-
PDF
- Split View
-
Views
-
Cite
Cite
Filippo Crea, New challenges in vascular biology and medicine: from unravelling the mechanisms of neointima formation to the prevention of amputations and of ischaemic stroke, European Heart Journal, Volume 42, Issue 18, 7 May 2021, Pages 1715–1719, https://doi.org/10.1093/eurheartj/ehab224
- Share Icon Share
For the podcast associated with this article, please visit https://dbpia.nl.go.kr/eurheartj/pages/Podcasts.
This Focus Issue on vascular biology and medicine contains a clinical research article entitled ‘The association of amputations and peripheral artery disease in patients with type 2 diabetes mellitus receiving sodium–glucose co-transporter type-2 inhibitors: real-world study’, authored by Sanjoy Paul from the University of Melbourne in Australia, and colleagues.1 Patients with peripheral artery disease (PAD) remain a challenging population to treat, in particular in the attempt to reduce the risk of amputation.2–4 Paul et al. evaluated the temporal pattern of amputations in type 2 diabetes (T2DM) patients, the risk of amputations by new and older antidiabetic drugs (ADDs), and the interplay of PAD with therapy and amputation risk. Using Centricity Electronic Medical Records from the USA, ∼3 300 000 patients with T2DM were identified. The proportion of incident amputations per 10 000 adults ranged between 4.7 and 6.8 during 2000–2008 and significantly increased to 12.3 in 2017. Patients with pre-existing PAD had a more than four-fold higher risk of lower limb amputation (LLA). In propensity score-adjusted pair-wise analyses, the risk of LLA was similar in sodium–glucose co-transporter type-2 inhibitors (SGLT-2is) vs. glucagon-like peptide 1 receptor agonists (GLP1-RAs), and lower in SGLT-2i vs. dipeptidyl peptidase-4 inhibitor (DPP-4i) or other ADDs (hazard ratio 0.65 and 0.43, respectively) (Figure 1). The rate of LLA was similar in patients treated with canagliflozin, empagliflozin, or dapagliflozin.
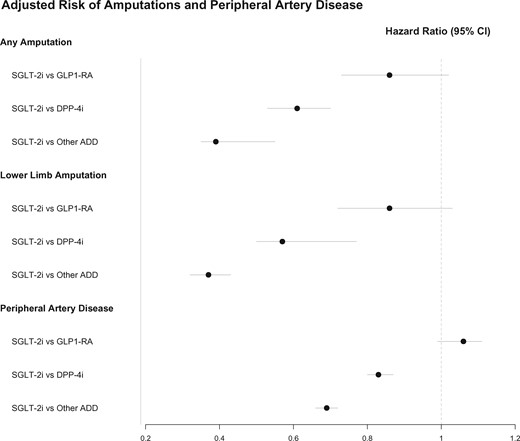
Adjusted risk of amputations and peripheral artery disease (from Paul SK, Bhatt DL, Montvida O. The association of amputations and peripheral artery disease in patients with type 2 diabetes mellitus receiving sodium–glucose co-transporter type-2 inhibitors: real-world study. See pages 1728–1738).
The authors conclude that the risk of amputation in patients treated with SGLT-2is and incretins is not higher compared with other ADDs. In addition, and not surprisingly, pre-existing PAD is the greatest driver of amputation risk. The manuscript is accompanied by an Editorial by Charalambos Vlachopoulos from the University of Athens Medical School in Greece, and colleagues.5 The authors conclude that a considerable number of original studies and analyses have been applied on the canvas of the risk of amputation by SGLT2is that as a whole reduce the contrast of the first randomized trials. While any risk appears to be related specifically to canagliflozin, recent large registries provide reassuring data on the safety of SGLT2is, as long as physicians are aware of this particular complication and monitor their patients closely. Undoubtedly, we are in need of more data, and the pursuit for proper evaluation of canagliflozin calls for ‘making haste slowly’.
