-
PDF
- Split View
-
Views
-
Cite
Cite
Patricia Marchio, Sol Guerra-Ojeda, Martín Aldasoro, Soraya Lilian Valles, Iván Martín-Gonzalez, Juan Bautista Martínez-León, Maria Dolores Mauricio, Jose Maria Vila, Relaxant and antiadrenergic effects of ranolazine in human saphenous vein, European Journal of Cardio-Thoracic Surgery, Volume 58, Issue 2, August 2020, Pages 277–285, https://doi.org/10.1093/ejcts/ezaa034
- Share Icon Share
Abstract
Ranolazine improves vascular function in animal models. We evaluate the effects of ranolazine on vascular function and adrenergic response in human saphenous vein.
Rings from 53 patients undergoing coronary artery bypass grafting were mounted in organ baths. Concentration–response curves to ranolazine were constructed in rings precontracted with phenylephrine, endothelin-1, vasopressin, KCl and the thromboxane A2 analogue U-46619. In rings precontracted with phenylephrine, relaxation to ranolazine was tested in the absence and presence of endothelial factors inhibitors, K+ channel blockers and verapamil. The effects of ranolazine on frequency–response and concentration–response curves to phenylephrine were performed in the absence and presence of endothelial factors inhibitors and K+ channel blockers. Endothelial nitric oxide synthase, α1 adrenergic receptor and large conductance Ca2+-activated K+ channel protein expressions were measured by Western blotting.
Ranolazine (10−9–10−4 M) produced a concentration-dependent relaxation only in rings precontracted with phenylephrine that was reduced by endothelial denudation, NG-nitro-l-arginine methyl ester (10−4 M), charybdotoxin (10−7 M) and verapamil (10−6 M). Ranolazine diminished adrenergic contractions induced by electrical field stimulation (2–4 Hz) and phenylephrine (10−9–10−5 M) that were prevented by tetraethylammonium (10−3 M) and charybdotoxin (10−7 M). Ranolazine significantly decreased α1 adrenergic receptor and increased large conductance Ca2+-activated K+ channel protein expression in the saphenous vein.
Ranolazine diminishes the adrenergic vasoconstriction, acting as α1 antagonist, and by increasing large conductance Ca2+-activated K+ channel involvement. The relaxant effects of ranolazine are partially mediated by endothelial nitric oxide, large conductance Ca2+-activated K+ channels and the blockade of voltage-dependent Ca2+ channels.
INTRODUCTION
Ranolazine, a piperazine derivative, is currently indicated as an add-on therapy for the management of patients with chronic stable coronary artery disease not adequately controlled by first-line drugs such as beta-blockers, calcium antagonists and nitrates [1, 2]. Ranolazine has also been proven to be effective for the management of heart failure [3] and heart rhythm disorders [4], including postoperative arrhythmias in patients undergoing coronary artery bypass grafting (CABG) [5]. Its cardioprotective effects are mainly mediated by the inhibition of late sodium current, which decreases calcium overload in myocardial cells [6], therefore stabilizing myocardial electrical activity, relieving left ventricle tension and improving coronary blood flow [7]. Ranolazine also reduces ischaemia by enhancing endothelial function in compromised areas [8, 9], with minimal effects on heart rate and blood pressure at therapeutic concentrations [10].
Results from animal studies demonstrate that ranolazine also improves peripheral vascular function in different vascular beds such as pig coronary and femoral arteries [11] and rat renal arteries [12]. The mechanisms involved are the enhancement of endothelium-dependent and independent vasodilation, the decrease in adrenergic tone and the inhibition of L-type Ca2+ channels [11, 12].
Saphenous vein is one of the most frequent vessels used for CABG. The 1-year incidence of saphenous vein grafts restenosis ranges between 10% and 30% [13], which is mainly attributed to thrombosis in damaged endothelium (early restenosis) and intimal hyperplasia [14] and, in the long term, to accelerated atherosclerosis [14]. Hence, the maintenance of vessel patency and endothelial integrity is of paramount importance in the management of these patients. In this regard, ranolazine improved endothelial dysfunction by reducing inflammatory markers levels in patients with stable coronary artery disease [9]. Moreover, ranolazine enhanced endothelium-dependent flow-mediated dilation in brachial arteries in patients with stable coronary artery disease [15] and type-II diabetes mellitus [16].
Hyperactivation of the adrenergic system after CABG contributes to atrial fibrillation, heart failure or hypertension, with beta-blockers being the mainstream treatment for most patients [17]. Ranolazine has been proven effective in the prevention and management of postoperative arrhythmias [18]. However, its effects on vascular tone have not yet been evaluated in vessels used for CABG. Therefore, the present study investigates the effects of ranolazine on vascular function and adrenergic response in human saphenous vein.
MATERIALS AND METHODS
Ethics statement and patients
All procedures were carried out in accordance with the principles of the Declaration of Helsinki. The study was approved by the Clinical Research Ethics Committee at the University Clinic Hospital, INCLIVA, Valencia, Spain. Informed consent was obtained from patients included in the study. Patients taking ranolazine prior to the CABG were excluded from the study. Human saphenous vein samples were harvested from 53 patients undergoing CABG and transported in chilled saline solution to the laboratory. The veins were cut in rings (3 mm in length) for in vitro experiments.
