0.89–1.00) | 0.034 | 0.81 (0.76–0.86) | <0.001 |
Model 2 | 1.00 | 0.97 (0.92–1.03) | 0.288 | 0.91 (0.86–0.97) | 0.002 |
Model 3 | 1.00 | 0.96 (0.90–1.02) | 0.141 | 0.89 (0.83–0.95) | <0.001 |
. | CVD events . | ||||
---|---|---|---|---|---|
. | HR . | HR (95% CI) . | P-value . | HR (95% CI) . | P-value . |
Potassium intake . | Tertile 1 . | Tertile 2 . | Tertile 3 . | ||
A Total cohort | |||||
CVD events, n (%) | 4760 (57.2) | 4633 (55.7) | 4203 (50.5) | ||
Model 1 | 1.00 | 0.91 (0.87–0.95) | <0.001 | 0.79 (0.75–0.82) | <0.001 |
Model 2 | 1.00 | 0.98 (0.94–1.02) | 0.232 | 0.95 (0.91–0.99) | 0.008 |
Model 3 | 1.00 | 0.95 (0.91–1.00) | 0.031 | 0.91 (0.87–0.96) | <0.001 |
Model 4 | 1.00 | 0.95 (0.90–1.01) | 0.093 | 0.87 (0.82–0.93) | <0.001 |
B Men | |||||
CVD events, n (%) | 2367 (63.0) | 2266 (60.3) | 2072 (55.2) | ||
Model 1 | 1.00 | 0.87 (0.82–0.92) | <0.001 | 0.76 (0.71–0.80) | <0.001 |
Model 2 | 1.00 | 0.98 (0.93–1.04) | 0.500 | 0.98 (0.92–1.04) | 0.435 |
Model 3 | 1.00 | 0.95 (0.89–1.01) | 0.097 | 0.93 (0.87–1.00) | 0.044 |
C Women | |||||
CVD events, n (%) | 2393 (52.4) | 2367 (51.9) | 2131 (46.7) | ||
Model 1 | 1.00 | 0.94 (0.89–1.00) | 0.034 | 0.81 (0.76–0.86) | <0.001 |
Model 2 | 1.00 | 0.97 (0.92–1.03) | 0.288 | 0.91 (0.86–0.97) | 0.002 |
Model 3 | 1.00 | 0.96 (0.90–1.02) | 0.141 | 0.89 (0.83–0.95) | <0.001 |
Cox proportional hazard model. A Model 1: unadjusted analysis. Model 2: adjusted for age and sex. Model 3: adjusted for age, sex, BMI, sodium intake (tertiles), use of lipid-lowering drugs, smoking status, alcohol use, diabetes mellitus, and history of CVD. Model 4: Model 3 including interaction by sex. The lowest tertile of potassium intake was regarded as reference. In the total cohort, interaction by sex for the association between potassium intake (highest vs. lowest tertile) and CVD events was significant (P = 0.033). B and C Model 1: unadjusted analysis. Model 2: adjusted for age. Model 3: adjusted for age, BMI, sodium intake (tertiles), use of lipid-lowering drugs, smoking status, alcohol use, diabetes mellitus, and history of CVD. In both men and women, no interaction by sodium intake (highest vs. lowest tertile) for the association between potassium intake (highest vs. lowest tertile) and CVD events was present (men: P = 0.611; women: P = 0.527). CVD, cardiovascular disease; CI, confidence interval.
Both men and women within the highest tertile of potassium intake had a lower risk of CVD events compared with those within the lowest tertile of potassium intake, but the HR associated with higher potassium intake was lower in women than in men (fully adjusted model: men: HR 0.93, 95% CI 0.87–1.00; women: HR 0.89, 95% CI 0.83–0.95) (Table 3). No interaction by sodium intake (highest vs. lowest tertile) for the association between potassium intake (highest vs. lowest tertile) and CVD events was present in both men and women (men: P = 0.611; women: P = 0.527). Supplementary material online, Figure S8 shows the results of the sodium-stratified analyses.
