-
PDF
- Split View
-
Views
-
Cite
Cite
Marcin Malinowski, Tomasz Jaźwiec, Matthew Goehler, Jared Bush, Nathan Quay, Haley Ferguson, Manuel K Rausch, Tomasz A Timek, Impact of tricuspid annular size reduction on right ventricular function, geometry and strain, European Journal of Cardio-Thoracic Surgery, Volume 56, Issue 2, August 2019, Pages 400–408, https://doi.org/10.1093/ejcts/ezy484
- Share Icon Share
Abstract
Restrictive tricuspid annuloplasty is a clinically accepted approach to treat functional tricuspid regurgitation. We set out to investigate the effect of varying degrees of tricuspid annular reduction on the right ventricular (RV) function, geometry and strain.
Eight, healthy sheep (45 ± 4 kg) had 6 sonomicrometry crystals implanted around the tricuspid annulus and 20 onto the epicardium of the right ventricle defining 3 free wall regions: basal, mid and lower. A polypropylene annuloplasty suture was placed around the tricuspid annulus and externalized to an epicardial tourniquet. Simultaneous echocardiographic, haemodynamic and sonomicrometry data were acquired at baseline and during 5 consecutive annular reduction steps (TAR 1–5) with successive (5–7 mm) suture cinching. RV free wall circumferential, longitudinal and areal cardiac and interventional strains, RV radius of curvature (ROC), cross-sectional area and tricuspid annular dimensions were calculated from 3-dimensional crystal coordinates.
TAR 1–5 resulted in 19 ± 15%, 35 ± 15%, 51 ± 15%, 60 ± 15% and 68 ± 13% tricuspid annular area reduction, respectively. TAR 1 and 2 had minimal influence on the RV function, RV-ROC and strains. TAR 4 and 5 decreased RV-ROC in basal and mid-regions, but reduced the RV cross-sectional area change (from 19 ± 4% at baseline to 14 ± 3% and 13 ± 2%, respectively, P < 0.001) and circumferential and areal strains. TAR 3 significantly decreased free wall RV-ROC from 44.0 ± 1.5 to 42.6 ± 2.4 mm P < 0.001 at the RV base but maintained the regional ventricular function and strains.
In healthy ovine hearts, a tricuspid annular area reduction of ∼50% provides optimal conditions for reducing RV-ROC while maintaining regional RV function and strain patterns.
INTRODUCTION
Tricuspid valve repair utilizing reductive annuloplasty is the preferred surgical treatment of functional tricuspid regurgitation (TR). Although the precise timing of surgical intervention remains debatable, there is a call towards more aggressive surgical therapy, guided by annular size and right ventricular (RV) function [1], for repair of concomitant functional TR to avoid subsequent high-risk reoperation [2]. Restrictive annuloplasty was initially proposed as a solution for secondary mitral regurgitation resulting from cardiomyopathy [3], and a similar approach has now been adopted for functional tricuspid insufficiency [4], yet the surgical technique has not been widely standardized. Despite numerous reports presenting positive effects of tricuspid annuloplasty on valvular regurgitation and RV function and geometry [5–8], there are no data on the influence of various degrees of annular reduction on regional RV shape and performance. As secondary TR and RV dysfunction are ‘synergistically related’ [9], detailed knowledge of the effect of annular restriction on ventricular geometry and performance is paramount to prevent TR recurrence and aid RV reverse remodelling. Using healthy adult sheep, we set out to investigate the influence of the magnitude of tricuspid annular reduction (TAR) on RV geometry, function and surface strain patterns. We hypothesized that there is a degree of annular size reduction beyond which there is a detrimental impact on RV function.
MATERIALS AND METHODS
All animals received humane care in compliance with the Principles of Laboratory Animal Care formulated by the National Society for Medical Research and the Guide for Care and Use of Laboratory Animals prepared by the National Academy of Science and published by the National Institutes of Health. The study protocol was approved by the local institutional animal care and use committee. Parts of the methods have previously been described [10].