Organ bath studies
Rings for organ bath studies were mounted for isometric recording of tension. Two stainless steel L-shaped pins were introduced through the lumen of the vessel. One pin was fixed to the wall of the organ bath, and the other one was connected to a force-displacement transducer (FT03; Grass Instruments, West Warwick, RI, USA). Changes in isometric force were recorded on a Macintosh computer (Apple Corp., Cupertino, CA, USA) using the Chart, version 7, and a MacLab/8e data acquisition system (AD Instruments). Each ring was suspended in a 4-ml bath containing modified Krebs–Henseleit solution composed of (in mmol/l) NaCl, 115; KCl, 4.6; MgCl2⋅6H2O, 1.2; CaCl2, 2.5; NaHCO3, 25; glucose 11.1 and disodium EDTA, 0.01. The solution was equilibrated with 95% O2 and 5% CO2 to obtain a pH of 7.3–7.4, and temperature was held at 37°C. The optimal resting tension for human saphenous vein was 3 g. The rings were allowed to attain a steady level of tension during a 2-h accommodation period before testing. In some experiments, the endothelium was mechanically removed by insertion of a roughened stainless steel wire into the lumen and gently rolling the rings on wet filter paper. Endothelium was considered functional if relaxation to a single concentration of bradykinin (10−7 M) in rings precontracted with noradrenaline (10−7–3 × 10−7 M) was ≥50%. Contractile capacity of vascular smooth muscle was evaluated by the maximum effect to KCl (60 mM), which was similar in rings with and without endothelium.
To evaluate the relaxant response to ranolazine (Sigma-Aldrich, St Louis, MO, USA) to different contractile agonists, concentration–response curves to ranolazine (10−9–10−4 M) were performed in rings precontracted with phenylephrine (10−7–10−6 M), endothelin-1 (10−7 M), vasopressin (10−7 M), KCl (60 mM) and the thromboxane A2 analogue U-46619 (10−7 M). In another set of experiments, concentration–response curves to ranolazine (10−9–10−4 M) were performed in rings precontracted with phenylephrine (to reach a contraction of ∼60–70% of KCl 60 M) in the absence (control response) and presence of the cyclooxygenase inhibitor indomethacin (10−5 M), to inhibit the production of prostacyclin (PGI2) and NG-nitro-l-arginine methyl ester (l-NAME, 10−4 M), to inhibit the nitric oxide synthase. To examine the participation of K+ channels, concentration–response curves to ranolazine were determined in the presence of indomethacin plus l-NAME combined with tetraethylammonium (TEA, 10−3 M). To establish the participation of Ca2+-activated K+ channels, concentration–response curves to ranolazine were performed in the presence of indomethacin plus l-NAME combined with charybdotoxin (10−7 M), TRAM-34 (10−6 M) and apamin (10−6 M), inhibitors of both large (BKCa) and intermediate, intermediate and small conductance Ca2+-activated K+ channels. In another group of experiments, rings were incubated with verapamil (10−6 M), to evaluate the participation of L-type voltage-dependent Ca2+ channels.
Electrical field stimulation (EFS) was provided by a Grass S88 stimulator (Grass Instruments, Quincy, MA, USA) via 2 platinum electrodes positioned on each side and parallel to the axis of the ring, as previously described [19]. Frequency–response curves were determined with 15-s trains of pulses (15 V, 0.2 ms duration) at 2 and 4 Hz. A period of 10 min was allowed between stimulations. To study the effects of ranolazine on adrenergic vasoconstriction, frequency and concentration–response curves were performed in the absence and presence of ranolazine (10−6–10−4 M).
To evaluate the participation of PGI2, nitric oxide (NO) and K+ channels on the effect of ranolazine, the curves were performed in the presence of indomethacin (10−5 M), l-NAME (10−4 M) and TEA (10−3 M). To study the participation of Ca2+-activated K+ channels, frequency–response relationships and concentration–response curves to phenylephrine were determined in the presence of indomethacin and l-NAME (control response) combined with charybdotoxin (10−7 M), TRAM-34 (10−6 M) and apamin (10−6 M). Control (in the absence of inhibitors) and experimental (after 20 min incubation with inhibitors) responses were obtained from different rings.
Western blot analysis
Vein rings for protein expression were incubated in the organ bath during 20 min in control conditions and with ranolazine (10−5 M) and stored in liquid nitrogen at −80°C until analysis.
For the Western blot technique, vascular tissues were homogenized in lysis buffer (0.125 M Tris–HCl, pH 6.8, 2% SDS, 19% glycerol and 1%, v/v, protease inhibitors) and centrifuged at 12 000 g for 15 min at 4°C. Protein concentration was determined using the BCA method (Thermo Scientific Pierce BCA Protein Assay Kit, USA). Then, 0.5% (v/v) 2-mercaptoethanol and 1% bromophenol blue were added and the samples were heated for 5 min at 90°C. Proteins (20 μg) were separated on SDS–PAGE gels and transferred to polyvinylidene difluoride membranes pre-wetted with methanol in a humid environment using a transfer buffer (25 mM Tris, 190 mM glycine and 20% methanol). After transfer, membranes were blocked for 1 h in albumin 5% in TBS + Tween 20. Then, the following primary antibodies were incubated overnight at 4°C: polyclonal endothelial nitric oxide synthase antibody (1:500; catalogue no. bs-0163R; BIOSSUSA, USA); polyclonal α1A adrenergic antibody (1:500; catalogue no. NB100-78585; NOVUSBIO, Madrid, Spain); and polyclonal Maxi Potassium channel alpha antibody (BKCa; 1:500; catalogue no. ab3586; Abcam, UK). After incubation, membranes were washed 3 times with wash buffer TBS + Tween-20 and incubated for 1 h at room temperature with the secondary antibodies anti-rabbit IgG, HRP-linked antibody (1:1000; catalogue no. 7074; Cell Signaling Technology, Leiden, Netherlands) or goat anti-mouse IgG (H + L) secondary antibody, HRP (1:10 000; catalogue no. 31430; Thermo Fisher Scientific, Waltham, MA, USA). Membranes were washed 3 times, and the enhanced chemiluminescence method (Amersham Biosciences, Barcelona, Spain) was used for antibody detection. Autoradiography signals were analysed with ImageJ software (NIH Image, National Institutes of Health, Bethesda, MD, USA). The primary antibody monoclonal α-tubulin (B 7) (1:1000; catalogue no. sc 5286; Santa Cruz Biotechnology, Heidelberg, Germany) was used as a control for the amount of protein.