Inflammation plays an important role in development of cardiovascular disease (CVD).6–8 The NOD-like receptor protein-3 (NLRP3) inflammasome contributes to the development of atherosclerosis in animal models. Components of the NLRP3 inflammasome pathway such as interleukin-1β (IL-1β) can be targeted therapeutically. In a clinical research article entitled ‘Genetically determined NLRP3 inflammasome activation associates with systemic inflammation and cardiovascular mortality’, Stefan Schunk from the Saarland University Hospital in Homburg/Saar, Germany, and colleagues note that associations of genetically determined inflammasome-mediated systemic inflammation with CVD and mortality in humans are unknown.9 The authors explored the association of genetic NLRP3 variants with prevalent CVD and cardiovascular mortality in 538 167 subjects on an individual participant level in an explorative gene-centric approach without performing multiple testing. The functional relevance of the single nucleotide polymorphism (SNP) for NLRP3 inflammasome activation was evaluated in monocyte-enriched peripheral blood mononuclear cells (PBMCs). Genetic analyses identified the highly prevalent intronic NLRP3 variant rs10754555 as affecting NLRP3 gene expression. rs10754555 carriers showed significantly higher C-reactive protein and serum amyloid A plasma levels. Carriers of the G allele showed higher NLRP3 inflammasome activation in isolated human PBMCs. In carriers of the rs10754555 variant, the prevalence of coronary artery disease (CAD) was significantly higher as compared with non-carriers, with a significant interaction between rs10754555 and age. Importantly, rs10754555 carriers had significantly higher risk for cardiovascular mortality during follow-up. Inflammasome inducers (e.g. urate, triglycerides, and ApoC3) modulated the association between rs10754555 and mortality.
The authors conclude that the NLRP3 intronic variant rs10754555 is associated with increased systemic inflammation, inflammasome activation, prevalent CAD, and mortality. This study provides evidence for a substantial role for genetically driven systemic inflammation in cardiovascular disease and highlights the NLRP3 inflammasome as a therapeutic target. The manuscript is accompanied by an Editorial by Christoph J. Binder and Nikolina Papac-Milicevic from the Medical University of Vienna in Austria.10 The authors conclude that the findings of this study provide important evidence for the individual differences in the ability to develop chronic inflammation in the context of metabolic disturbances. This may open up the possibility for more personalized therapeutic approaches by enabling stratification of patients based on their genetically determined inflammatory risk before clinical manifestations occur.
The aim of endovascular stent implantation at the time of coronary angioplasty is to prevent acute vessel closure and chronic negative arterial remodelling in patients affected by coronary disease. However, stents are sensed as a foreign body, leading to immune cell activation, resulting in chronic inflammation and, eventually, in-stent restenosis due to the local proliferation of arterial smooth muscle cells. Mitigating the body’s reaction by improving stent biocompatibility thus represents a major challenge to increase the efficacy of arterial stents and hence the clinical outcome of patients affected by coronary disease.11,12 In a translational research article entitled ‘Coronary stent CD31-mimetic coating favours endothelialization and reduces local inflammation and neointimal development in vivo’, Sergio Diaz-Rodriguez from the Laval University, Québec, Canada, and colleagues evaluate the effect of CD31-mimetic metal stent coating on the in vitro adherence of endothelial cells (ECs) and blood elements, and the in vivo strut coverage and neointimal growth.13 The rapid endothelialization of bare metal stents (BMS) is counterbalanced by inflammation-induced neointimal growth. Drug-eluting stents (DES) prevent leucocyte activation but impair endothelialization, delaying effective device integration into arterial walls. Previously, it has been shown that engaging the vascular CD31 co-receptor is crucial for endothelial and leucocyte homeostasis and arterial healing. Furthermore, it has been shown that a soluble synthetic peptide (known as P8RI) acts like a CD31 agonist. The authors produced cobalt chromium disks and stents coated with a CD31-mimetic peptide through two procedures, plasma amination or dip-coating, both yielding comparable results. They found that CD31-mimetic disks significantly reduced the extent of primary human coronary artery EC and blood platelet/leucocyte activation in vitro. In vivo, CD31-mimetic stent properties were compared with those of DES and BMS by coronarography and microscopy at 7 and 28 days post-implantation in pig coronary arteries (n = 9 stents/group/time point). Seven days post-implantation, only CD31-mimetic struts were fully endothelialized, with no activated platelets/leucocytes. At day 28, neointima development over CD31-mimetic stents was significantly reduced compared with BMS, appearing as a normal arterial media with absence of thrombosis in contrast to DES.
