-
PDF
- Split View
-
Views
-
Cite
Cite
Giulia Angelini, Davide Flego, Ramona Vinci, Daniela Pedicino, Francesco Trotta, Aureliano Ruggio, Giuseppe P Piemontese, Domenico Galante, Myriana Ponzo, Luigi M Biasucci, Giovanna Liuzzo, Filippo Crea, Matrix metalloproteinase-9 might affect adaptive immunity in non-ST segment elevation acute coronary syndromes by increasing CD31 cleavage on CD4+ T-cells, European Heart Journal, Volume 39, Issue 13, 01 April 2018, Pages 1089–1097, https://doi.org/10.1093/eurheartj/ehx684
- Share Icon Share
Abstract
In patients with acute coronary syndrome (ACS), the higher activity of effector T-cells suggests that mechanisms involving adaptive immunity dysregulation might play a role in coronary instability. The shedding of the functional CD31 domain 1–5 leads to uncontrolled lymphocyte activation. In experimental models, matrix metalloproteinase-9 (MMP-9) has been implicated in endothelial CD31 cleavage. Interestingly, higher serum levels of MMP-9 have been observed in ACS. We aim to investigate the mechanisms underlying CD31 dysregulation in ACS.
To assess CD31 cleavage on CD4+ T-cells, we analysed by flow cytometry CD4+ T-cells of 30 ACS, 25 stable angina (SA) patients, and 28 controls (CTRL) using two different CD31 antibodies that specifically recognize domain 1–5 or the non-functional membrane-proximal domain 6. The ratio between the domains was significantly lower in ACS than in SA and CTRL (P = 0.002 ACS vs. SA; P = 0.002 ACS vs. CTRL). After stimulation with anti-CD3/CD28, the 1–5/6 domain ratio was significantly lower in ACS than in SA (P = 0.005). ELISA of supernatants obtained from T-cell receptor-stimulated CD4+ T-cells showed higher production of MMP-9 in ACS than in SA (P < 0.001). CD31 domain 1–5 expression in activated CD4+ T-cells from ACS patients increased after treatment with a specific MMP-9 inhibitor (P = 0.042).
Our study suggest that enhanced MMP-9 release plays a key role in determining the cleavage and shedding of the functional CD31 domain 1–5 in CD4+ T-cells of ACS patients. This mechanism might represent an important therapeutic target to modulate T-cell dysregulation in ACS.
Translational Perspective
The immune system is a complex network tightly modulated by several feedback loops. Elevated soluble markers of inflammation in patients with acute coronary syndrome (ACS) are associated with worse outcomes and might derive from abnormalities in innate and adaptive immunity cross-talk.
The higher plasmatic matrix metalloproteinase-9 (MMP-9) concentration consistently observed in ACS, affecting CD31 expression, might explain the systemic T-cell abnormalities typical of these patients. So, molecules such as MMP-9 and CD31, could represent desirable molecular targets for specific anti-inflammatory treatments and might be used as clinical biomarkers of prognosis in patients with ACS.