Chemicals
Substances and reagents were purchased from Sigma-Aldrich unless otherwise specified. Drugs for organ bath were prepared daily in normal saline solution.
Statistical analysis
Descriptive analyses were performed for every study variable, where continuous variables are expressed in mean ± standard deviation (SD) and categorical variables with absolute (n) and relative (percentages) frequency measures.
For organ bath experiments, values are expressed as mean ± standard error of mean, and for Western blot, values are expressed as mean ± SD. Relaxation was expressed as a percentage of inhibition of agonist-induced response. Contraction was expressed as a percentage of the response to KCl 60 mM. EC50 values (concentration of agonist producing half-maximum effect) were expressed as pD2 (−log EC50). Normality of the data was tested with the Shapiro–Wilk test. For intergroup comparisons following a normal distribution, one-way ANOVA was used. For non-normal distribution, the non-parametric Mann–Whitney U-test was applied. For multiple comparisons (post hoc), Bonferroni test has been applied. For the concentration–response curves, the comparison has been made on the representative parameters of the curves in the different experimental conditions, pD2 (−log EC50) and the maximum effect (Emax) using a two-way ANOVA. For electrical stimulation experiments and Western blot analysis, in which the same veins were used in control and experimental conditions, a paired t-test was used. The level of significance used was 5% (P < 0.05). Statistical analysis was performed using GraphPad Prism 8.3.0 (GraphPad Software, San Diego, CA, USA).
RESULTS
Baseline characteristics of patients
Samples were taken from 53 patients undergoing CABG (44 men and 9 women) aged 70.2 (SD ±8.9) years. Patients’ demographics, risk factors and baseline treatment are shown in Supplementary Material, Table S1.
Relaxant effects of ranolazine
Ranolazine induced a concentration-dependent relaxation in rings precontracted with phenylephrine (10−7–10−6 M) but not in rings precontracted with endothelin-1 (10−7 M), vasopressin (10−7 M), KCl (60 mM) and U-46619 (10−7 M) (Fig. 1).
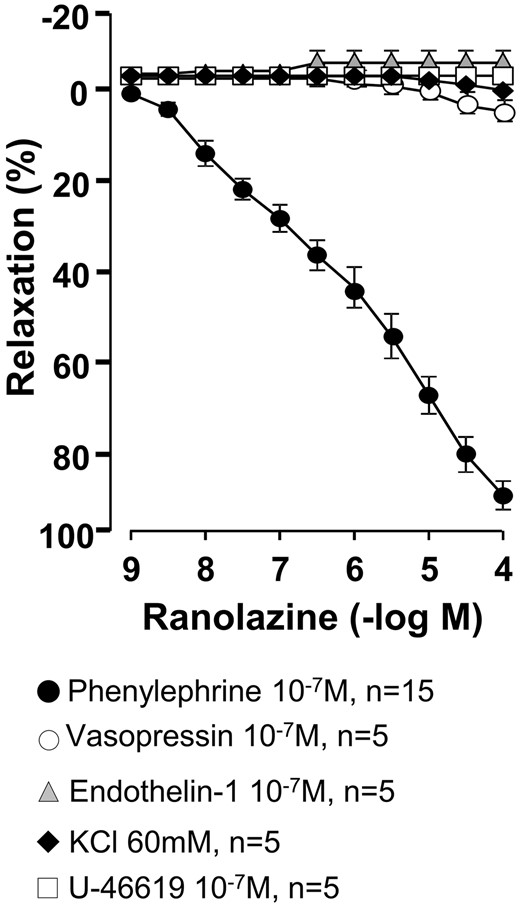
Relaxant effects of ranolazine on human saphenous rings precontracted with different agonists. Values are mean ± standard error of mean.
In rings precontracted with phenylephrine, denudation of endothelium significantly diminished the relaxation induced by ranolazine (pD2 = 6.55 ± 0.26 vs 5.56 ± 0.22, for intact and denuded rings, respectively, P = 0.01) (Fig. 2A). Indomethacin (10−5 M) did not affect the relaxation induced by ranolazine (data not shown). However, l-NAME (10−4 M) reduced the relaxation to ranolazine in rings with endothelium, indicating that part of the relaxation induced by ranolazine is due to the participation of NO (Fig. 2A). The endothelium-dependent relaxation to ranolazine after incubation with indomethacin plus l-NAME was further reduced by TEA (10−3 M) and charybdotoxin (10−7 M), whereas apamin (10−6 M) and TRAM-34 (10−6 M) had no effect (Fig. 2B), suggesting that large Ca2+-activated K+ channels participate in the relaxant response to ranolazine. The effects of charybdotoxin also occur in denuded rings (data not shown). In another set of experiments, verapamil (10−6 M) shifted the ranolazine–relaxation curve (10−9–10−4 M) to the right, decreasing the pD2 value (from 6.55 ± 0.26 to 5.40 ± 0.35; P = 0.02) without changes in the maximum effect (Emax = 94 ± 2 vs 93 ± 4 for control and verapamil, respectively, P = 0.68; n = 8). These results suggest that the relaxation induced by ranolazine is partially due to the blockage of L-type Ca2+ channels. Table 1 summarizes the pD2 and Emax values in the different experimental conditions.
