-
PDF
- Split View
-
Views
-
Cite
Cite
Clemens Kietaibl, Ines Horvat Menih, Adrian Engel, Roman Ullrich, Klaus U Klein, Gabor Erdoes, Cerebral microemboli during extracorporeal life support: a single-centre cohort study, European Journal of Cardio-Thoracic Surgery, Volume 61, Issue 1, January 2022, Pages 172–179, https://doi.org/10.1093/ejcts/ezab353
- Share Icon Share
Abstract
The aim of this study was to investigate the load and composition of cerebral microemboli in adult patients undergoing venoarterial extracorporeal life support (ECLS).
Adult ECLS patients were investigated for the presence of cerebral microemboli and compared to critically ill, pressure-controlled ventilated controls and healthy volunteers. Cerebral microemboli were detected in both middle cerebral arteries for 30 min using transcranial Doppler ultrasound. Neurological outcome (ischaemic stroke, global brain ischaemia, intracerebral haemorrhage, seizure, metabolic encephalopathy, sensorimotor sequelae and neuropsychiatric disorders) was additionally evaluated.
Twenty ECLS patients (cannulations: 15 femoro-femoral, 4 femoro-subclavian, 1 femoro-aortic), 20 critically ill controls and 20 healthy volunteers were analysed. ECLS patients had statistically significantly more cerebral microemboli than critically ill controls {123 (43–547) [median (interquartile range)] vs 35 (16–74), difference: 88 [95% confidence interval (CI) 19–320], P = 0.023} and healthy volunteers [11 (5–12), difference: 112 (95% CI 45–351), P < 0.0001]. In ECLS patients, 96.5% (7346/7613) of cerebral microemboli were of gaseous composition, while solid cerebral microemboli [1 (0–5)] were detected in 12 out of 20 patients. ECLS patients had more neurological complications than critically ill controls (12/20 vs 3/20, P = 0.003). In ECLS patients, a high microembolic rate (>100/30 min) tended to be associated with neurological complications including ischaemic stroke, neuropsychiatric disorders, sensorimotor sequelae and non-convulsive status epilepticus (odds ratio 4.5, 95% CI 0.46–66.62; P = 0.559).
Our results indicate that adult ECLS patients are continuously exposed to many gaseous and, frequently, to few solid cerebral microemboli. Prolonged cerebral microemboli formation may contribute to neurological morbidity related to ECLS treatment.
ClinicalTrials.gov, NCT02020759, https://clinicaltrials.gov/ct2/show/NCT02020759?term=erdoes&rank=1.
INTRODUCTION
Venoarterial extracorporeal life support (ECLS) is increasingly used as bridging therapy in critically ill patients with severe cardiorespiratory failure or recovering from major cardiothoracic surgery [1, 2]. However, patients are at high risk for treatment-related neurological complications including embolic stroke, intracerebral haemorrhage and long-term neurocognitive impairment [3, 4]. Similar to cardiopulmonary bypass (CPB) circuits, ECLS configurations bypassing the pulmonary circulation may induce microemboli entering the cerebral circulation [5, 6], which could critically affect cerebral integrity [5, 7].
During CPB procedures, median numbers of cerebral microemboli range from >100 up to >1000 in total [8]. However, these findings are not equally applicable to ECLS treatment due to substantial differences in the circuit composition and field of applications [5, 6]. While ECLS systems are closed circuits without blood–air interface and reduced artificial surface, the venous reservoir, the cardiotomy suction and surgical manipulations of the aorta and supra-aortic arteries are major sources of cerebral microemboli during CPB support [5]. Moreover, ECLS patients are predominantly normothermic with ejecting hearts in contrast to hypothermic CPB patients with cardioplegia-induced cardiac arrest. Further differences include pump types [centrifugal pumps (ECLS) versus roller pumps (CPB)], cannulation strategies (mostly peripheral during ECLS versus central during CPB) and anticoagulation regimes (lower heparin levels during ECLS). Hence, limited evidence [9, 10] exists on the load and composition of cerebral microemboli in ECLS patients.
The primary study goal was to quantify and qualify (gaseous versus solid) cerebral microemboli in adult ECLS patients using transcranial Doppler ultrasound [11]. Cerebral blood flow velocity of both middle cerebral arteries was simultaneously measured. Critically ill, pressure-controlled ventilated patients without extracorporeal treatment served as the control group, and healthy volunteers were investigated for reference values. Neurological outcome was additionally evaluated.
PATIENTS AND METHODS
Complying with the Declaration of Helsinki, the study was approved by the Ethics Committee of the Medical University of Vienna (reference number: 1557/2013, date: 01 August 2013) and registered with ClinicalTrials.gov (reference number: NCT02020759, date: 25 December 2013). The study design was a single-centre cohort study, and the study was performed according to the STROBE recommendations for observational studies on methods [12]. Since ECLS and critically ill control patients were sedated, they signed written informed consent after regaining the capacity to give consent. Due to the purely observational study design, local Ethics Committee allowed including ECLS patients who died without consent. All study participants were prospectively investigated for the presence of cerebral microemboli at the Medical University of Vienna (June 2014–February 2019). Neurological outcome was retrospectively evaluated (July 2020–August 2020).
Adult ECLS patients were selected from 6 in-house critical care units including patients after major cardiothoracic surgery, with acute (perioperative) cardiac failure, and with acute respiratory distress syndrome (ARDS). Exclusion criteria were: intermittent and continuous renal replacement therapy (microemboli generation [13]), any additional mechanical circulatory support devices, untreated severe mitral or aortic stenosis/regurgitation, internal carotid artery stenosis >70%, atrial fibrillation, septicaemia, vascular dementia/Alzheimer’s disease and age <18 years. The patients were mostly under pressure-controlled ventilation and sedated with propofol and remifentanil. Anticoagulation was performed either with unfractionated heparin (target activated partial thromboplastin time: 60–80 s) or with low-molecular-weight heparin (target anti-Xa activity: 0.3–0.5).
The control group consisted of critically ill, pressure-controlled ventilated patients after major surgeries/interventional procedures, or with ARDS/severe pneumonia. Exclusion criteria were any extracorporeal treatment, trauma/orthopaedic surgeries (microemboli generation [14]), laparoscopic surgeries (carbon dioxide insufflation) and the exclusion criteria applied to ECLS patients. Sedation was performed with propofol and remifentanil, and standard thrombosis prevention with low-molecular-weight heparin. Both ECLS and control patients were recruited using convenience sampling.
Healthy volunteers (18–30 years, half of them female) were investigated for a baseline rate of cerebral microemboli potentially related to signal errors of transcranial Doppler technology.