Introduction
In patients with acute coronary syndrome (ACS) and systemic evidence of inflammation, the higher frequency and enhanced function of effector T-cells compared with patients with stable angina (SA) suggests that the sudden changes leading to coronary instability might be related to mechanisms involving dysregulation of adaptive immunity.1–4
CD31 is a member of the immunoglobulin (Ig) superfamily of cell adhesion molecules. It is expressed on most cells of the haematopoietic lineage including platelets, monocytes, neutrophils, and T-cells and plays an important role in the inflammatory response through the modulation of leucocyte activation, cytokine production, and the maintenance of vascular barrier integrity.5 , 6 CD31 is a type I transmembrane glycoprotein consisting in an extracellular region composed of six Ig-like homology domains, a 19-residue transmembrane domain, and a 118-residue cytoplasmic tail.7 The biological properties of CD31 in cellular adhesion and signalling have been mapped to specific regions of the CD31 molecule. Extracellular Ig-like domain 1–5 contains residues that are important for mediating the homophilic CD31 interactions and that are critical for the inhibition of T-cell receptor (TCR) signalling through the action of protein tyrosine-phosphatases recruited by its Immunoreceptor Tyrosine-based Inhibition Motifs (ITIMs). Thus, the cleavage and shedding of CD31 Ig-like domain 1–5 from the surface of T-cells upon activation via their TCR prevent homophilic binding interaction and the consequent activation of CD31 ITIM inhibitory pathway, finally leading to uncontrolled lymphocyte activation.8 , 9
CD31 has been also implicated in the development of atherosclerosis in animal models10 and of its clinical complications in humans.11 CD31 expression and function on CD4+ T-cells is transiently reduced at the time of the acute event in patients with ACS,12 and it might contribute to the immune dysregulation observed in this setting.12–15
In murine experimental models, matrix metalloproteinase-9 (MMP-9) seems to be implicated in endothelial CD31 cleavage.16 Of note, higher serum levels of MMP9 in ACS have been consistently observed in patients with poor disease outcome and plaque vulnerability.17–19
In this study, we analysed the expression of CD31 functional domain 1–5 and CD31 membrane-proximal domain 6 on circulating T-cells from patients with ACS (non-ST-segment elevation myocardial infarction), SA, or controls (CTRL) before and after TCR-stimulation. We sought to investigate if the reduced CD31 expression previously observed on peripheral T-cells of ACS is caused by cleavage and shedding of functional domain 1–5, rather than its total absence.
We also investigated the mechanisms underlying CD31 down-regulation in ACS by studying the role of MMP-9 in the modulation of CD31 expression.
Methods
For a detailed description of all methods, see the Supplementary material online, Appendix.
Study population
We enrolled 30 patients admitted to our Coronary Care Unit with a diagnosis of non-ST-elevation myocardial infarction (NSTEMI). As control groups, we enrolled 25 patients with chronic SA and 28 individuals without a previous history and/or current symptoms or signs of ischaemic heart disease (CTRL). All patients gave their written informed consent. The study has been approved by the Ethics Committee of the Catholic University of Rome. Supplementary material online, Table S1, reports demographic and clinical characteristics of the study population.
Statistical analysis
The continuous variables that were normally distributed, as assessed by the Shapiro–Wilk test, were described as mean ± SD and analysed with parametric tests. For comparisons among the three groups, we used one-way analysis of variance (ANOVA) with Bonferroni correction. For multiple pairwise comparisons, we used two-way ANOVA for repeated measures, with Bonferroni correction. For between-group comparisons, we used an unpaired Student’s t-test. To compare the means of two related samples within groups, we used a paired samples Student’s t-test. Data that did not follow a normal distribution were analysed by using non-parametric test. We used the Kruskal–Wallis non-parametric ANOVA and the Dunn’s test for comparisons among groups, and the Friedman test for repeated measures for multiple pairwise comparisons, as appropriate. For between-group comparisons, we used Mann–Whitney U-test. To compare two related samples within groups, we used the Wilcoxon test. A two-tailed P-value <0.05 was considered statistically significant. Statistical analysis was performed with GraphPad Prism version 5.00 (GraphPad Software, San Diego, CA, USA) and SPSS version 18.0 (SPSS Inc., Chicago, IL, USA).
Results
Populations
The main features of the study populations are summarized in the Supplementary material online, Table S1.
Baseline surface expression of CD31 domains on CD4+ T-cells
To establish whether the reduced expression of the CD31 molecule previously observed on peripheral T-cells of ACS is restricted only to the functional domain 1–5 or extended to the totality of its six extracellular Ig-like domains, we performed multicolour flow cytometry analysis of peripheral blood mononuclear cells (PBMCs). We analysed PBMCs obtained from all the patients enrolled in the study (30 ACS patients, 25 SA patients, and 28 CTRL).
We used two different CD31 antibodies specifically recognizing either the membrane-distal Ig-like domain 1–5 (clone WM59) or the membrane-proximal Ig-like domain 6 (clone MBC 78.2) (see the Supplementary material online, Appendix).