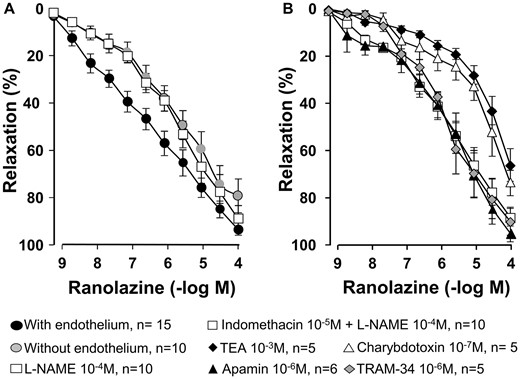
Concentration–response curves for ranolazine in human saphenous rings (A) with and without endothelium and in the presence of NG-nitro-l-arginine methyl ester (10−4 M) and (B) in control conditions (indomethacin 10−5 M plus NG-nitro-l-arginine methyl ester 10−4 M) and in the presence of tetraethylammonium (10−3 M), charybdotoxin (10−7 M), apamin (10−6 M) and TRAM-34 (10−6 M). Values are mean ± standard error of mean.
pD2 values and Emax to ranolazine in human saphenous rings precontracted with phenylephrine (10−7–10−6 M) in control and experimental conditions
Ranolazine . | n . | pD2 . | Emax . |
---|---|---|---|
Control | 15 | 6.55 ± 0.26 | 94 ± 2 |
l-NAME 10−4 M | 10 | 5.78 ± 0.18* | 90 ± 4 |
Indomethacin 10−5 M | 5 | 5.98 ± 0.45 | 99 ± 1 |
Indomethacin 10−5 M + l-NAME 10−4 M | 10 | 5.88 ± 0.17* | 88 ± 4 |
Indomethacin 10−5 M + l-NAME 10−4 M + TEA 10−3 M | 5 | 4.89 ± 0.11*,† | 66 ± 7*,† |
Indomethacin 10−5 M + l-NAME 10−4 M + charybdotoxin 10−7 M | 5 | 5.22 ± 0.43* | 73 ± 5*,† |
Indomethacin 10−5 M + l-NAME 10−4 M + TRAM-34 10−6 M | 5 | 5.84 ± 0.08 | 89 ± 6 |
Indomethacin 10−5 M + l-NAME 10−4 M + apamin 10−6 M | 6 | 5.60 ± 0.18 | 85 ± 8 |
Ranolazine . | n . | pD2 . | Emax . |
---|---|---|---|
Control | 15 | 6.55 ± 0.26 | 94 ± 2 |
l-NAME 10−4 M | 10 | 5.78 ± 0.18* | 90 ± 4 |
Indomethacin 10−5 M | 5 | 5.98 ± 0.45 | 99 ± 1 |
Indomethacin 10−5 M + l-NAME 10−4 M | 10 | 5.88 ± 0.17* | 88 ± 4 |
Indomethacin 10−5 M + l-NAME 10−4 M + TEA 10−3 M | 5 | 4.89 ± 0.11*,† | 66 ± 7*,† |
Indomethacin 10−5 M + l-NAME 10−4 M + charybdotoxin 10−7 M | 5 | 5.22 ± 0.43* | 73 ± 5*,† |
Indomethacin 10−5 M + l-NAME 10−4 M + TRAM-34 10−6 M | 5 | 5.84 ± 0.08 | 89 ± 6 |
Indomethacin 10−5 M + l-NAME 10−4 M + apamin 10−6 M | 6 | 5.60 ± 0.18 | 85 ± 8 |
Data are shown as mean ± SEM. Emax are expressed as a percentage of response to 60 mM KCl.
P < 0.05 versus control.
P < 0.05 versus indomethacin 10−5 M + l-NAME 10−4 M.
Emax: maximum effect; l-NAME: NG-nitro-l-arginine methyl ester; n: number of patients; pD2: −log EC50; SEM: standard error of mean; TEA: tetraethylammonium.