Cerebral microemboli (primary study outcome) and cerebral blood flow velocity (secondary study outcome) were continuously recorded for 30 min during a haemodynamically stabilized time period independently from ECLS implantation time. The investigations did not affect the individual patient treatment or diagnostic measures and were carried out once in accordance with the clinical routine work of the particular critical care unit. All study participants were investigated in supine position under nearly steady-state conditions. No patient care activities were performed, anaesthetic agents and vasopressors remained at the same level and both ECLS and ventilator settings remained unchanged. Patient monitoring included continuous recordings of arterial blood pressure (non-invasive blood pressure in healthy volunteers), electrocardiogram and peripheral oxygen saturation.
Reviewing digital patient records, we retrospectively evaluated neurological outcome (secondary study outcome)—defined as the diagnosis of ischaemic stroke, global brain ischaemia, intracerebral haemorrhage, seizure, metabolic encephalopathy, sensorimotor sequelae and neuropsychiatric disorders. Specifically, all patients had been examined for clinical signs of neurological injury including 6-hourly pupil controls and at least once-daily physical examinations for asymmetries/paresis/paralysis. Awake patients had been daily tested for delirium using the Confusion Assessment Method for the Intensive Care Unit. A cranial computed tomography scan or electroencephalography had been performed in patients suspicious of stroke or seizure. A neurological/neuropsychiatric consultation had been carried out in patients with neurological/neuropsychiatric disorders.
The ECLS systems consisted of heparin-coated tubes, an integrated heat exchanger, and the following centrifugal pumps/hollow fibre membrane oxygenators: (i) Biomedicus Bio-Pump BP80, Medtronic, Minneapolis, MN, USA—Affinity NT oxygenator, Medtronic or Eurosets A.L.ONE ECMO Adult oxygenator, Medolla, Italy; (ii) HLS Module Advanced 7.0, Maquet, Rastatt, Germany; and (iii) Deltastream DP3 diagonal rotary pump, Xenios, Heilbronn, Germany—Medos HILITE 7000 LT oxygenator, Xenios. All venous drainage cannulae were percutaneously inserted into the right/left femoral vein positioning the tip at the level of the right atrium. The arterial reinfusion cannula was commonly inserted into the right femoral artery using open cutdown Seldinger technique. Further arterial cannulation sites were the right subclavian artery (end-to-side graft) or the ascending aorta (chest opening and direct surgical cannulation). The level of anticoagulation and the blood flow were checked before the investigation. The ECLS circuit—including the in- and outflow side of the oxygenator and the visible part of the arterial cannula—was daily inspected by a perfusionist for fibrin depositions and thrombi.
We used an advanced, multifrequency pulsed transcranial Doppler device (Doppler BoxX, Compumedics Germany/DWL, Singen, Germany). Insonating both middle cerebral arteries through the temporal acoustic window, cerebral microemboli were continuously detected with a pulsed-wave transducer at 2 and 2.5 MHz. A head frame (DiaMon, Compumedics Germany/DWL) retained the transducers’ position. High-intensity transient signals were differentiated into artefacts, solid or gaseous cerebral microemboli by transcranial Doppler software algorithms. Emboli differentiation basically relies on the principle that solid emboli reflect more ultrasound at 2.5 than at 2 MHz, while gaseous emboli have the opposite characteristic [11, 15]. All measurements were performed by 3 experienced investigators (C.K, I.H.M. and A.E.) in compliance with the guidelines from the International Consensus Group on microembolus detection [16, 17]. Cerebral microemboli were counted in real-time automatically. In addition, all measurements were evaluated (continuous signal quality check, artefact identification). The insonation depth was 55–65 mm, the threshold for microembolic signals was 9 dB, the sample volume length was 8 mm and the pulse repetition frequency was 7 kHz. Simultaneously, cerebral blood flow velocity of both middle cerebral arteries was recorded.
The power analysis was based on the initial study populations of ECLS patients and healthy volunteers including an effect size (d) of 1.26 standard deviations (SD), an alpha level of 0.05 and a power of 0.8. Using pre-existing microembolic rates in ECLS patients [9, 10] and in patients before orthopaedic surgery [14], and considering potential artefacts in awake study participants [15], microembolic signals/30 min were estimated as follows: 100 (105) [mean (SD)] for ECLS patients and 6 (5) for healthy volunteers. Calculated sample sizes were 20 for both groups. After investigating these initial study populations, we prospectively investigated 20 critically ill control patients to contextualize microembolic rates in ECLS patients within the critical care setting.
Gaussian distribution was examined by the D’Agostino–Pearson omnibus test. Non-normally distributed data were tested for differences within a single group applying the Wilcoxon matched-pairs signed-ranks test, between 2 groups applying the Mann–Whitney U-test, and between 3 groups applying the Kruskal–Wallis test followed by the Dunn’s multiple comparisons test. Normally distributed data were tested for differences within a single group using the paired t-test, between 2 groups using the unpaired t-test, and between 3 groups using one-way ANOVA followed by the Tukey’s multiple comparisons test. Correlations between ECLS parameters and microemboli were calculated using the Spearman correlation. Group differences of categorical variables were tested using the χ2 or Fisher’s exact test. Associations between cerebral microemboli/cerebral blood flow velocities and neurological complications were calculated using odds ratios. A P-value of <0.05 was considered statistically significant and multiplicity-adjusted P-values were provided for multiple comparisons. GraphPad Prism software version 7.0d for Mac OS X was used for statistics (GraphPad Software, La Jolla, CA, USA).
RESULTS
Out of 25 ECLS patients screened for eligibility, 23 were enrolled [2 screen failures due to venovenous extracorporeal membrane oxygenation (ECMO)]. After 3 exclusions (2 informed refusals, 1 ineligible temporal acoustic window), 20 ECLS patients were analysed (Table 1). ECLS indications were: intraoperative weaning failures from extracorporeal cardiopulmonary support during double lung transplantation (n = 9), pulmonary endarterectomy (n = 2) and cardiac valve surgery (n = 2); right ventricular failure due to pulmonary embolism during nephrectomy (n = 1), amniotic fluid embolism during delivery (n = 1) and aggravated pulmonary hypertension before caesarean section (n = 1); cardiogenic shock after pneumonectomy (n = 1) and coronary artery bypass surgery (n = 1); and ARDS with cardiac failure (n = 2). The cannulation site was femoro-femoral (n = 15), femoro-subclavian (2 patients after cardiac valve surgery, 2 ARDS patients) and femoro-aortic (1 patient with cardiogenic shock after coronary artery bypass surgery).