As shown in Figure 1A and B, C, D31 functional domain 1–5 surface expression on unstimulated naïve (1.54 ± 0.52 in ACS, 1.99 ± 0.45 in SA, 1.89 ± 0.50 in CTRL; P = 0.001 ACS vs. SA, P = 0.009 ACS vs. CTRL) and memory T-cells (1.57 ± 0.55 in ACS, 2.02 ± 0.62 in SA, 1.84 ± 0.50 in CTRL; P = 0.001 ACS vs. SA, P = 0.049 ACS vs. CTRL) was lower in ACS patients as compared with SA and CTRL.
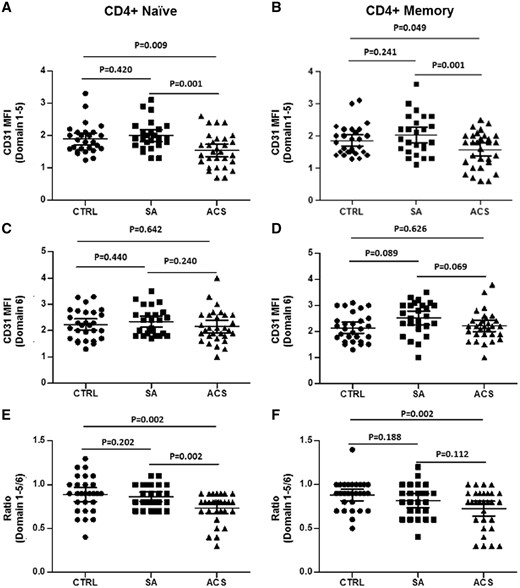
Basal CD31 expression on CD4+ T-cells. Flow-cytometric analysis of CD31 expression on T-cell subsets. (A, B) CD31 domain 1–5 surface expression (assessed by clone WM59 median fluorescence intensity) on naïve and memory CD4+ T-cells was significantly lower in acute coronary syndrome than stable angina and controls. (C, D) No differences were observed among the three groups of study in CD31 domain 6 surface expression (assessed by clone MBC78.2 median fluorescence intensity) on naïve and memory T-cells. (E) Acute coronary syndrome patients showed a reduced ratio between domain 1–5/domain 6 in naïve T-cells as compared with stable angina and controls. (F) Acute coronary syndrome patients showed a reduced ratio between domain 1–5/domain 6 in memory T-cells as compared with controls. Naïve and memory CD4+ T-cells were identified as CD4+CD45RA+CD45RO− and CD4+CD45RA−CD45RO+, respectively. Data are presented as single data points and mean 95% CI (30 acute coronary syndrome patients, 25 stable angina patients, and 28 controls were included in the analysis).
No significant differences were observed in the expression of CD31 domain 6 among the three study groups for both naïve and memory T-cells (Figure 1C and D). Accordingly, the ratio between domain 1–5 and 6 in naïve T-cells was significantly lower in ACS when compared with SA and CTRL (0.73 ± 0.20 in ACS, 0.86 ± 0.10 in SA, 0.89 ± 0.20 in CTRL; P = 0.002 ACS vs. SA, P = 0.002 ACS vs. CTRL) (Figure 1E). A similar trend was observed for memory T-cells (0.72 ± 0.23 in ACS, 0.88 ± 0.17 in SA, 0.81 ± 0.19 in CTRL; P = 0.002 ACS vs. CTRL) (Figure 1F).
These results suggest a selective down-regulation of the CD31 functional domain 1–5 in CD4+ T-cells from ACS patients compared to SA and CTRL.
Surface expression of CD31 domains on CD4+ T-cells after T-cell receptor-stimulation
After TCR stimulation, CD31 differently modulates the expression of its two domains on T-cells.9 Therefore, CD4+ T-cells from ACS and SA patients were stimulated for 24 h, 72 h, and 6 days with anti-CD3/CD28 monoclonal antibodies (mAb) and the expression of the two CD31 domains was assessed as previously described (eight patients for each group of study were analysed).