pD2 values and Emax to ranolazine in human saphenous rings precontracted with phenylephrine (10−7–10−6 M) in control and experimental conditions
Ranolazine . | n . | pD2 . | Emax . |
---|---|---|---|
Control | 15 | 6.55 ± 0.26 | 94 ± 2 |
l-NAME 10−4 M | 10 | 5.78 ± 0.18* | 90 ± 4 |
Indomethacin 10−5 M | 5 | 5.98 ± 0.45 | 99 ± 1 |
Indomethacin 10−5 M + l-NAME 10−4 M | 10 | 5.88 ± 0.17* | 88 ± 4 |
Indomethacin 10−5 M + l-NAME 10−4 M + TEA 10−3 M | 5 | 4.89 ± 0.11*,† | 66 ± 7*,† |
Indomethacin 10−5 M + l-NAME 10−4 M + charybdotoxin 10−7 M | 5 | 5.22 ± 0.43* | 73 ± 5*,† |
Indomethacin 10−5 M + l-NAME 10−4 M + TRAM-34 10−6 M | 5 | 5.84 ± 0.08 | 89 ± 6 |
Indomethacin 10−5 M + l-NAME 10−4 M + apamin 10−6 M | 6 | 5.60 ± 0.18 | 85 ± 8 |
Ranolazine . | n . | pD2 . | Emax . |
---|---|---|---|
Control | 15 | 6.55 ± 0.26 | 94 ± 2 |
l-NAME 10−4 M | 10 | 5.78 ± 0.18* | 90 ± 4 |
Indomethacin 10−5 M | 5 | 5.98 ± 0.45 | 99 ± 1 |
Indomethacin 10−5 M + l-NAME 10−4 M | 10 | 5.88 ± 0.17* | 88 ± 4 |
Indomethacin 10−5 M + l-NAME 10−4 M + TEA 10−3 M | 5 | 4.89 ± 0.11*,† | 66 ± 7*,† |
Indomethacin 10−5 M + l-NAME 10−4 M + charybdotoxin 10−7 M | 5 | 5.22 ± 0.43* | 73 ± 5*,† |
Indomethacin 10−5 M + l-NAME 10−4 M + TRAM-34 10−6 M | 5 | 5.84 ± 0.08 | 89 ± 6 |
Indomethacin 10−5 M + l-NAME 10−4 M + apamin 10−6 M | 6 | 5.60 ± 0.18 | 85 ± 8 |
Data are shown as mean ± SEM. Emax are expressed as a percentage of response to 60 mM KCl.
P < 0.05 versus control.
P < 0.05 versus indomethacin 10−5 M + l-NAME 10−4 M.
Emax: maximum effect; l-NAME: NG-nitro-l-arginine methyl ester; n: number of patients; pD2: −log EC50; SEM: standard error of mean; TEA: tetraethylammonium.
Effects of ranolazine on electrical stimulation-induced responses
Ranolazine (10−6–10−5 M) diminished neurogenic adrenergic contractions induced by EFS in saphenous rings in a concentration-dependent manner (Fig. 3A). Incubation with indomethacin (10−5 M) and l-NAME (10−4 M) did not modify the inhibitory effects of ranolazine on neurogenic contraction (Fig. 3B). Charybdotoxin (10−7 M) increased the contractile response to EFS and prevented the inhibitory effects of ranolazine, whereas apamin (10−6 M) and TRAM-34 (10−6 M) did not modify ranolazine effects (Fig. 3C). The combination of charybdotoxin (10−7 M) plus apamin (10−6 M) was similar to the effect of charybdotoxin alone (data not shown). Ranolazine (10−4 M) abolished contractile response induced by EFS both in the absence and presence of inhibitors.
![Frequency–response relationship (A) in control conditions and in the presence of ranolazine (10−6–10−5 M). Contractile response to electrical field stimulation (2 Hz) (B) in control conditions and in the presence of indomethacin (10−5 M) or l-NAME (10−4 M) alone and combination with ranolazine (10−5 M) and (C) control [indomethacin (10−5 M) plus l-NAME (10−4 M)] combined with charybdotoxin (10−7 M), TRAM-34 (10−6 M) or apamin (10−6 M) alone and in combination with ranolazine (10−5 M). n = 7 for each condition. Values are mean ± standard error of mean. *P < 0.05 compared with controls. l-NAME: NG-nitro-l-arginine methyl ester.](https://oup.silverchair-cdn.com/oup/backfile/Content_public/Journal/ejcts/58/2/10.1093_ejcts_ezaa034/1/m_ezaa034f3.jpeg?Expires=1747879426&Signature=KcTu4xS7i5QH7vKWNmiYUzyH0L-DK9PGwLNOSeHKiE0NBPnfz6~-1OsOkJtbs0Pel4f0YyBfgodCGOlKQ85yEKBKdS25uEnYBAl7qUagRicRkSGCRMmaEX~Fwt32Iv7cEDejFhEWpLcnCHcu8h1deP0QX0C2DJFq7I2QVDY6xTylHkJZkSUkn4zPYDH7c1kLEBumBeprr7ZNApxuSqXviHoYNgeFjXKCMOLvQj5zImA3Zt69iHjIMtrV8UhmGcSOc3PShBMdLoeUgj4gEh7AR6N7ci55Musawz3xwzGiMjVfuOSZmb-CoS5KP-EJTc6b88oDvL~Dqdkno4oi3KCQWQ__&Key-Pair-Id=APKAIE5G5CRDK6RD3PGA)
Frequency–response relationship (A) in control conditions and in the presence of ranolazine (10−6–10−5 M). Contractile response to electrical field stimulation (2 Hz) (B) in control conditions and in the presence of indomethacin (10−5 M) or l-NAME (10−4 M) alone and combination with ranolazine (10−5 M) and (C) control [indomethacin (10−5 M) plus l-NAME (10−4 M)] combined with charybdotoxin (10−7 M), TRAM-34 (10−6 M) or apamin (10−6 M) alone and in combination with ranolazine (10−5 M). n = 7 for each condition. Values are mean ± standard error of mean. *P < 0.05 compared with controls. l-NAME: NG-nitro-l-arginine methyl ester.