Characteristics . | ECLS patients (n = 20) . | Critically ill controls (n = 20) . | P-value . |
---|---|---|---|
Age (years), mean (range) | 52.1 (20.2–78.4) | 60.0 (32.3–92.8) | 0.141 |
Female gender, n (%) | 8 (40) | 8 (40) | 1 |
Body mass index (kg/m2), mean (SD) | 25.3 (4.8) | 24.7 (4.7) | 0.700 |
Simplified acute physiology score 3, mean (SD) | 54.8 (8.1) | 52.3 (12.0) | 0.559 |
Arteriosclerosis, n (%)a | 7 (35) | 3 (15) | 0.273 |
ECLS settings, median (IQR) | |||
FsO2 (%) | 95 (80–100) | n.a | |
Blood flow (l/min) | 2.2 (1.8–2.9) | n.a. | |
Pump rotation (rpm) | 2390 (2155–2695) | n.a. | |
Sweep gas flow (l/min) | 2.5 (2.0–4.5) | n.a. | |
Operating time (ECLS start—investigation) (h) | 35.5 (17.3–46.8) | n.a. | |
CPB prior to ECLS, n (%) | 5 (25) | n.a. | |
Elapsed time (CPB end—investigation) (h), median (IQR) | 26.0 (10.5–38.5) | n.a. | |
Pre-ECLS cardiac arrest, n (%) | 5 (25) | n.a. | |
Mechanical ventilation, n (%) | 19 (95) | 20 (100) | 1 |
FiO2 (%), median (IQR) | 45 (40–60) | 35 (30–40) | <0.0001 |
Positive end-expiratory pressure (mbar), median (IQR) | 8 (8–10) | 6 (5–8) | 0.001 |
Mean airway pressure (mbar), median (IQR) | 13 (10–15) | 10 (8–12) | 0.019 |
Respiratory minute volume (l), median (IQR) | 4.6 (3.3–6.3) | 6.4 (5.7–7.5) | 0.002 |
Spontaneous respiration, n (%) | 1 (5)b | 0 (0) | 1 |
Gas exchange, mean (SD) | |||
Arterial pH | 7.45 (0.11) | 7.42 (0.06) | 0.089 |
PaO2 (kPa) | 15.6 (8.0) | 15.5 (3.5) | 0.441 |
PaCO2 (kPa) | 5.2 (1.1) | 5.4 (0.8) | 0.298 |
Anticoagulation, n (%) | |||
Low-molecular-weight heparin | 12 (60) | 8 (40) | 0.206 |
Unfractionated heparin | 3 (15) | 0 (0) | 0.231 |
Argatroban | 1 (5) | 0 (0) | 1 |
Nonec | 4 (20) | 12 (60) | 0.010 |
Level of anticoagulation and blood count, mean (SD) | |||
Prothrombin time (%) | 61 (18) | 60 (16) | 0.741 |
Activated partial thromboplastin time (s) | 59 (38)d | 40 (8) | 0.029 |
Anti-Xa activity | 0.17 (0.09)d,e | n.a. | |
Thrombocytes (109/l) | 65 (31) | 203 (116) | <0.0001 |
Haemoglobin (g/dl) | 9.5 (1.5) | 10.1 (1.5) | 0.256 |
Central lines, median (IQR) | 2 (1–2) | 1 (1–1) | <0.001 |
Fluid administration (ml), median (IQR) | 25 (8–50) | 25 (6–44) | 0.632 |
Syringe pumps, median (IQR) | 6 (5–7) | 3 (3–4) | <0.0001 |
Catecholamines | |||
Noradrenaline (µg/kg/min), mean (SD) n (%) | 0.19 (0.23) 18 (90) | 0.10 (0.08) 14 (70) | 0.425 0.235 |
Dobutamine (µg/kg/min), mean (SD) n (%) | 4.4 (1.3) 9 (45) | n.a. | |
Cardiac output (l/min), median (IQR)f | 2.5 (2.2–3.6) | n.a. |
Characteristics . | ECLS patients (n = 20) . | Critically ill controls (n = 20) . | P-value . |
---|---|---|---|
Age (years), mean (range) | 52.1 (20.2–78.4) | 60.0 (32.3–92.8) | 0.141 |
Female gender, n (%) | 8 (40) | 8 (40) | 1 |
Body mass index (kg/m2), mean (SD) | 25.3 (4.8) | 24.7 (4.7) | 0.700 |
Simplified acute physiology score 3, mean (SD) | 54.8 (8.1) | 52.3 (12.0) | 0.559 |
Arteriosclerosis, n (%)a | 7 (35) | 3 (15) | 0.273 |
ECLS settings, median (IQR) | |||
FsO2 (%) | 95 (80–100) | n.a | |
Blood flow (l/min) | 2.2 (1.8–2.9) | n.a. | |
Pump rotation (rpm) | 2390 (2155–2695) | n.a. | |
Sweep gas flow (l/min) | 2.5 (2.0–4.5) | n.a. | |
Operating time (ECLS start—investigation) (h) | 35.5 (17.3–46.8) | n.a. | |
CPB prior to ECLS, n (%) | 5 (25) | n.a. | |
Elapsed time (CPB end—investigation) (h), median (IQR) | 26.0 (10.5–38.5) | n.a. | |
Pre-ECLS cardiac arrest, n (%) | 5 (25) | n.a. | |
Mechanical ventilation, n (%) | 19 (95) | 20 (100) | 1 |
FiO2 (%), median (IQR) | 45 (40–60) | 35 (30–40) | <0.0001 |
Positive end-expiratory pressure (mbar), median (IQR) | 8 (8–10) | 6 (5–8) | 0.001 |
Mean airway pressure (mbar), median (IQR) | 13 (10–15) | 10 (8–12) | 0.019 |
Respiratory minute volume (l), median (IQR) | 4.6 (3.3–6.3) | 6.4 (5.7–7.5) | 0.002 |
Spontaneous respiration, n (%) | 1 (5)b | 0 (0) | 1 |
Gas exchange, mean (SD) | |||
Arterial pH | 7.45 (0.11) | 7.42 (0.06) | 0.089 |
PaO2 (kPa) | 15.6 (8.0) | 15.5 (3.5) | 0.441 |
PaCO2 (kPa) | 5.2 (1.1) | 5.4 (0.8) | 0.298 |
Anticoagulation, n (%) | |||
Low-molecular-weight heparin | 12 (60) | 8 (40) | 0.206 |
Unfractionated heparin | 3 (15) | 0 (0) | 0.231 |
Argatroban | 1 (5) | 0 (0) | 1 |
Nonec | 4 (20) | 12 (60) | 0.010 |
Level of anticoagulation and blood count, mean (SD) | |||
Prothrombin time (%) | 61 (18) | 60 (16) | 0.741 |
Activated partial thromboplastin time (s) | 59 (38)d | 40 (8) | 0.029 |
Anti-Xa activity | 0.17 (0.09)d,e | n.a. | |
Thrombocytes (109/l) | 65 (31) | 203 (116) | <0.0001 |
Haemoglobin (g/dl) | 9.5 (1.5) | 10.1 (1.5) | 0.256 |
Central lines, median (IQR) | 2 (1–2) | 1 (1–1) | <0.001 |
Fluid administration (ml), median (IQR) | 25 (8–50) | 25 (6–44) | 0.632 |
Syringe pumps, median (IQR) | 6 (5–7) | 3 (3–4) | <0.0001 |
Catecholamines | |||
Noradrenaline (µg/kg/min), mean (SD) n (%) | 0.19 (0.23) 18 (90) | 0.10 (0.08) 14 (70) | 0.425 0.235 |
Dobutamine (µg/kg/min), mean (SD) n (%) | 4.4 (1.3) 9 (45) | n.a. | |
Cardiac output (l/min), median (IQR)f | 2.5 (2.2–3.6) | n.a. |
Atherosclerosis of the aorta or the carotid/coronary/peripheral arteries.