As shown in Figure 2A, after TCR stimulation of CD4+ T-cells, the expression of CD31 domain 1–5 was lower in ACS than in SA (24 h: 0.97 ± 0.25 vs. 1.97 ± 0.60, P < 0.001; 72 h: 2.61 ± 1.11 vs. 4.32 ± 1.10, P = 0.007; 6 days 2.55 ± 0.88 vs. 3.88 ± 0.97, P = 0.01). No differences were observed in CD31 domain 6 expressions (Figure 2B). Accordingly, the ratio between domain 1–5 and 6 in CD4+ T-cells was significantly lower in ACS when compared with SA (24 h: 0.48 ± 0.25 vs. 0.79 ± 0.27, P = 0.03; 72 h: 0.8 ± 0.23 vs. 1.03 ± 0.30, P = 0.05; 6 days 0.72 ± 0.21 vs. 1.02 ± 0.13, P = 0.005) (Figure 2C). Soluble CD31 levels in the supernatants of in vitro activated CD4+ T-cells, as assessed by ELISA, were higher (although not significantly) in ACS as compared with SA patients after 6 days of TCR stimulation (five patients for each group of study were analysed) (Figure 2D).
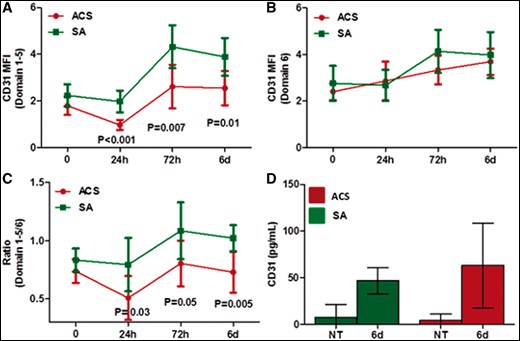
CD31 expression after T-cell receptor stimulation. CD4+ T-cells were activated in vitro with aCD3/aCD28 for T-cell receptor stimulation. Cells were harvested after 24 h, 72 h, and 6 days of stimulation and CD31 domain surface expression was assessed by flow cytometry. (A) Acute coronary syndrome showed a reduced expression of CD31 domain 1–5 on CD4+ T-cells as compared with stable angina. (B) No differences were observed in CD31 domain 6 surface expression on CD4+ T-cells after T-cell receptor stimulation. (C) Accordingly, the ratio between domain 1–5/domain 6 was significantly lower in acute coronary syndrome when compared with stable angina. Cumulative data from eight acute coronary syndrome and eight stable angina patients. Values are fold-increases relative to levels in untreated CD4+ T-cells (95% CI). (D) Soluble CD31 levels in the supernatants of in vitro activated CD4+ T-cells, as assessed by ELISA, were higher (although not significantly) in acute coronary syndrome as compared with stable angina patients after 6 days of T-cell receptor stimulation (five patients for each study group). Green lines and histograms: stable angina; red lines and histograms: acute coronary syndrome. MFI, median fluorescence intensity; NT, untreated.
All together, these results suggest that the selective down-regulation of the CD31 functional domain 1–5 in ACS is linked to T-cell activation.
CD31 mRNA expression in CD4+ T-cells
To quantify CD31 mRNA expression and the CD31 functional domain 1–5 protein expression simultaneously, PrimeFlow® RNA Assay was performed in ACS and SA patients (five patients for each group of study were analysed). This technique allows quantifying mRNA expression at single cells levels by flow cytometry, avoiding cell sorting.
PrimeFlow mRNA® experiments revealed that both naïve and memory CD4+ T-cells, as well as CD31+ and CD31-CD4+ T-cells, had the same CD31 mRNA level (Figure 3A and B), suggesting the existence of a post-transcriptional mechanism of CD31 protein regulation in T-cells.