Effects of ranolazine on phenylephrine-induced contractions
Ranolazine (10−5–10−4 M) decreased the phenylephrine-induced contractions in a concentration-dependent manner (Fig. 4A and Table 2). Neither indomethacin (10−5 M) nor l-NAME (10−4 M) modified the effects induced by ranolazine (10−5 M) on phenylephrine-induced contractions (Fig. 4B). TEA (10−3 M) and charybdotoxin (10−7 M) significantly reduced the effects of ranolazine (10−5 M) (Fig. 4C), whereas apamin (10−6 M) and TRAM-34 (10−6 M) had no effects, suggesting that ranolazine effects on phenylephrine-induced contractions involve large conductance Ca2+-activated K+ channels. Table 2 summarizes the pD2 and Emax values in the different experimental conditions.
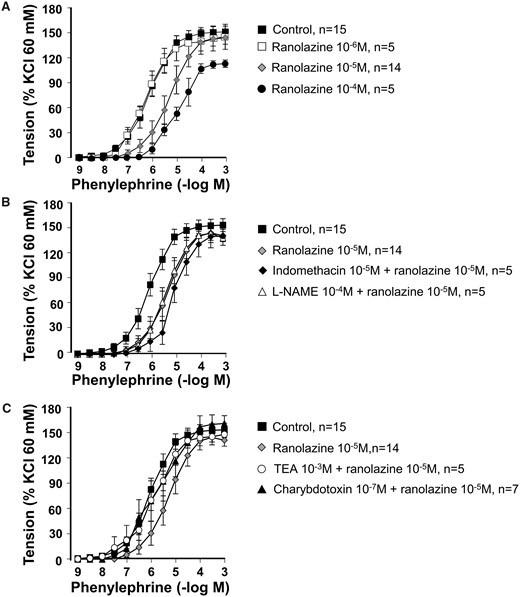
Concentration–response curves for phenylephrine in the absence (control) and presence of (A) ranolazine (10−6–10−4 M); (B) ranolazine (10−5 M) and ranolazine (10−5 M) plus indomethacin (10−5 M) or l-NAME (10−4 M); (C) ranolazine (10−5 M) and ranolazine (10−5 M) plus indomethacin (10−5 M) plus l-NAME (10−4 M) in combination with TEA (10−3 M) or charybdotoxin (10−7 M). Values are mean ± standard error of mean. l-NAME: NG-nitro-l-arginine methyl ester; TEA: tetraethylammonium.
pD2 values and Emax to phenylephrine in saphenous rings in control and experimental conditions
Phenylephrine . | n . | pD2 . | Emax . |
---|---|---|---|
Control | 15 | 6.03 ± 0.13 | 152 ± 8 |
Ranolazine 10−6 M | 5 | 6.22 ± 0.11 | 146 ± 10 |
Ranolazine 10−5 M | 14 | 5.31 ± 0.23* | 139 ± 7 |
Ranolazine 10−4 M | 5 | 5.17 ± 0.26* | 110 ± 4* |
Ranolazine 10−5 M + indomethacin 10−5 M | 5 | 4.93 ± 0.20 | 139 ± 11 |
Ranolazine 10−5 M + l-NAME 10−4 M | 5 | 5.34 ± 0.20 | 138 ± 2 |
Ranolazine 10−5 M + TEA 10−3 M | 5 | 5.74 ± 0.09 | 146 ± 4 |
Ranolazine 10−5 M + charybdotoxin 10−7 M | 7 | 5.61 ± 0.23 | 159 ± 11 |
Ranolazine 10−5 M + TRAM-34 10−6 M | 5 | 5.23 ± 0.24 | 138 ± 13 |
Ranolazine 10−5 M + apamin 10−6 M | 5 | 5.35 ± 0.39 | 133 ± 5 |
Phenylephrine . | n . | pD2 . | Emax . |
---|---|---|---|
Control | 15 | 6.03 ± 0.13 | 152 ± 8 |
Ranolazine 10−6 M | 5 | 6.22 ± 0.11 | 146 ± 10 |
Ranolazine 10−5 M | 14 | 5.31 ± 0.23* | 139 ± 7 |
Ranolazine 10−4 M | 5 | 5.17 ± 0.26* | 110 ± 4* |
Ranolazine 10−5 M + indomethacin 10−5 M | 5 | 4.93 ± 0.20 | 139 ± 11 |
Ranolazine 10−5 M + l-NAME 10−4 M | 5 | 5.34 ± 0.20 | 138 ± 2 |
Ranolazine 10−5 M + TEA 10−3 M | 5 | 5.74 ± 0.09 | 146 ± 4 |
Ranolazine 10−5 M + charybdotoxin 10−7 M | 7 | 5.61 ± 0.23 | 159 ± 11 |
Ranolazine 10−5 M + TRAM-34 10−6 M | 5 | 5.23 ± 0.24 | 138 ± 13 |
Ranolazine 10−5 M + apamin 10−6 M | 5 | 5.35 ± 0.39 | 133 ± 5 |
Data are shown as mean ± SEM. Emax are expressed as a percentage of response to 60 mM KCl.
P < 0.05 versus control.