Oxygen delivery by face mask.
Preceding lysis therapy (1 ECLS patient) or postoperatively increased risk of bleeding.
Initial acceptance of lower levels of anticoagulation due to heparin-coated tubes.
Patients on low-molecular-weight heparin.
Assessed in 8 ECLS patients.
CPB: cardiopulmonary bypass; ECLS: extracorporeal life support; FiO2: fraction of inspired oxygen; FsO2: sweep gas inlet oxygen fraction; n.a.: not applicable; SD: standard deviation.
Characteristics . | ECLS patients (n = 20) . | Critically ill controls (n = 20) . | P-value . |
---|---|---|---|
Age (years), mean (range) | 52.1 (20.2–78.4) | 60.0 (32.3–92.8) | 0.141 |
Female gender, n (%) | 8 (40) | 8 (40) | 1 |
Body mass index (kg/m2), mean (SD) | 25.3 (4.8) | 24.7 (4.7) | 0.700 |
Simplified acute physiology score 3, mean (SD) | 54.8 (8.1) | 52.3 (12.0) | 0.559 |
Arteriosclerosis, n (%)a | 7 (35) | 3 (15) | 0.273 |
ECLS settings, median (IQR) | |||
FsO2 (%) | 95 (80–100) | n.a | |
Blood flow (l/min) | 2.2 (1.8–2.9) | n.a. | |
Pump rotation (rpm) | 2390 (2155–2695) | n.a. | |
Sweep gas flow (l/min) | 2.5 (2.0–4.5) | n.a. | |
Operating time (ECLS start—investigation) (h) | 35.5 (17.3–46.8) | n.a. | |
CPB prior to ECLS, n (%) | 5 (25) | n.a. | |
Elapsed time (CPB end—investigation) (h), median (IQR) | 26.0 (10.5–38.5) | n.a. | |
Pre-ECLS cardiac arrest, n (%) | 5 (25) | n.a. | |
Mechanical ventilation, n (%) | 19 (95) | 20 (100) | 1 |
FiO2 (%), median (IQR) | 45 (40–60) | 35 (30–40) | <0.0001 |
Positive end-expiratory pressure (mbar), median (IQR) | 8 (8–10) | 6 (5–8) | 0.001 |
Mean airway pressure (mbar), median (IQR) | 13 (10–15) | 10 (8–12) | 0.019 |
Respiratory minute volume (l), median (IQR) | 4.6 (3.3–6.3) | 6.4 (5.7–7.5) | 0.002 |
Spontaneous respiration, n (%) | 1 (5)b | 0 (0) | 1 |
Gas exchange, mean (SD) | |||
Arterial pH | 7.45 (0.11) | 7.42 (0.06) | 0.089 |
PaO2 (kPa) | 15.6 (8.0) | 15.5 (3.5) | 0.441 |
PaCO2 (kPa) | 5.2 (1.1) | 5.4 (0.8) | 0.298 |
Anticoagulation, n (%) | |||
Low-molecular-weight heparin | 12 (60) | 8 (40) | 0.206 |
Unfractionated heparin | 3 (15) | 0 (0) | 0.231 |
Argatroban | 1 (5) | 0 (0) | 1 |
Nonec | 4 (20) | 12 (60) | 0.010 |
Level of anticoagulation and blood count, mean (SD) | |||
Prothrombin time (%) | 61 (18) | 60 (16) | 0.741 |
Activated partial thromboplastin time (s) | 59 (38)d | 40 (8) | 0.029 |
Anti-Xa activity | 0.17 (0.09)d,e | n.a. | |
Thrombocytes (109/l) | 65 (31) | 203 (116) | <0.0001 |
Haemoglobin (g/dl) | 9.5 (1.5) | 10.1 (1.5) | 0.256 |
Central lines, median (IQR) | 2 (1–2) | 1 (1–1) | <0.001 |
Fluid administration (ml), median (IQR) | 25 (8–50) | 25 (6–44) | 0.632 |
Syringe pumps, median (IQR) | 6 (5–7) | 3 (3–4) | <0.0001 |
Catecholamines | |||
Noradrenaline (µg/kg/min), mean (SD) n (%) | 0.19 (0.23) 18 (90) | 0.10 (0.08) 14 (70) | 0.425 0.235 |
Dobutamine (µg/kg/min), mean (SD) n (%) | 4.4 (1.3) 9 (45) | n.a. | |
Cardiac output (l/min), median (IQR)f | 2.5 (2.2–3.6) | n.a. |
Characteristics . | ECLS patients (n = 20) . | Critically ill controls (n = 20) . | P-value . |
---|---|---|---|
Age (years), mean (range) | 52.1 (20.2–78.4) | 60.0 (32.3–92.8) | 0.141 |
Female gender, n (%) | 8 (40) | 8 (40) | 1 |
Body mass index (kg/m2), mean (SD) | 25.3 (4.8) | 24.7 (4.7) | 0.700 |
Simplified acute physiology score 3, mean (SD) | 54.8 (8.1) | 52.3 (12.0) | 0.559 |
Arteriosclerosis, n (%)a | 7 (35) | 3 (15) | 0.273 |
ECLS settings, median (IQR) | |||
FsO2 (%) | 95 (80–100) | n.a | |
Blood flow (l/min) | 2.2 (1.8–2.9) | n.a. | |
Pump rotation (rpm) | 2390 (2155–2695) | n.a. | |
Sweep gas flow (l/min) | 2.5 (2.0–4.5) | n.a. | |
Operating time (ECLS start—investigation) (h) | 35.5 (17.3–46.8) | n.a. | |
CPB prior to ECLS, n (%) | 5 (25) | n.a. | |
Elapsed time (CPB end—investigation) (h), median (IQR) | 26.0 (10.5–38.5) | n.a. | |
Pre-ECLS cardiac arrest, n (%) | 5 (25) | n.a. | |
Mechanical ventilation, n (%) | 19 (95) | 20 (100) | 1 |
FiO2 (%), median (IQR) | 45 (40–60) | 35 (30–40) | <0.0001 |
Positive end-expiratory pressure (mbar), median (IQR) | 8 (8–10) | 6 (5–8) | 0.001 |
Mean airway pressure (mbar), median (IQR) | 13 (10–15) | 10 (8–12) | 0.019 |
Respiratory minute volume (l), median (IQR) | 4.6 (3.3–6.3) | 6.4 (5.7–7.5) | 0.002 |
Spontaneous respiration, n (%) | 1 (5)b | 0 (0) | 1 |
Gas exchange, mean (SD) | |||
Arterial pH | 7.45 (0.11) | 7.42 (0.06) | 0.089 |
PaO2 (kPa) | 15.6 (8.0) | 15.5 (3.5) | 0.441 |
PaCO2 (kPa) | 5.2 (1.1) | 5.4 (0.8) | 0.298 |
Anticoagulation, n (%) | |||
Low-molecular-weight heparin | 12 (60) | 8 (40) | 0.206 |
Unfractionated heparin | 3 (15) | 0 (0) | 0.231 |
Argatroban | 1 (5) | 0 (0) | 1 |
Nonec | 4 (20) | 12 (60) | 0.010 |
Level of anticoagulation and blood count, mean (SD) | |||
Prothrombin time (%) | 61 (18) | 60 (16) | 0.741 |
Activated partial thromboplastin time (s) | 59 (38)d | 40 (8) | 0.029 |
Anti-Xa activity | 0.17 (0.09)d,e | n.a. | |
Thrombocytes (109/l) | 65 (31) | 203 (116) | <0.0001 |
Haemoglobin (g/dl) | 9.5 (1.5) | 10.1 (1.5) | 0.256 |
Central lines, median (IQR) | 2 (1–2) | 1 (1–1) | <0.001 |
Fluid administration (ml), median (IQR) | 25 (8–50) | 25 (6–44) | 0.632 |
Syringe pumps, median (IQR) | 6 (5–7) | 3 (3–4) | <0.0001 |
Catecholamines | |||
Noradrenaline (µg/kg/min), mean (SD) n (%) | 0.19 (0.23) 18 (90) | 0.10 (0.08) 14 (70) | 0.425 0.235 |
Dobutamine (µg/kg/min), mean (SD) n (%) | 4.4 (1.3) 9 (45) | n.a. | |
Cardiac output (l/min), median (IQR)f | 2.5 (2.2–3.6) | n.a. |
Atherosclerosis of the aorta or the carotid/coronary/peripheral arteries.