Results were essentially similar when estimates of potassium and sodium intake were based on the Tanaka formula. Moreover, the exclusion of participants with an outcome event within the first 3 years of follow-up did not materially change results (see Supplementary material online, Table S9). Also, in men and women without a history of diabetes mellitus, CVD, hypertension, and CKD, and women not using HRT associations remained present (data not shown).
Discussion
In this analysis of the population-based EPIC-Norfolk cohort, we demonstrate that the association between potassium consumption and both SBP and CVD risk is sex specific. Moreover, among women, the association between potassium intake and SBP was modified by sodium intake (Structured Graphical Abstract). Specifically, an inverse relation between potassium intake and SBP was only present in women within the highest tertile of sodium intake. With regard to long-term outcomes, women within the highest tertile of potassium intake had a respectively 11% lower risk of incident and/or recurrent CVD events during follow-up of almost 20 years, compared with women within the lowest tertile of potassium intake. In men, the risk of CVD events was 7% lower for those within the highest tertile of potassium intake compared with those within the lowest tertile of potassium intake. Both in men and in women, the association between potassium intake and CVD events was not modified by sodium intake.
The inverse association between urinary potassium excretion, reflecting daily potassium consumption, and BP is in line with previous observational and interventional studies in both healthy and hypertensive people.8,29–31 The association between potassium consumption and lower CVD event rates has also been confirmed by other cohort studies.11–13 However, the effect of sodium consumption on these associations has not been studied in detail. Previous cohort and interventional studies have explored the effect of sodium intake on the potentially BP-lowering effect of potassium. Yet, sex-specific effects were not evaluated. Results from the INTERMAP study suggested that the association between potassium consumption and BP was reduced in participants with lower levels of sodium excretion.32 Similar associations were observed in the PURE study, showing that the potassium-associated BP differences were larger in persons with increased levels of estimated sodium excretion.8 In addition, a recent meta-analysis of randomized controlled trials assessing the relationship between potassium supplementation and BP, indicated that participants with a high sodium intake might benefit more from a high potassium diet.33 Our analysis now adds the insight that sex-specific differences exist in the association between potassium excretion and both SBP and CVD events, and that in women the association between potassium intake and SBP depends on the amount of sodium excretion.
Based on experimental and controlled intervention studies, we hypothesize that the beneficial effects of potassium may be attributed to increased potassium-mediated natriuresis and subsequent correction of a hypervolemic state. High potassium intake results in dephosphorylation of the thiazide-sensitive sodium-chloride cotransporter (NCC) in the distal convoluted tubule, thereby reducing the activity of this transporter and causing a net increase in sodium excretion.34,35 Interestingly, the expression of the NCC is sex dependent. In rats, the density of the NCC was higher in female rats than in male rats.36,37 Moreover, ovariectomy in female rats decreased the expression and phosphorylation of the NCC, suggesting that female sex hormones are involved in the regulation of the activity of the NCC.38 Yet, given the mean age in our cohort, most women were presumably postmenopausal, indicating that other mechanisms leading to sodium sensitivity may also play a role. Results from experimental studies indicate that an unbalance between nitric oxide and angiotensin II, resulting in oxidative stress, increased sodium reabsorption, and decreased pressure natriuresis, caused sodium sensitivity in ovariectomized rats.39,40 The difference in sodium sensitivity between men and women may explain, at least in part, the sex-specific associations between potassium intake and SBP and CVD events. In addition to potential sex differences in sodium sensitivity, hypertension and CKD also lead to sodium sensitivity,41,42 which may explain the attenuated interaction between potassium and sodium intake after excluding women with hypertension and CKD at baseline.
Thus, the lower risk of CVD events associated with high potassium intake may in part be linked to the natriuretic effect of potassium and subsequent BP lowering. However, considering the lack of interaction with daily sodium intake in our study, other cardiovascular protective effects of potassium may be involved as well. These effects presumably relate to the antioxidant and vasoactive properties of potassium. Animal studies indicate that potassium supplementation protects against vascular injury, vascular calcification, and stiffness, and improves left ventricular function during high sodium conditions.43–45 Human studies have demonstrated that the addition of potassium to a sodium-rich meal diminishes the sodium-induced post-prandial reduction in flow-mediated dilatation.46 These observations indicate that potassium might play an important role in cardiovascular health beyond its natriuretic effects, explaining the sodium-independent inverse association between potassium intake and CVD events.