Surgical procedure
Eight healthy castrated male Dorsett sheep (45 ± 4 kg) were used in the study. The animals were premedicated with propofol (2–5 mg/kg), intubated and mechanically ventilated. General anaesthesia was maintained with inhalational isoflurane (1–2.5%) and fentanyl (5–20 μg/kg/min). Median sternotomy was performed, the right carotid artery and the right internal jugular vein were cannulated for cardiopulmonary bypass, and the procedure was performed on the beating heart. Six 2-mm sonomicrometry crystals (Sonometrics Corporation, London, ON, Canada) were implanted around the tricuspid annulus with 3 crystals on the commissures and 3 in-between. Crystal electrodes were exteriorized through the right atriotomy. Additionally, 20 crystals were sutured onto the RV free wall along 4 equally spaced parallels defining 3 ventricular regions (basal, mid and lower; Fig. 1, Supplementary Material, Fig. S1). Two layers of 2-0 polypropylene sutures were sewn around the tricuspid annulus anchored at the anteroseptal commissure and mid-septal annulus. The sutures, representing a DeVega like annuloplasty, were externalized through the anteroposterior annulus to a tourniquet allowing for subsequent stepwise suture cinching [10]. The animal was weaned from cardiopulmonary bypass, and pressure transducers (PA4.5-X6; Konigsberg Instruments, Inc.) were placed in the right ventricle and the left ventricle through the apex and in the right atrium.

The location of sonomicrometry crystals implanted around the tricuspid annulus and on the epicardium of the right ventricular free wall defining 3 RV free wall regions. AL: anterior leaflet; PL: posterior leaflet; SL: septal leaflet.
Data acquisition
After 30 min of stable haemodynamics post-weaning from cardiopulmonary bypass, data acquisition was performed. Progressive tricuspid annular area reduction simulating undersized annuloplasty was achieved by 5 consecutive, measured (5–7 mm) pulls of the annular suture. Simultaneous haemodynamic, echocardiographic and sonomicrometry data were acquired before suture cinching (Baseline) and with each consecutive tricuspid annular reduction (TAR 1–5).
All data were recorded with Sonometrics Digital Ultrasonic Measurement System DS3 as previously reported [11]. Data from 3 consecutive heart cycles acquired at 128 Hz during sinus rhythm and steady-state haemodynamic conditions were merged to yield 3-dimensional co-ordinates of each sonomicrometry crystal for Baseline and each step of annular reduction. The time-resolved 3-dimensional crystal coordinates obtained from the SonoSoft software (Sonometrics Corporation) were analysed in MATLAB (MathWorks, Natic, MA, USA) using the custom written code. Least-squares cubic splines were fitted to discrete sonomicrometry annular crystal data to create approximations of the tricuspid annulus at each time point and for each animal [12]. To recreate smooth surface representations of the RV epicardial surfaces, we used the modified Loop subdivision algorithm [13]. Epicardial echocardiography (Vivid S6, GE Healthcare) was used to monitor the flow across the tricuspid valve and measure valvular gradients.
Right ventricular function and geometry
RV cross-sectional area was calculated based on 3-dimensional crystal co-ordinates as the area circumscribed by RV crystals at each level. The RV cross-sectional area change from its maximal to minimal value during heart cycle at each ventricular level was calculated and expressed in percentage; this parameter was used as a metric of regional RV free wall contractility. The RV radius of curvature (ROC) was determined as the radius of epicardial curvature for the epicardial crystals at each ventricular level. The RV volume was calculated using the convex hull method based on annular and epicardial crystal coordinates. RV fractional volume change, being a surrogate for RV ejection fraction, was calculated as (EDV − ESV)/EDV × 100%. The longitudinal diameter of the RV (RV length) was measured from the apex to the centroid of the annular plane.
Tricuspid annulus
The tricuspid annular area, perimeter and the septo-lateral distance were calculated based on crystal coordinates as previously described [14]. In brief, the tricuspid annular area was calculated as the area enclosed by the 2-dimensional projection of the spline approximation of the annulus onto its best-fit plane. The annular perimeter was measured as the arc-length integral along the annulus. The % annular area reduction was calculated as the % difference between the maximal and the minimal annular areas.
Right ventricular strains
To calculate RV epicardial strains in the beating ovine heart, we used an approach previously described [15, 16]. In brief, 25 triangles between RV crystal co-ordinates, identical between all animals and all time points, were created. Each triangular element was interpolated via linear shape functions to characterize surface deformation of the right ventricle. In particular, Green-Lagrange circumferential, longitudinal and areal strains were calculated for the basal, mid and lower RV free wall regions (Fig. 1). Areal strains combine changes in circumferential and longitudinal directions and thus represent the directionally independent total deformation. Average cardiac cycle strains, a measure of RV free wall regional function, were calculated for each ventricular region with end-diastole as the reference state.
Interventional strains, assessing the direct effect of annular size reduction on RV function, were calculated at end-diastole and end-systole (ES) for all interventions (TAR 1–5) with the same time point at Baseline serving as the reference. End-diastole was defined as the time of the beginning of positive deflection in ECG voltage (R wave), whereas ES was determined as the time of maximum negative dp/dt of left ventricular pressure.