Oxygen delivery by face mask.
Preceding lysis therapy (1 ECLS patient) or postoperatively increased risk of bleeding.
Initial acceptance of lower levels of anticoagulation due to heparin-coated tubes.
Patients on low-molecular-weight heparin.
Assessed in 8 ECLS patients.
CPB: cardiopulmonary bypass; ECLS: extracorporeal life support; FiO2: fraction of inspired oxygen; FsO2: sweep gas inlet oxygen fraction; n.a.: not applicable; SD: standard deviation.
Out of 32 critically ill patients screened for the control group, 23 were enrolled (7 screen failures due to intraoperative extracorporeal cardiopulmonary support, 1 due to intraoperative carbon dioxide insufflation, 1 due to trauma surgery). After 3 exclusions (ineligible temporal acoustic windows), 20 patients were analysed (Table 1). Indications for pressure-controlled ventilation were: postoperatively after surgery for oral cavity cancer (n = 4), intestinal surgery (n = 2), liver transplantation (n = 2), cystectomy (n = 2), surgical debulking (n = 1), oesophageal perforation repair (n = 1), retroperitoneal lymph node dissection (n = 1), surgery for post-tonsillectomy haemorrhage (n = 1), pleurectomy decortication (n = 1), aortobifemoral bypass (n = 1), embolization of a gastroduodenal artery aneurysm (n = 1), stenting of oesophageal bleeding (n = 1); and severe pneumonia/ARDS (n = 2).
Reference values were obtained from 20 healthy volunteers {age [mean (range)]: 23.7 (19.8–28.0) years, body mass index [mean (SD)]: 23.1 (2.7) kg/m2, 10 female}.
Gaseous cerebral microemboli were detected in all study participants (at least 1 gaseous microembolus in each critically ill control/healthy volunteer). Solid cerebral microemboli were detected in 12 out of 20 ECLS patients. One singular solid microembolus was further detected in 1 critically ill control patient and 1 healthy volunteer. Total cerebral microemboli [median (interquartile range)/30 min] in ECLS patients were statistically significantly higher than in critically ill controls [123 (43–547) vs 35 (16–74), difference: 88 (95% confidence interval (CI) 19–320), P = 0.023] and healthy volunteers [11 (5–12), difference: 112 (95% CI 45–351), P < 0.0001] (Fig. 1A). In ECLS patients, 96.5% (7346/7613) of cerebral microemboli were of gaseous composition [gaseous: 122 (42–546) vs solid: 1 (0–5), difference: 121 (95% CI 40–403), P < 0.0001]. Notably, cerebral microemboli were distributed towards the left middle cerebral artery in ECLS patients but were equally distributed in both critically ill controls and healthy volunteers (Fig. 1B). Accordingly, predominant femoral artery cannulation (n = 15) was linked with a left-sided emboli distribution [left: 69 (20–469) vs right: 27 (9–97), difference: 42 (95% CI −7 to 228), P = 0.030]. Finally, cerebral microemboli/30 min correlated neither to the blood flow (R = −0.12, P = 0.605), pump rotation (R = 0.12, P = 0.623) or sweep gas flow (R = −0.30, P = 0.193), nor to the duration of ECLS treatment (R = 0.16, P = 0.505).
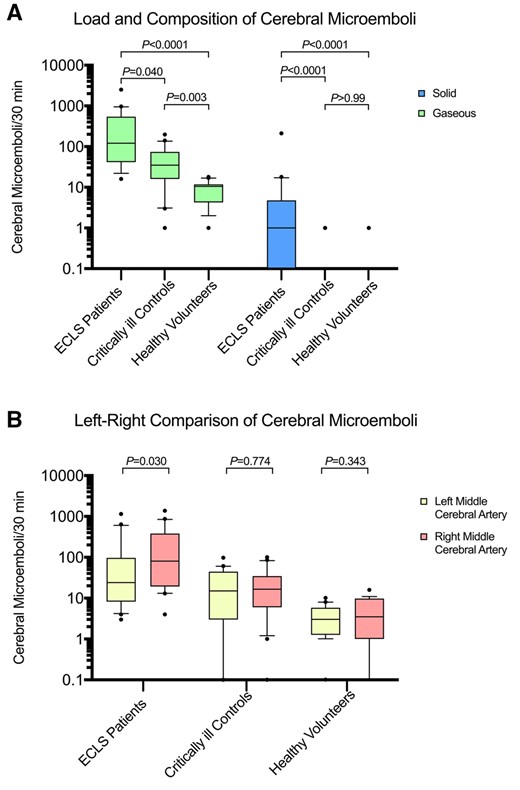
(A) Patients on venoarterial ECLS are exposed to a higher rate of gaseous cerebral microemboli than critically ill controls and healthy volunteers. ECLS patients show a low rate of solid cerebral microemboli. Multiplicity-adjusted P-values are reported. (B) Cerebral microemboli are distributed towards the left middle cerebral artery in ECLS patients but are equally distributed in critically ill controls and healthy volunteers. ECLS: extracorporeal life support.