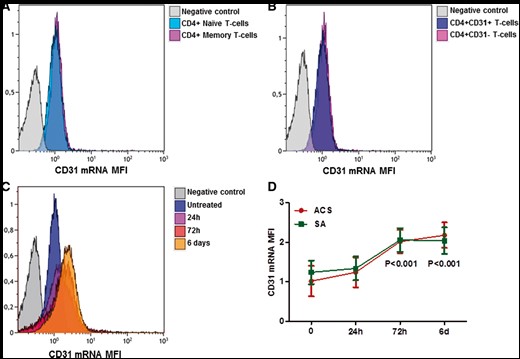
CD31 mRNA expression in CD4+ T-cells. CD31 mRNA expression in CD4+ T-cells was assessed by PrimeFlow® assay. (A) Representative flow cytometric histogram plot of CD31 mRNA expression in naïve and memory CD4+ T-Cells at basal level. (B) Representative flow cytometric histogram plot of CD31 mRNA expression in CD31 + (clone WM59 to detect the CD31 membrane-distal domain 1–5) and CD31-CD4+ T-cells at basal level. (C) Representative flow cytometric histogram plot of CD31 mRNA expression in CD4+ T-cells after TCR stimulation with anti-CD3/CD28 antibodies. (D) Trends over time of the CD31 mRNA expression in T-cell receptor-stimulated CD4+ T-cells obtained from acute coronary syndrome and stable angina patients (five patients for each study group). Data are presented as mean 95% CI. Green lines: stable angina; red lines: acute coronary syndrome. *P < 0.001 compared to basal condition.
After in vitro activation of TCR signalling with anti-CD3/CD28 mAb crosslink, CD31 mRNA expression significantly increased at 72 h and 6 days in both groups (P < 0.001 for both groups at 72 h and 6 days vs. basal condition), and no differences were observed between ACS and SA patients (Figure 3C and D). These results support the hypothesis that the mechanism triggering CD31 down-regulation in ACS is due to a selective loss of CD31 domain 1–5.
Matrix metalloproteinase-9 mRNA expression in CD4+ T-cells and MMP-9 release
In order to identify CD31 cleavage sites, we used a software for the prediction of novel substrates and their cleavage sites (see Supplementary material online). This analysis suggested a role for MMP-9 in CD31 cleavage, so we decided to assess the expression of MMP-9 mRNA in CD4+ T-cells obtained from 24 ACS and 20 SA patients. As shown in Figure 4A, ACS patients showed higher expression of MMP-9 mRNA compared with SA (860.6 ± 674.3 vs. 371.0 ± 440.1; P = 0.009).
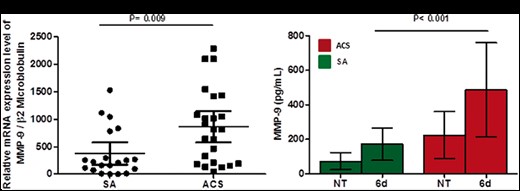
Matrix metalloproteinase-9 expression in CD4+ T-cells at baseline and after T-cell receptor stimulation. (A) Matrix metalloproteinase-9 mRNA expression was assessed by quantitative real-time polymerase chain reaction in CD4+ T-cells (20 stable angina patients and 24 acute coronary syndrome patients were included). Data are presented as single data points, mean 95% CI. (B) Matrix metalloproteinase-9 protein levels were assessed by ELISA in the supernatants of CD4+ T-cells at baseline and after 6 days of T-cell receptor stimulation (five patients for each group of study). Data are presented as mean 95% CI. Green histograms: stable angina; Red histograms: acute coronary syndrome. MFI, median fluorescence intensity; NT, untreated.
T-cells constitutively produce small amounts of MMP-9 that are up-regulated after activation,20 therefore we stimulated CD4+ T-cells from ACS and SA patients with anti-CD3/CD28 mAb crosslink and performed ELISA assay of supernatants. Acute coronary syndrome patients showed higher levels of MMP-9 as compared with SA after stimulation (487 ± 260.3 pg/mL vs. 172 ± 88.8 pg/mL P < 0.001) (Figure 4B) (six patients for each group of study were analysed). No differences were observed between the two groups at baseline.
Effects of matrix metalloproteinase-9 inhibition on CD31 expression in CD4+ T-cells from acute coronary syndrome patients
To determine MMP-9 involvement in CD31 cleavage, we treated CD4+ T-cells obtained from ACS with the specific MMP-9 inhibitor I. As shown in Figure 5A and C, we observed an increase of CD31 domain 1–5 expression in 6 days of TCR-stimulated CD4+ T-cells after MMP-9 inhibition (1.41 ± 0.30 vs. 3.01 ± 1.3; P = 0.042) (five patients for each group of study were analysed). No differences were observed in CD31 domain 6 (Figure 5B).