There are some strengths and limitations to the present analysis. Strengths of this analysis include the large study population and the long-term follow-up (19.5 years), resulting in a large number of events in both men and women. In addition, the consistency of our results was assessed by using different models and performing multiple sensitivity analyses. An important limitation of this study is that only one random spot urine sample was collected for estimation of 24-h excretion of sodium and potassium by using the Kawasaki formula. Nevertheless, results from 24-h urine samples collected by a subgroup of this cohort (n = 340) indicated that there was a good agreement between average measured 24-h urine sodium and potassium excretion and average 24-h urine sodium and potassium excretion estimated from a random urine sample.47 Since the Kawasaki formula was originally validated in a fasting morning sample (second-morning void),23 we also used the Tanaka formula to assess daily sodium and potassium intake.26 Results from the linear and Cox regression analyses in men and women were essentially similar for both formulas. Since estimated daily potassium excretion may differ considerably from actual intake,48 we pooled the estimations of potassium and sodium excretion into tertiles to explore the association with long-term cardiovascular outcomes. By doing so, we reduced the effect of outliers, imprecise estimations, misclassification of high vs. low consumers, and day-to-day variations in potassium and sodium excretion. Our previous studies showed that single urine samples correctly estimate average daily sodium and potassium excretion in a large sample of people,13,49,50 and therefore adequately detect the direction of associations between potassium excretion and health outcomes in observational studies. In addition to that, sex-specific associations were also present between spot urine sodium-to-potassium ratio, SBP, and CVD events. In contrast to estimated 24-h sodium and potassium excretion, the sodium-to-potassium ratio does not depend on urine creatinine and therefore is not affected by sex-specific estimation of 24-h urine creatinine. Furthermore, the estimated sodium intake was higher than usually can be expected.51 Although these amounts of sodium consumption are in line with other large cohort studies,52–54 different results might be found in populations that are characterized by overall lower sodium intakes. Moreover, dietary habits may change over the years, which may attenuate the observed associations. Nevertheless, to prevent the biased classification of the level of sodium consumption, again our analyses on estimated sodium consumption were also performed using tertiles. Another limitation regarding sensitivity analyses is that only one measurement of eGFR was used to define CKD, which might overestimate the incidence of CKD.55 Lastly, a high potassium consumption may reflect a better diet quality and/or nutritional status. Although, we adjusted for many potential confounding variables, we cannot exclude residual confounding.
In conclusion, we demonstrate that the association between daily potassium intake, SBP, and CVD events is sex specific. In women, the association between potassium intake and SBP was modified by sodium intake. Considering the natriuretic effects of potassium, these findings seem consistent with increased sodium sensitivity of BP in women. The stronger association between potassium intake and SBP in women seems also relevant for long-term CV outcomes, as our study shows that the inverse association between potassium intake and CVD events was stronger in women than in men. Our observations may therefore have impact on the differences in cardiovascular morbidity and mortality between men and women and underline the beneficial effects of a potassium replete diet.
Supplementary material
Supplementary material is available at European Heart Journal online.
Acknowledgements
We are grateful to all the participants who have been part of the project and to the many members of the study teams at the University of Cambridge who have enabled this research.
Funding
The EPIC-Norfolk study (DOI 10.22025/2019.10.105.00004) has received funding from the Medical Research Council (MR/N003284/1 MC-UU_12015/1 and MC_UU_00006/1) and Cancer Research UK (C864/A14136). L.V., J.I.R., E.J.H., M.H.d.B., and R.D.W. have received funding from the Dutch Kidney Foundation (K+onsortium, grant CP1601).
References
Author notes
Conflict of interest: None declared.