In ECLS patients, cerebral blood flow velocity [mean velocity; mean (SD), cm/s] was slightly distributed towards the right middle cerebral artery [right: 38 (9) vs left: 34 (11), P = 0.047], while in critically ill controls and healthy volunteers, cerebral blood flow velocity was equally distributed (Table 2). Accordingly, left-sided cerebral blood flow velocity was lower in ECLS patients than in healthy volunteers.
Cerebral blood flow velocity of both middle cerebral arteries and haemodynamic parameters
Variables . | ECLS patients (n = 20) . | Critically ill controls (n = 20) . | P-valuea . | Healthy volunteers (n = 20) . | P-valuea . |
---|---|---|---|---|---|
Mean right cerebral blood flow velocity (cm/s), mean (SD) | 38 (9) | 44 (15) | 0.665 | 42 (10) | 0.850 |
Mean left cerebral blood flow velocity (cm/s), mean (SD) | 34 (11) | 45 (16) | 0.051 | 44 (8) | 0.004 |
Heart rate (beats/min), mean (SD) | 91 (17) | 65 (12) | <0.0001 | 65 (7) | <0.0001 |
MAP (mmHg), mean (SD) | 79 (13) | 70 (8) | 0.039 | 89 (9) | 0.010 |
Variables . | ECLS patients (n = 20) . | Critically ill controls (n = 20) . | P-valuea . | Healthy volunteers (n = 20) . | P-valuea . |
---|---|---|---|---|---|
Mean right cerebral blood flow velocity (cm/s), mean (SD) | 38 (9) | 44 (15) | 0.665 | 42 (10) | 0.850 |
Mean left cerebral blood flow velocity (cm/s), mean (SD) | 34 (11) | 45 (16) | 0.051 | 44 (8) | 0.004 |
Heart rate (beats/min), mean (SD) | 91 (17) | 65 (12) | <0.0001 | 65 (7) | <0.0001 |
MAP (mmHg), mean (SD) | 79 (13) | 70 (8) | 0.039 | 89 (9) | 0.010 |
Multiplicity adjusted P-values.
ECLS: extracorporeal life support; MAP: mean arterial pressure; SD: standard deviation.
Cerebral blood flow velocity of both middle cerebral arteries and haemodynamic parameters
Variables . | ECLS patients (n = 20) . | Critically ill controls (n = 20) . | P-valuea . | Healthy volunteers (n = 20) . | P-valuea . |
---|---|---|---|---|---|
Mean right cerebral blood flow velocity (cm/s), mean (SD) | 38 (9) | 44 (15) | 0.665 | 42 (10) | 0.850 |
Mean left cerebral blood flow velocity (cm/s), mean (SD) | 34 (11) | 45 (16) | 0.051 | 44 (8) | 0.004 |
Heart rate (beats/min), mean (SD) | 91 (17) | 65 (12) | <0.0001 | 65 (7) | <0.0001 |
MAP (mmHg), mean (SD) | 79 (13) | 70 (8) | 0.039 | 89 (9) | 0.010 |
Variables . | ECLS patients (n = 20) . | Critically ill controls (n = 20) . | P-valuea . | Healthy volunteers (n = 20) . | P-valuea . |
---|---|---|---|---|---|
Mean right cerebral blood flow velocity (cm/s), mean (SD) | 38 (9) | 44 (15) | 0.665 | 42 (10) | 0.850 |
Mean left cerebral blood flow velocity (cm/s), mean (SD) | 34 (11) | 45 (16) | 0.051 | 44 (8) | 0.004 |
Heart rate (beats/min), mean (SD) | 91 (17) | 65 (12) | <0.0001 | 65 (7) | <0.0001 |
MAP (mmHg), mean (SD) | 79 (13) | 70 (8) | 0.039 | 89 (9) | 0.010 |
Multiplicity adjusted P-values.
ECLS: extracorporeal life support; MAP: mean arterial pressure; SD: standard deviation.
ECLS patients had statistically significantly more neurological complications than critically ill control patients [12/20 (60%) vs 3/20 (15%), P = 0.003] (Table 3). In ECLS patients—although failing statistical significance—both a high microembolic rate (>100/30 min) and a low cerebral blood flow velocity (left or both sided <30 cm/s) tended to be associated with neurological complications including ischaemic stroke, neuropsychiatric disorders, sensorimotor sequelae and non-convulsive status epilepticus (Fig. 2A and B).
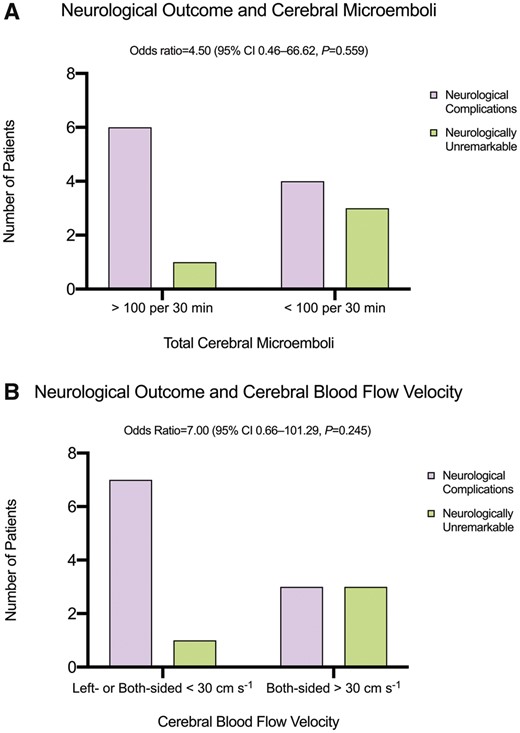
In patients on extracorporeal life support, (A) a high number of cerebral microemboli and (B) a low cerebral blood flow velocity tend to be associated (failing statistical significance) with neurological complications including ischaemic stroke, neuropsychiatric disorders, sensorimotor sequelae and non-convulsive status epilepticus.