Statistical analysis
All of the data are reported as mean ± 1 SD, unless otherwise stated. Statistical inference testing between the interventions (Baseline, TAR 1–5) was performed with repeated measures analysis of variance or Friedman repeated measures analysis of variance on ranks when the normality test failed, with TAR 1–5 compared to Baseline with the Dunnett’s test for multiple comparisons. The statistical difference between cardiac cycle RV strains was determined by comparing the data for ES. P-values <0.05 were considered significant. SigmaPlot 12.5 Software (SYSTAT, San Jose, CA, USA) was utilized for all statistical analyses.
RESULTS
Haemodynamics
All the animals underwent an uneventful surgery. Haemodynamic parameters during Baseline and with each progressive tricuspid annular size reduction are presented in Table 1. Progressive TAR resulted in an increased pressure gradient across the valve (although still below clinical significance) and decreased RV end-diastolic volume. TAR 4 and 5 were associated with increased central venous pressure, decreased RV fractional volume change and decreased left ventricular (LV) pressure. None of the levels of annular restriction affected the peak RV pressure.
Haemodynamics and right ventricular function during tricuspid annular size reduction
n = 8 . | Baseline . | TAR 1 . | TAR 2 . | TAR 3 . | TAR 4 . | TAR 5 . | P-value . |
---|---|---|---|---|---|---|---|
HR (min−1) | 102 ± 6 | 102 ± 6 | 102 ± 6 | 102 ± 6 | 100 ± 4 | 101 ± 5 | 0.002 |
LVPmax (mmHg) | 103 ± 8 | 102 ± 6 | 103 ± 12 | 95 ± 13 | 91 ± 10a | 86 ± 13a | <0.001 |
RVPmax (mmHg) | 29 ± 8 | 29 ± 7 | 30 ± 8 | 29 ± 9 | 29 ± 9 | 26 ± 6 | 0.13 |
RV-EDP (mmHg) | 5 ± 2 | 5 ± 2 | 5 ± 3 | 5 ± 2 | 5 ± 3 | 4 ± 2 | 0.37 |
CVP (mmHg) | 9 ± 2 | 9 ± 2 | 10 ± 2 | 11 ± 2 | 12 ± 2a | 12 ± 3a | <0.001 |
RV-EDV (ml) | 132 ± 10 | 132 ± 14 | 132 ± 12 | 121 ± 14a | 119 ± 18a | 115 ± 15a | <0.001 |
RV-FVC (%) | 17 ± 5 | 17 ± 4 | 17 ± 3 | 16 ± 3 | 13 ± 5a | 12 ± 3a | 0.002 |
Mean TV gradient (mmHg) | 0.85 ± 0.3 | 1.0 ± 0.5 | 1.3 ± 0.6 | 1.9 ± 1.1a | 2.4 ± 1.4a | 2.8 ± 1.5a | <0.001 |
n = 8 . | Baseline . | TAR 1 . | TAR 2 . | TAR 3 . | TAR 4 . | TAR 5 . | P-value . |
---|---|---|---|---|---|---|---|
HR (min−1) | 102 ± 6 | 102 ± 6 | 102 ± 6 | 102 ± 6 | 100 ± 4 | 101 ± 5 | 0.002 |
LVPmax (mmHg) | 103 ± 8 | 102 ± 6 | 103 ± 12 | 95 ± 13 | 91 ± 10a | 86 ± 13a | <0.001 |
RVPmax (mmHg) | 29 ± 8 | 29 ± 7 | 30 ± 8 | 29 ± 9 | 29 ± 9 | 26 ± 6 | 0.13 |
RV-EDP (mmHg) | 5 ± 2 | 5 ± 2 | 5 ± 3 | 5 ± 2 | 5 ± 3 | 4 ± 2 | 0.37 |
CVP (mmHg) | 9 ± 2 | 9 ± 2 | 10 ± 2 | 11 ± 2 | 12 ± 2a | 12 ± 3a | <0.001 |
RV-EDV (ml) | 132 ± 10 | 132 ± 14 | 132 ± 12 | 121 ± 14a | 119 ± 18a | 115 ± 15a | <0.001 |
RV-FVC (%) | 17 ± 5 | 17 ± 4 | 17 ± 3 | 16 ± 3 | 13 ± 5a | 12 ± 3a | 0.002 |
Mean TV gradient (mmHg) | 0.85 ± 0.3 | 1.0 ± 0.5 | 1.3 ± 0.6 | 1.9 ± 1.1a | 2.4 ± 1.4a | 2.8 ± 1.5a | <0.001 |
Data are presented as means ± 1 SD. RV volume includes myocardium. P-value from RM ANOVA.
P-value <0.05 versus Baseline using the Dunnett’s test.