The authors conclude that CD31-mimetic coating favours vascular homeostasis and arterial wall healing, preventing in-stent stenosis and thrombosis. Hence, such coatings seem to improve metal stent biocompatibility. The manuscript is accompanied by an Editorial by Alexandra Lansky from the Yale School of Medicine in New Haven, CT, USA and colleagues.14 The authors conclude that the effect of a CD31-mimetic stent in CAD patients may be blunted due to impaired function of CD31-expressing cells in this patient population. These will be critical benchmarks to more reliably predict whether this breakthrough combination stent technology can provide the incremental safety and effectiveness benefit needed to further advance the management options of our patients with obstructive coronary disease.
In another translational research article entitled ‘A proteomic atlas of the neointima identifies novel druggable targets for preventive therapy’, Thorsten Kessler from the Deutsches Herzzentrum München in Germany, and colleagues sought to investigate the molecular processes underlying neointima formation and to identify new treatment and prevention targets.15 Neointima formation was induced by wire injury in mouse femoral arteries. High-accuracy proteomic measurement of single femoral arteries to a depth of ∼5000 proteins revealed massive proteome remodelling, with more than half of all proteins exhibiting expression differences between injured and non-injured vessels. The authors observed major changes in the composition of the extracellular matrix and cell migration processes. Among the latter, they identified the classical transient receptor potential channel 6 (Trpc6) as driving neointima formation. This was confirmed in an experimental model. Indeed, Trpc6–/– mice presented reduced neointima formation compared with wild-type mice. In addition, activating or repressing TRPC6 in human vascular smooth muscle cells resulted in increased or decreased migratory capacity, respectively. Finally, in a cohort of individuals with angiographic follow-up in >3000 patients, homozygous carriers of a common genetic variant associated with elevated TRPC6 expression were at increased risk of restenosis after coronary stenting (adjusted odds ratio 1.49) during a mean follow-up of 217 days.
The authors conclude that their study provides a proteomic atlas of the healthy and injured arterial wall that can be used to define novel factors for therapeutic targeting. They present TRPC6 as an actionable target to prevent neointima formation secondary to vascular injury and stent implantation. The manuscript is accompanied by an Editorial by Giuseppina Caligiuri from INSERM in Paris and Gregory Franck from the Hôpitaux Universitaires Paris Nord Val-de-Seine in France.16 The authors conclude that further studies are needed in order to specifically address the therapeutic potential of TRCP6 inhibitors in a clinical perspective. If confirmed, a combo device eluting both mTOR inhibitors and TRCP blockers could select the right ‘channels’, affecting the broadest relevant targets and eventually reaching the ‘no-restenosis’ Holy Grail.
‘Embolic stroke of undetermined source’ (ESUS) is used to describe patients with a non-lacunar ischaemic stroke without any identified embolic source from the heart or the arteries supplying the ischaemic territory, or any other apparent cause. In a State of the Art review article entitled ‘Supracardiac atherosclerosis in embolic stroke of undetermined source: the underestimated source’, George Ntaios from the University of Thessaly in Greece, and colleagues note that when the ESUS concept was introduced, covert atrial fibrillation was conceived to be the main underlying cause in the majority of ESUS patients.17 Yet another important embolic source in ESUS is the atherosclerotic plaque in the carotid, vertebrobasilar, and intracranial arteries, or the aortic arch—collectively described as supracardiac atherosclerosis. There is emerging evidence showing that the role of supracardiac atherosclerosis is larger than was initially perceived. Advanced imaging methods are available to identify plaques which carry high embolic risk. The role of novel antithrombotic strategies in these patients needs to be assessed in randomized controlled trials. This review presents the evidence which points towards a major aetiological association between atherosclerotic plaques and ESUS, summarizes the imaging features which may aid in identifying plaques more likely to be associated with ESUS, discusses strategies to reduce the associated stroke risk, and highlights the rationale for future research in this field.