Characteristic, n (%) . | ECLS patients (n = 20) . | Critically ill controls (n = 20) . | P-value . |
---|---|---|---|
Neurologically unremarkablea,b | 4 (20) | 16 (80) | 0.0001 |
Neurological complicationsc | 12 (60) | 3 (15) | 0.003 |
Global brain ischaemiad | 1 (5) | 0 | >0.99 |
Ischaemic stroke | 4 (20) | 0 | 0.106 |
Intracerebral haemorrhage | 1 (5) | 0 | >0.99 |
Non-convulsive status epilepticus | 1 (5) | 0 | >0.99 |
Severe metabolic encephalopathy | 1 (5) | 0 | >0.99 |
Sensorimotor sequelae | 4 (20) | 0 | 0.106 |
Neuropsychiatric/neurocognitive disorders | 7 (35) | 3 (15) | 0.273 |
Delirium | 3 (15) | 2 (10) | >0.99 |
Other | 4 (20) | 1 (5) | 0.342 |
Multiple neurological complications | 5 (25) | 0 | 0.047 |
Successful ECLS weaning | 14 (70) | n.a. | |
Transfer to general ward | 11 (55) | 18 (90) | 0.013 |
Death in the ICU | 9 (45) | 2 (10) | 0.013 |
Death related to neurological injury | 5 (25) | 0 | 0.047 |
Characteristic, n (%) . | ECLS patients (n = 20) . | Critically ill controls (n = 20) . | P-value . |
---|---|---|---|
Neurologically unremarkablea,b | 4 (20) | 16 (80) | 0.0001 |
Neurological complicationsc | 12 (60) | 3 (15) | 0.003 |
Global brain ischaemiad | 1 (5) | 0 | >0.99 |
Ischaemic stroke | 4 (20) | 0 | 0.106 |
Intracerebral haemorrhage | 1 (5) | 0 | >0.99 |
Non-convulsive status epilepticus | 1 (5) | 0 | >0.99 |
Severe metabolic encephalopathy | 1 (5) | 0 | >0.99 |
Sensorimotor sequelae | 4 (20) | 0 | 0.106 |
Neuropsychiatric/neurocognitive disorders | 7 (35) | 3 (15) | 0.273 |
Delirium | 3 (15) | 2 (10) | >0.99 |
Other | 4 (20) | 1 (5) | 0.342 |
Multiple neurological complications | 5 (25) | 0 | 0.047 |
Successful ECLS weaning | 14 (70) | n.a. | |
Transfer to general ward | 11 (55) | 18 (90) | 0.013 |
Death in the ICU | 9 (45) | 2 (10) | 0.013 |
Death related to neurological injury | 5 (25) | 0 | 0.047 |
A neurological event could not be ruled out in 4 ECLS patients (2 pre-ECLS cardiac arrests)/1 critically ill control due to a non-neurological death.
Including 1 pre-ECLS cardiac arrest/2 CPB procedures.
Including 2 pre-ECLS cardiac arrests/3 CPB procedures.
Prolonged mechanical cardiopulmonary resuscitation prior to ECLS.
CPB: cardiopulmonary bypass; ECLS: extracorporeal life support; ICU: intensive care unit; n.a.: not applicable.
Characteristic, n (%) . | ECLS patients (n = 20) . | Critically ill controls (n = 20) . | P-value . |
---|---|---|---|
Neurologically unremarkablea,b | 4 (20) | 16 (80) | 0.0001 |
Neurological complicationsc | 12 (60) | 3 (15) | 0.003 |
Global brain ischaemiad | 1 (5) | 0 | >0.99 |
Ischaemic stroke | 4 (20) | 0 | 0.106 |
Intracerebral haemorrhage | 1 (5) | 0 | >0.99 |
Non-convulsive status epilepticus | 1 (5) | 0 | >0.99 |
Severe metabolic encephalopathy | 1 (5) | 0 | >0.99 |
Sensorimotor sequelae | 4 (20) | 0 | 0.106 |
Neuropsychiatric/neurocognitive disorders | 7 (35) | 3 (15) | 0.273 |
Delirium | 3 (15) | 2 (10) | >0.99 |
Other | 4 (20) | 1 (5) | 0.342 |
Multiple neurological complications | 5 (25) | 0 | 0.047 |
Successful ECLS weaning | 14 (70) | n.a. | |
Transfer to general ward | 11 (55) | 18 (90) | 0.013 |
Death in the ICU | 9 (45) | 2 (10) | 0.013 |
Death related to neurological injury | 5 (25) | 0 | 0.047 |
Characteristic, n (%) . | ECLS patients (n = 20) . | Critically ill controls (n = 20) . | P-value . |
---|---|---|---|
Neurologically unremarkablea,b | 4 (20) | 16 (80) | 0.0001 |
Neurological complicationsc | 12 (60) | 3 (15) | 0.003 |
Global brain ischaemiad | 1 (5) | 0 | >0.99 |
Ischaemic stroke | 4 (20) | 0 | 0.106 |
Intracerebral haemorrhage | 1 (5) | 0 | >0.99 |
Non-convulsive status epilepticus | 1 (5) | 0 | >0.99 |
Severe metabolic encephalopathy | 1 (5) | 0 | >0.99 |
Sensorimotor sequelae | 4 (20) | 0 | 0.106 |
Neuropsychiatric/neurocognitive disorders | 7 (35) | 3 (15) | 0.273 |
Delirium | 3 (15) | 2 (10) | >0.99 |
Other | 4 (20) | 1 (5) | 0.342 |
Multiple neurological complications | 5 (25) | 0 | 0.047 |
Successful ECLS weaning | 14 (70) | n.a. | |
Transfer to general ward | 11 (55) | 18 (90) | 0.013 |
Death in the ICU | 9 (45) | 2 (10) | 0.013 |
Death related to neurological injury | 5 (25) | 0 | 0.047 |
A neurological event could not be ruled out in 4 ECLS patients (2 pre-ECLS cardiac arrests)/1 critically ill control due to a non-neurological death.
Including 1 pre-ECLS cardiac arrest/2 CPB procedures.
Including 2 pre-ECLS cardiac arrests/3 CPB procedures.
Prolonged mechanical cardiopulmonary resuscitation prior to ECLS.
CPB: cardiopulmonary bypass; ECLS: extracorporeal life support; ICU: intensive care unit; n.a.: not applicable.
DISCUSSION
This study shows that adult ECLS patients are continuously exposed to many gaseous and, frequently, to few solid cerebral microemboli, which could affect neurological outcome.
Only 2 studies have investigated cerebral microemboli in venoarterial ECLS patients so far. One case series [10] of 6 ECLS patients showed more gaseous than solid cerebral microemboli varying from <100 to >1200/15 min in total. Another study [9] about 42 venovenous ECMO and 11 ECLS patients, found a higher frequency of cerebral microemboli in ECLS patients (>100/60 min in 5 out of 11 patients). These findings of individually varying rates of cerebral microemboli are in accordance with our results. However, previously reported emboli counts may be biased since patients under continuous renal replacement therapy, a potentially major source of cerebral microemboli [13], were not excluded [9, 10]. In addition, in 1 study [9], cerebral microemboli were not differentiated into solid or gaseous ones. However, our findings reveal that ECLS treatment may induce in addition to a high rate of gaseous a low rate of solid cerebral microemboli (Figs 1A and 2A).