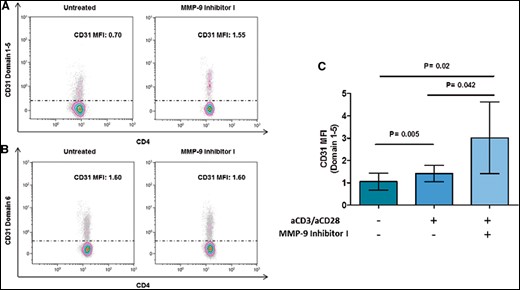
Effects of matrix metalloproteinase-9 inhibition on CD31 expression in CD4+ T-cells from acute coronary syndrome patients. CD4+ T-cells were stimulated for 6 days with anti-CD3/CD28 monoclonal antibodies with or without matrix metalloproteinase-9 inhibitor I. (A, B) Representative flow cytometric histogram plots of CD31 domain 1–5 and CD31 domain 6 surface expression on T-cell receptor-stimulated CD4+ T-cells before and after matrix metalloproteinase-9 inhibition. (C) CD31 domain 1–5 surface expression on T-cell receptor-stimulated CD4+ T-cells from five acute coronary syndrome patients before and after matrix metalloproteinase-9 inhibitions. After matrix metalloproteinase-9 inhibitions, activated CD4+ T-cells showed a higher expression of CD31 domain 1–5. Data are presented as mean 95% CI. MFI, median fluorescence intensity.
Role of circulating matrix metalloproteinase-9 in CD31 cleavage
Consistent data have shown that higher serum levels of MMP-9 in ACS are associated with poor disease outcome and plaque vulnerability.17–19 In order to study the role of circulating MMP-9 in CD31 cleavage, we performed experiments using serum from ACS and SA patients.
CD4+ T-cells obtained from SA and ACS patients were incubated with serum obtained from SA or ACS patients, with or without MMP9 inhibitor I. As shown in Figure 6A, incubation for 24 h of CD4+ T-cells obtained from five SA patients with serum obtained from ACS patients resulted in a reduction of CD31 domain 1–5 expression (1.3 ± 0.28 after incubation with ACS serum vs. 1.93 ± 0.44 in basal condition, P = 0.023). This reduction was not anymore observed when MMP-9 inhibitor was added to the cell cultures. In the same experimental conditions, when CD4+ T-cells from five ACS patients were incubated with serum obtained from SA or ACS patients, and with or without MMP9 inhibitor, no differences were observed in the expression of CD31 domain 1–5 (Figure 6B).
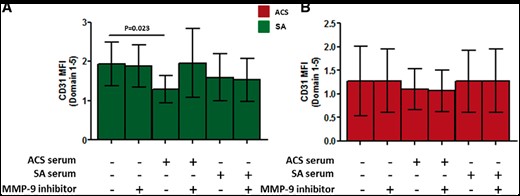
Role of circulating matrix metalloproteinase-9 in CD31 cleavage. CD31 domain 1–5 surface expression on CD4+ T-cells from five stable angina (left panel, green histograms) and from five acute coronary syndrome patients (right panel, red histograms). CD4+ T-cells were incubated for 24 h with serum obtained from acute coronary syndrome or stable angina patients in presence or absence of matrix metalloproteinase-9 inhibitor I. Only CD4+ T-cells from stable angina incubated with acute coronary syndrome serum showed a significant reduction of CD31 domain 1–5. This effect was prevented by the addition of matrix metalloproteinase-9 inhibitor I. Data are presented as mean 95% CI. MFI, median fluorescence intensity.
Discussion
The biological activity of CD31 is carried out by homophilic interaction. During antigen presentation, the homophilic nature of CD31 facilitates lymphocyte engagement with other cells expressing CD31 such as antigen presenting cells (APCs), platelets and endothelial cells (ECs). CD31 triggering modulates T-cell activation by inhibiting TCR signalling. The shedding of CD31 Ig-like domain 1–5 prevents homophilic binding interaction and the consequent activation of CD31 ITIM/SHP2 inhibitory pathway.