Unlike native LDL, modified LDLs such as oxidized, carbamylated, or acetylated LDLs are not recognized by the native LDL receptor (LDL-R). Rather, modified LDL binds to the lectin-like oxidized LDL receptor-1 (LOX-1).8,18,19 In a State of the Art review article entitled ‘Lectin-like oxidized low-density lipoprotein receptor-1 (LOX-1): a crucial driver of atherosclerotic cardiovascular disease’, Alexander Akhmedov from the University of Zurich in Switzerland, and colleagues note that LOX-1, a scavenger receptor that promotes endothelial dysfunction by inducing proatherogenic signalling and plaque formation via the endothelial uptake of oxidized LDL (oxLDL) and electronegative LDL, contributes to the initiation, progression, and destabilization of atheromatous plaques, eventually leading to the development of myocardial infarction and certain forms of stroke.20 In addition to its expression in endothelial cells, LOX-1 is expressed in macrophages, cardiomyocytes, fibroblasts, dendritic cells, lymphocytes, and neutrophils, further implicating this receptor in multiple aspects of atherosclerotic plaque formation. LOX-1 holds promise as a novel diagnostic and therapeutic target for certain CVDs; therefore, understanding the molecular structure and function of LOX-1 is of critical importance. In this review, the authors highlight the latest scientific findings related to LOX-1, its ligands, and their roles in the broad spectrum of CVDs. They also describe recent findings from basic research, delineate their translational value, and discuss the potential of LOX-1 as a novel target for the prevention, diagnosis, and treatment of related CVDs (Figure 2).
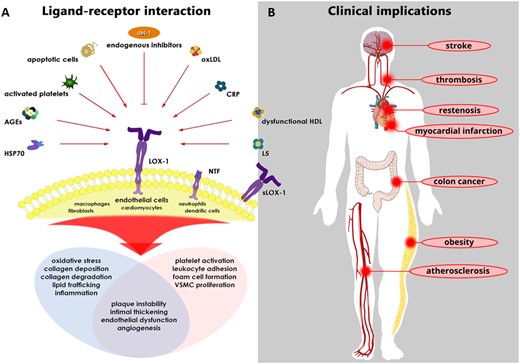
Ligand–receptor interactions (left) and their potential role in various diseases (right). (A) Multiple ligands, including oxLDL, L5, and dysfunctional HDL, have been shown to induce full-length LOX-1 stimulation. Depending on the cell type studied, LOX-1 stimulation activates subcellular signalling pathways that play major roles in the pathogenesis of various cardiovascular diseases. Notably, in human coronary endothelial cells exposed to oxLDL, Del-1 inhibits the LOX-1-dependent up-regulation of endothelial adhesion molecules, thereby representing an interesting endogenous molecule that—at least in part—curtails the detrimental effects mediated by LOX-1. (B) In recent years, the byproduct of LOX-1 cleavage (i.e. sLOX-1) and the most electronegative LDL subfraction (i.e. L5) have emerged as novel biomarkers, specifically in atherosclerosis-related events, such as myocardial infarction and ischaemic stroke. Furthermore, enhanced LAB activity has been shown to be associated with the incidence of cardiovascular diseases, particularly ischaemic stroke. AGEs, advanced glycation end-products; CRP, C-reactive protein; Del-1, developmental endothelial locus-1; HSP70, heat shock protein 70; LAB, LOX-1 ligand containing apoB; LOX-1, lectin-like oxidized LDL receptor-1; L5, L5 LDL; NTF, N-terminal fragment; oxLDL, oxidized LDL; sLOX-1, soluble LOX-1; VSMC, vascular smooth muscle cell (from Akhmedov A, Sawamura T, Chen CH, Kraler S, Vdovenko D, Lüscher TF. Lectin-like oxidized low-density lipoprotein receptor-1 (LOX-1): a crucial driver of atherosclerotic cardiovascular disease. See pages 1797–1807).
The issue is complemented by two Discussion Forum articles: in a contribution entitled ‘Which biomarker to use, when to start, and how to improve adherence for reducing atherosclerotic cardiovascular disease risk?’, Kwang Kon Koh from Gachon University in Korea comments on the contribution ‘2019 vs. 2016 ESC/EAS statin guidelines for primary prevention of atherosclerotic cardiovascular disease’ by Martin Bødtker Mortensen from the Aarhus University Hospital in Denmark, and colleagues.21,22 Mortensen et al. respond in a separate comment.23
The editors hope that readers of this issue of the European Heart Journal will find it of interest.
With thanks to Amelia Meier-Batschelet, Johanna Huggler, and Martin Meyer for help with compilation of this article.
References
Wierer M, Werner J, Wobst J, Kastrati A, Cepele G, Aherrahrou, Sager HB, Erdmann J, Dichgans M, Flockerzi V, Civelek M, Dietrich A, Mann M, Schunkert H, Kessler T.