The ECLS circuit probably plays a key role in cerebral microemboli formation. First, gaseous cerebral microemboli can originate from the centrifugal pump or the oxygenator [5, 6]. Fibrin depositions/thrombi within the circuit or the arterial cannula and inorganic particles [7] may induce solid cerebral microemboli. Second, gaseous microemboli have been detected in the arterial line during ECLS [6]. Third, the left-sided distribution of cerebral microemboli in the ECLS population (Fig. 1B) may rely on the predominantly femoral site of the arterial cannula. Namely, the cannula’s retrograde outflow competing with the ejection of the heart may imply that the right middle cerebral artery preferably receives blood from the heart, while the left middle cerebral artery preferably receives blood potentially rich in emboli from the arterial cannula [3, 18]. Fourth, solid cerebral microemboli occurred almost only in ECLS patients, although they had less thrombocytes and a longer activated partial thromboplastin time than critically ill controls (Table 1). This finding substantiates that solid microemboli formation—potentially influenced by the atherosclerotic burden and the anticoagulation strategy—was probably related to ECLS treatment. Finally, previous research suggests that ECLS configurations bypassing the pulmonary circulation induce higher rates of cerebral microemboli than venovenous ECMO configurations [3, 9].
The significant rates of gaseous cerebral microemboli in critically ill control patients (Fig. 1A) suggest that individual patient factors substantially contribute to microemboli formation. In particular, specific features of individual intensive therapy including central venous lines [19], fluid administration [10] or drug infusion by syringe pumps [20] may be sources of gaseous cerebral microemboli. Accordingly, specific ECLS-related features (e.g. type and individual state of the oxygenator, pump type, different cannulation configurations) together with individual patient factors (e.g. anticoagulation strategy, central lines, fluid administration, native cardiac ejection) may explain individually varying rates of solid and gaseous microemboli.
Concerning the accuracy of transcranial Doppler ultrasound, various studies show a reliable classification (up to 95%) of cerebral microemboli [11, 15, 21]. However, a lower accuracy in specific clinical settings has been acknowledged [22]. In healthy volunteers, the unexpected rate of gaseous cerebral microemboli could either represent a few unrecognized artefacts (e.g. minimal head rotations [15]) or result from flow phenomena at vessel bifurcations [23].
The frequency of neurological events in our ECLS population (60%, Table 3) was higher than previously reported [24]. Specifically, previous research showed neurological complications (including brain death, ischaemic stroke, cerebral haemorrhage, and seizure) in 15.1% of ECLS patients [25] and a prevalence of 17.4% of cerebrovascular complications [26]. However, none of these studies assessed neuropsychiatric disorders—the predominant event (35%) in our study. Importantly, long-term neuropsychological impairment is common in ECLS patients [4]. Notably, a higher rate of ischaemic stroke (25%) occurred in ECLS patients with postcardiotomy shock [27]. In our ECLS group, numerous neurological risk factors—including preceding on-pump cardiac surgery (3 patients) [7, 27], inotrope use (9 patients) [25], a femoral arterial cannulation site carrying the risk for cerebral hypoperfusion and hypoxia (15 patients) [18, 25], ARDS (2 patients) [28] and pre-ECLS cardiac arrest (5 patients) [24, 25]—may have increased the frequency of neurological complications.
Cerebral microemboli potentially affect the brain by various pathomechanisms including microvasculature obstruction, the impairment of the blood–brain barrier and inflammatory reactions [5, 7, 29]. Conflicting study results on the impact of cerebral microemboli on neurocognitive outcome [7, 8] may rely on the complex pathophysiology underlying neurological deficits in patients with extracorporeal cardiopulmonary support [3, 5, 7]. Specifically, the precipitating underlying disease, the critical pre-ECLS circulatory state and ECLS-related features—including loss of cerebral autoregulation and pulsatile flow, inflammatory changes, thromboembolic events or anticoagulation-related haemorrhage—may be of major significance [3, 18, 24, 25]. In addition, prolonged mechanical ventilation, drug-induced coma, systemic inflammatory response to major surgery, metabolic disturbances and pre-existing brain disorders may contribute to the complex pathophysiology [28, 30]. In our study, a high microembolic rate and a low cerebral blood flow velocity tended to be associated with neurological complications (Fig. 2A and B). Furthermore, solid cerebral microemboli potentially affecting the brain more seriously than dissolvable gaseous ones [8] were almost only detected in ECLS patients. Finally, the total burden and embolic composition of cerebral microemboli and impaired cerebral blood flow patterns may contribute to neurological morbidity related to prolonged [1] ECLS treatment.
Limitations
A major study limitation is that the sample size was underpowered for a sensible correlation between cerebral microemboli and neurological outcome. Accordingly, neurological events could not be linked with different cannulation sites or specific risk factors including a preceding CPB procedure or pre-ECLS cardiac arrest. Evaluating neurological outcome retrospectively further implied that the protocol for the assessment of neurological events was not the same for all patients. Moreover, the rather small sample size including a primarily cardiothoracic surgical population may limit the generalizability of specific findings (e.g. left-sided distribution of cerebral microemboli, cerebral blood flow patterns, frequency of neurological events). Our study was further underpowered for a statistical analysis of solid microemboli related to specific cannulation configurations, aortic atherosclerosis or different anticoagulation strategies. Finally, concerning the limitations of transcranial Doppler ultrasound, aside from its operator dependency, a few false positive signals potentially related to transcranial Doppler technology cannot be excluded.
CONCLUSION
In summary, this study shows that adult patients on venoarterial ECLS are continuously exposed to many gaseous and, frequently, to few solid cerebral microemboli. The detection of gaseous cerebral microemboli in critically ill control patients further indicates that individual patient factors (e.g. central lines, fluid administration) contribute to overall microemboli formation. Finally, prolonged cerebral microemboli formation and impaired cerebral blood flow patterns could affect neurological outcome in ECLS patients.
Conflict of interest: All authors declare no competing interests. None of the authors’ professional and financial affiliations has influenced this work. All authors have no financial or ethical conflict of interest to declare.
Author contributions
Clemens Kietaibl: Conceptualization; Data curation; Formal analysis; Investigation; Methodology; Project administration; Resources; Supervision; Validation; Visualization; Writing—original draft; Writing—review & editing. Ines Horvat Menih: Data curation; Formal analysis; Investigation; Methodology; Resources; Software; Visualization; Writing—original draft; Writing—review & editing. Adrian Engel: Data curation; Investigation; Methodology; Writing—review & editing. Roman Ullrich: Conceptualization; Methodology; Resources; Supervision; Validation; Writing—review & editing. Klaus U. Klein: Conceptualization; Methodology; Resources; Supervision; Validation; Writing—review & editing. Gabor Erdoes: Conceptualization; Methodology; Project administration; Resources; Supervision; Validation; Writing—original draft; Writing—review & editing.
Reviewer information
European Journal of Cardio-Thoracic Surgery thanks Roman Gottardi and the other, anonymous reviewer(s) for their contribution to the peer review process of this article.
Preliminary data presented at the AIC, Innsbruck, Austria, 29 September 2016.
REFERENCES
ABBREVIATIONS
- ARDS
Acute respiratory distress syndrome
- CI
Confidence interval
- CPB
Cardiopulmonary bypass
- ECLS
Extracorporeal life support
- ECMO
Extracorporeal membrane oxygenation
- SD
Standard deviation