CD31 plays an important immunoregulatory role by setting the threshold for T-cell activation. Its involvement has been described in several inflammatory diseases, including atherosclerosis and plaque destabilization.8–13 CD4 + T-cells from patients with ACS show an enhanced response to TCR stimulation and a lower activation threshold, at least partially caused by an enhanced amplification of proximal TCR-mediated signals.3 , 21 The importance of the TCR signal-strength in the differentiation of helper T-cell subsets has increasingly been documented.22 A lower setting of the T-cell activation threshold could affect the T-cell differentiation fate, leading to an imbalanced expansion of effector T-cell and regulatory T-cell subsets observed in patients with ACS.3 , 23–25 The altered activity of proteins involved in the modulation of upstream TCR signalling, such as CD31, might modify the phosphorylation of downstream effectors and finally the T-cell commitment. Moreover, CD31 signalling modulates the overall expression of lineage-specifying transcription factors for proinflammatory Th1 and Th17 cells, e.g. through modulation of the Mitogen-activated protein kinases (MAPK) pathways.12
Our experiments suggested a role for MMP-9 in CD31 shedding, a mechanism similar to that observed with other Ig-like transmembrane molecules. Notably, several studies have described high plasma and serum levels of MMP-9 in patients with ACS.17–19 Moreover, plasma MMP-9 concentration was identified as predictor of cardiovascular mortality in patients with symptomatic coronary artery disease.19
Our study suggests that the reduced expression of the CD31 functional domain 1–5 in patients with ACS as compared to SA patients and CTRL might be at least partially mediated by MMP-9. Indeed, an MMP-9-dependent enhanced cleavage of CD31 domain 1–5 might promote excessive TCR activation thus influencing T-cell homeostasis (Take home figure). Loss of CD31 Ig-like domain 1–5 prevents the activation of CD31 ITIM/SHP2 inhibitory pathways. The consequent reduction of CD31 signalling might lead to uncontrolled T-cell activation and altered naïve T-cells differentiation.8 , 9 , 12 This is confirmed by our observation that CD4+ T-cells from SA patients incubated with serum from ACS patients exhibit reduced expression of CD31 functional domain 1–5, which is prevented by MMP-9 inhibition. These findings suggest a possible autocrine effect of MMP-9 on activated CD4+ T-cells, which further amplifies their activation by generating a positive feedback. In alternative, the effect of MMP-9 on CD4+ T-cell activation might provide an additional link between innate and adaptive immunity in ACS. Indeed, this metalloprotease is produced by several cell populations including monocytes, lymphocytes, and ECs that might contribute to the overall bulk of plasma MMP-9. Overall, the higher expression of this protein could affect coronary stability not only by promoting plaque fissuring as previously described26 , 27 but also by increasing T-cell activation and by promoting T-cell differentiation towards a pro-inflammatory phenotype. Moreover, MMP-9 expression in ECs is transcriptionally regulated in response to shear stress.28 CD31 acts as mechanosensor that mediates EC responses to shear stress and ECs are able to act as antigen-presenting-cells.29 Thus, MMP-9 expression might also be critical during T cell–EC interaction, by modulating CD31 expression, and thus providing a further link between the immune system and the vascular wall. Notably, T-cells can stimulate MMP-9 endothelial production through a CD40-CD40L contact-dependent mechanism.30 This process might further affect the CD31 expression, and thus contribute to T-cell hyper-reactivity in the atherosclerotic plaque.