Emax: maximum effect; l-NAME: NG-nitro-l-arginine methyl ester; n: number of patients; pD2: −log EC50; SEM: standard error of mean; TEA: tetraethylammonium.
pD2 values and Emax to phenylephrine in saphenous rings in control and experimental conditions
Phenylephrine . | n . | pD2 . | Emax . |
---|---|---|---|
Control | 15 | 6.03 ± 0.13 | 152 ± 8 |
Ranolazine 10−6 M | 5 | 6.22 ± 0.11 | 146 ± 10 |
Ranolazine 10−5 M | 14 | 5.31 ± 0.23* | 139 ± 7 |
Ranolazine 10−4 M | 5 | 5.17 ± 0.26* | 110 ± 4* |
Ranolazine 10−5 M + indomethacin 10−5 M | 5 | 4.93 ± 0.20 | 139 ± 11 |
Ranolazine 10−5 M + l-NAME 10−4 M | 5 | 5.34 ± 0.20 | 138 ± 2 |
Ranolazine 10−5 M + TEA 10−3 M | 5 | 5.74 ± 0.09 | 146 ± 4 |
Ranolazine 10−5 M + charybdotoxin 10−7 M | 7 | 5.61 ± 0.23 | 159 ± 11 |
Ranolazine 10−5 M + TRAM-34 10−6 M | 5 | 5.23 ± 0.24 | 138 ± 13 |
Ranolazine 10−5 M + apamin 10−6 M | 5 | 5.35 ± 0.39 | 133 ± 5 |
Phenylephrine . | n . | pD2 . | Emax . |
---|---|---|---|
Control | 15 | 6.03 ± 0.13 | 152 ± 8 |
Ranolazine 10−6 M | 5 | 6.22 ± 0.11 | 146 ± 10 |
Ranolazine 10−5 M | 14 | 5.31 ± 0.23* | 139 ± 7 |
Ranolazine 10−4 M | 5 | 5.17 ± 0.26* | 110 ± 4* |
Ranolazine 10−5 M + indomethacin 10−5 M | 5 | 4.93 ± 0.20 | 139 ± 11 |
Ranolazine 10−5 M + l-NAME 10−4 M | 5 | 5.34 ± 0.20 | 138 ± 2 |
Ranolazine 10−5 M + TEA 10−3 M | 5 | 5.74 ± 0.09 | 146 ± 4 |
Ranolazine 10−5 M + charybdotoxin 10−7 M | 7 | 5.61 ± 0.23 | 159 ± 11 |
Ranolazine 10−5 M + TRAM-34 10−6 M | 5 | 5.23 ± 0.24 | 138 ± 13 |
Ranolazine 10−5 M + apamin 10−6 M | 5 | 5.35 ± 0.39 | 133 ± 5 |
Data are shown as mean ± SEM. Emax are expressed as a percentage of response to 60 mM KCl.
P < 0.05 versus control.
Emax: maximum effect; l-NAME: NG-nitro-l-arginine methyl ester; n: number of patients; pD2: −log EC50; SEM: standard error of mean; TEA: tetraethylammonium.
Effects of ranolazine on endothelial nitric oxide synthase, ɑ1 adrenergic receptor and BKCa channel protein expression
Ranolazine significantly decreased ɑ1 adrenergic receptor (P = 0.02, n = 4) and increased BKCa channel protein expression in saphenous vein (P = 0.02, n = 4) without differences in endothelial nitric oxide synthase expression (P = 0.7, n = 4) (Fig. 5).
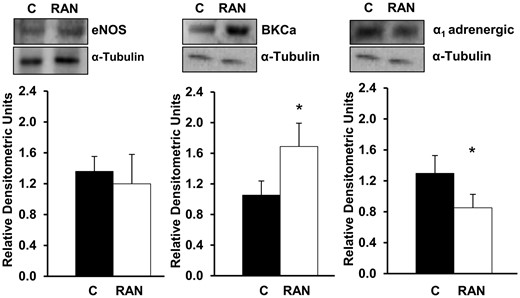
Effects of RAN on eNOS, BKCa channel and ɑ1 adrenergic receptor protein expression. Values are mean ± standard deviation for 4 independent experiments. *P < 0.05. BKCa: large conductance Ca2+-activated K+ channel; C: control; eNOS: endothelial nitric oxide synthase; RAN: ranolazine 10−5 M.
DISCUSSION
The main findings of our study are that, in saphenous veins of patients undergoing CABG, ranolazine, at concentrations equivalent to those used in the clinical setting, improved relaxation by (i) an endothelium NO dependent mechanism and (ii) an endothelium independent mechanism involving large conductance Ca2+-activated K+ channels and the blockade of L-type voltage-dependent Ca2+ channels. Furthermore, ranolazine decreased the adrenergic response by acting as an α1-antagonist.
Our results demonstrate that ranolazine induced vasorelaxation when human saphenous vein was precontracted with phenylephrine, but not with endothelin-1, vasopressin, KCl or thromboxane A2. This effect could be attributed to the blockade of α1 receptors, as previously reported [12]. In addition, different vasodilator mechanisms counterbalance the vasocontractile response, depending on vascular bed and type of agonist used. In this regard, previous studies demonstrated that vasopressin [20] and U-46619 [21] do not induce NO release in human saphenous vein. Therefore, it could partially explain why ranolazine only induced relaxation when precontracted with phenylephrine.
However, we cannot rule out the blockade of voltage-gated sodium channels by ranolazine at perivascular sympathetic nerve endings, preventing the release of catecholamines and thus the activation of postsynaptic α1-adrenergic receptor [18]. We found that ranolazine, at concentrations corresponding to therapeutic doses (2–8 μM) [22], decreased contractions due to both EFS and phenylephrine, indicating that ranolazine acutely modulates the action of the adrenergic neurotransmitters on smooth muscle rather than their release. Moreover, we found that ranolazine significantly reduces α1-adrenoceptors expression. Taken together, these results support the role of ranolazine as an α1-blocker in human saphenous vein. Since this vessel can undergo vasospasm after autologous grafts in the arterial circulation or CABG, ranolazine may limit smooth muscle contractions in response to adrenergic stimulation, contributing to protecting the venous grafts when the adrenergic tone is increased.