Limitations
This study has several limitations. Indeed, it was a prospective observational analysis that included a limited number of patients. No power calculation could be performed because of a lack of previous studies in this setting; these limitations imply that several variables other than the coronary disease state might explain the differences observed across these three small populations. Indeed, differences regarding gender, smoke, and diabetes that are known to influence MMP-9 expression were found among the groups. However, our results seem to suggest that those factors, as well as others increasing MMP-9 levels, might influence plaque stability in ACS also through MMP-9, by determining the cleavage and shedding of the functional CD31 domain 1–5 on CD4+ T-cells. Importantly, we were unable to determine a cause–effect relationship. To this regard, we cannot exclude that loss of CD31 and the higher production of MMP-9 might be part of the general stress response in the acute setting of ACS. Moreover, although our experiments with MMP-9 inhibitor suggest a role for this protease in CD31 cleavage, other shedding mechanisms cannot be excluded.
In this study, we did not evaluate MMP-9 total activity in cell supernatant. However, our inhibition experiments showed a significant recovery of CD31 domain 1–5 by the addition of MMP-9 inhibitor I, suggesting an increased activity of this metalloprotease. Finally, we have not had the opportunity to collect coronary plaque specimens in ACS and SA patients, thus losing information on the role of CD31 on T-cell locally resident in unstable plaques.
Although the coronary plaque characteristics were not assessed in this study, our results introduce a new role for MMP-9 in adaptive immunity dysregulation widely described in ACS3 , 23–25 and provide novel insights into the mechanisms responsible for coronary instability. Taken together the results of this study and of our previous studies on this topic,12 , 13 we propose the following sequence of events in patients with ACS and systemic evidence of inflammation: MMP-9, released by innate immunity cells and by T-cells, causes the cleavage of CD31 domain 1–5; the increased expression of MMP-9 might affect TCR-dependent T-cell activation and induce T-cell hyper-reactivity, through the alteration of cellular pathways linked to CD31 cleavage (Take home figure).
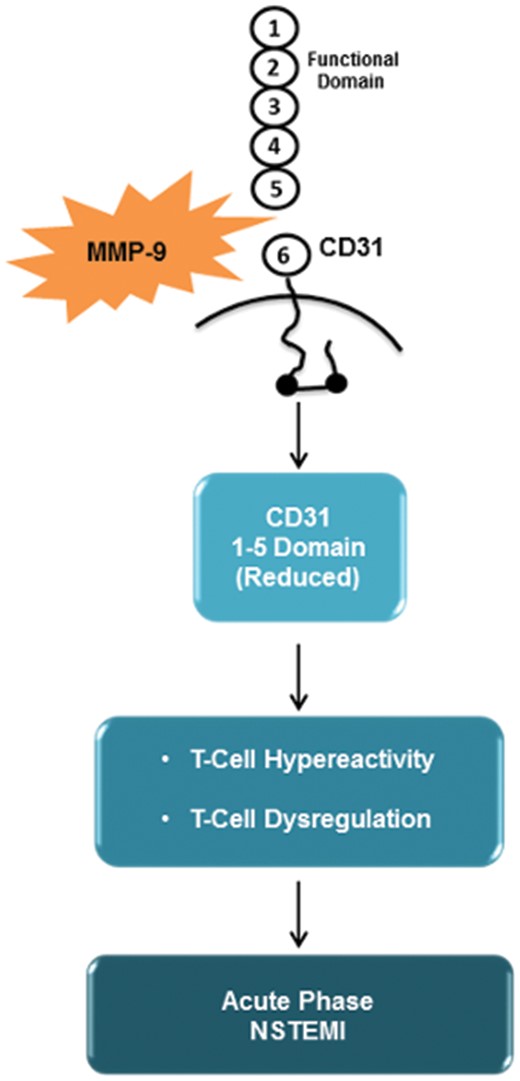
This schematic outline describe matrix metalloproteinase-9 involvement in adaptive immunity dysregulation observed during the acute phase of acute coronary syndrome. See the text for description.
Supplementary material
Supplementary material is available at European Heart Journal online.
Funding
Catholic University of the Sacred Heart, Rome, Italy [R4124500302 LINEA D.1 2016].
Conflict of interest: none declared.
References
Author notes
Giulia Angelini and Davide Flego have contributed equally as first authors.
Giovanna Liuzzo and Filippo Crea have contributed equally as last authors.
See page 1098 for the editorial comment on this article (doi: 10.1093/eurheartj/ehx743)