The role of the endothelium in the regulation of vascular tone and cardiovascular health is widely documented [23]. In our study, ranolazine induced a concentration-dependent relaxation in rings with and without endothelium, which was lower in denuded vessels. In endothelium intact rings, indomethacin did not modify the relaxation induced by ranolazine whereas l-NAME partially reduced it. These results indicate that part of the relaxation induced by ranolazine is due to NO and independent of PGI2. The remaining relaxation to ranolazine after inhibition of NO/PGI2 pathways was further reduced by blocking K+ channels. Previous studies suggest a small participation of the endothelium-derived hyperpolarization in the relaxation induced by ranolazine, which is closely related to the Ca2+-activated K+ channels [12]. Incubation with charybdotoxin, but not apamin or TRAM-34, significantly inhibited ranolazine-induced relaxation in both endothelium intact and denuded rings. In addition, charybdotoxin blocked ranolazine effects on both exogenous and endogenous adrenergic response. Furthermore, ranolazine increased large conductance Ca2+-activated K+ channels protein expression, suggesting that this type of channels contributes to the relaxant and antiadrenergic effects of ranolazine in human saphenous veins. Because these channels are mainly located in the vascular smooth muscle, we can assume that ranolazine activates large conductance Ca2+-activated K+ channels by an endothelium independent mechanism, and therefore, it could be useful in conditions associated with endothelial dysfunction. Most studies evaluating the effects of ranolazine on vascular tone agree that ranolazine induces relaxation mainly by an endothelium-independent mechanism, acting directly on smooth vascular muscle [12, 24]. We have previously reported that in saphenous veins from patients undergoing CABG, the blockade of large conductance Ca2+-activated K+ channels significantly potentiated adrenergic contractile effects, suggesting that these channels may participate in the regulation of human saphenous vein tone [19].
High concentrations (>30 μM) of ranolazine also block voltage-gated Ca2+ channels [12]. Our results demonstrated that verapamil significantly decreased the relaxation induced by ranolazine in the concentrations used, evidencing that L-type voltage-gated Ca2+ channels participate in the vasodilatation induced by ranolazine at both therapeutic and higher concentrations.
Surgical manipulation can lead to vein damage and impairment of vascular function and compromises both endothelium-dependent and independent vasodilation [25]. Vessel injury decreases NO bioavailability and promotes platelet adhesion, and consequently, thrombosis. Besides, saphenous vein produces less NO compared to arteries as had been demonstrated in non-grafted rings harvested from patients undergoing CABG [26]. Later, intimal hyperplasia is the leading cause of failure. The degree of intimal hyperplasia is inversely related to endothelium-dependent relaxation and directly related to the enhancement of contractile response in CABG patients [27]. The lack of endothelium integrity precludes its protective effects against cell proliferation as an adaptive mechanism in a vessel acutely exposed to high arterial pressures. In this regard, ranolazine improves endothelial-dependent relaxation in both patients with and without cardiovascular risk factors [9, 16].
Over time, atherosclerosis becomes the primary cause of graft failure [28]. Patients with significant graft stenosis are at higher risk of non-fatal myocardial infarction, repeat revascularization and cardiovascular death [29]. In this sense, in patients with stable coronary artery disease [9], ranolazine decreases C-reactive protein levels, an inflammatory marker and an independent strong predictor of cardiac events [30].
Limitations
One of the limitations of our study is that it was carried out in isolated vessels, thus lacking the effects of the integrated neuro-humoral, hormonal and cellular responses to the drug expected to be found in a living organ. However, this experimental approach allowed us to identify the direct mechanisms of ranolazine in the human saphenous vein, avoiding confounding factors that could have masked our findings.
CONCLUSION
In conclusion, our study provides evidence that ranolazine could be useful in the management of patients undergoing CABG because of its properties in enhancing vascular relaxation and decreasing adrenergic vasoconstriction, in addition to the effects against inflammation and platelet adhesion and the lack of significant adverse cardiovascular and haemodynamic effects.
SUPPLEMENTARY MATERIAL
Supplementary material is available at EJCTS online.
Funding
S.G.-O. has been funded by grant PDBCEx COLDOC 679, scholarship programme from COLCIENCIAS (Administrative Department of Science, Technology and Innovation of Colombia).
Conflict of interest: the authors declare that they have no conflict of interest.
Author contributions
Patricia Marchio: Formal analysis; Investigation; Supervision; Writing—original draft. Sol Guerra-Ojeda: Formal analysis; Investigation; Supervision; Writing—original draft. Martín Aldasoro: Conceptualization; Supervision; Validation. Soraya Lilian Valles: Conceptualization; Validation. Iván Martín-Gonzalez: Project administration; Validation; Writing—review & editing. Juan Bautista Martínez-León: Project administration; Validation; Writing—review & editing. Maria Dolores Mauricio: Data curation; Investigation; Writing—review & editing. Jose Maria Vila: Data curation; Supervision; Writing—original draft; Writing—review & editing.
Presented at the 31st Annual Meeting of the European Association for Cardio-Thoracic Surgery, Vienna, Austria, 7–11 October 2017.
REFERENCES
ABBREVIATIONS
- CABG
Coronary artery bypass grafting
- EFS
Electrical field stimulation
- L-NAME
NG-nitro-l-arginine methyl ester
- PGI2
Prostacyclin
- TEA
Tetraethylammonium
Author notes
Patricia Marchio and Sol Guerra-Ojeda contributed equally to this work and share first authorship.