ANOVA: analysis of variance; CVP: central venous pressure; EDP: end-diastolic pressure; EDV: end-diastolic volume; FVC: fractional volume change; HR: heart rate; LVP: left ventricular pressure; RM: repeated measures; RV: right ventricular; RVP: right ventricular pressure; SD: standard deviation; TAR: tricuspid annular reduction; TV: tricuspid valve.
Haemodynamics and right ventricular function during tricuspid annular size reduction
n = 8 . | Baseline . | TAR 1 . | TAR 2 . | TAR 3 . | TAR 4 . | TAR 5 . | P-value . |
---|---|---|---|---|---|---|---|
HR (min−1) | 102 ± 6 | 102 ± 6 | 102 ± 6 | 102 ± 6 | 100 ± 4 | 101 ± 5 | 0.002 |
LVPmax (mmHg) | 103 ± 8 | 102 ± 6 | 103 ± 12 | 95 ± 13 | 91 ± 10a | 86 ± 13a | <0.001 |
RVPmax (mmHg) | 29 ± 8 | 29 ± 7 | 30 ± 8 | 29 ± 9 | 29 ± 9 | 26 ± 6 | 0.13 |
RV-EDP (mmHg) | 5 ± 2 | 5 ± 2 | 5 ± 3 | 5 ± 2 | 5 ± 3 | 4 ± 2 | 0.37 |
CVP (mmHg) | 9 ± 2 | 9 ± 2 | 10 ± 2 | 11 ± 2 | 12 ± 2a | 12 ± 3a | <0.001 |
RV-EDV (ml) | 132 ± 10 | 132 ± 14 | 132 ± 12 | 121 ± 14a | 119 ± 18a | 115 ± 15a | <0.001 |
RV-FVC (%) | 17 ± 5 | 17 ± 4 | 17 ± 3 | 16 ± 3 | 13 ± 5a | 12 ± 3a | 0.002 |
Mean TV gradient (mmHg) | 0.85 ± 0.3 | 1.0 ± 0.5 | 1.3 ± 0.6 | 1.9 ± 1.1a | 2.4 ± 1.4a | 2.8 ± 1.5a | <0.001 |
n = 8 . | Baseline . | TAR 1 . | TAR 2 . | TAR 3 . | TAR 4 . | TAR 5 . | P-value . |
---|---|---|---|---|---|---|---|
HR (min−1) | 102 ± 6 | 102 ± 6 | 102 ± 6 | 102 ± 6 | 100 ± 4 | 101 ± 5 | 0.002 |
LVPmax (mmHg) | 103 ± 8 | 102 ± 6 | 103 ± 12 | 95 ± 13 | 91 ± 10a | 86 ± 13a | <0.001 |
RVPmax (mmHg) | 29 ± 8 | 29 ± 7 | 30 ± 8 | 29 ± 9 | 29 ± 9 | 26 ± 6 | 0.13 |
RV-EDP (mmHg) | 5 ± 2 | 5 ± 2 | 5 ± 3 | 5 ± 2 | 5 ± 3 | 4 ± 2 | 0.37 |
CVP (mmHg) | 9 ± 2 | 9 ± 2 | 10 ± 2 | 11 ± 2 | 12 ± 2a | 12 ± 3a | <0.001 |
RV-EDV (ml) | 132 ± 10 | 132 ± 14 | 132 ± 12 | 121 ± 14a | 119 ± 18a | 115 ± 15a | <0.001 |
RV-FVC (%) | 17 ± 5 | 17 ± 4 | 17 ± 3 | 16 ± 3 | 13 ± 5a | 12 ± 3a | 0.002 |
Mean TV gradient (mmHg) | 0.85 ± 0.3 | 1.0 ± 0.5 | 1.3 ± 0.6 | 1.9 ± 1.1a | 2.4 ± 1.4a | 2.8 ± 1.5a | <0.001 |
Data are presented as means ± 1 SD. RV volume includes myocardium. P-value from RM ANOVA.
P-value <0.05 versus Baseline using the Dunnett’s test.
ANOVA: analysis of variance; CVP: central venous pressure; EDP: end-diastolic pressure; EDV: end-diastolic volume; FVC: fractional volume change; HR: heart rate; LVP: left ventricular pressure; RM: repeated measures; RV: right ventricular; RVP: right ventricular pressure; SD: standard deviation; TAR: tricuspid annular reduction; TV: tricuspid valve.
Tricuspid annulus
Changes in annular geometry and dynamics with each TAR step are summarized in Table 2. As intended, the gradual pull of the cinching suture resulted in a stepwise decrease in the tricuspid annular size. TAR 1–5 resulted in approximately 20%, 35%, 50%, 60% and 70% annular area reduction, respectively. This also led to a significant decrease in the annular circumference. Despite considerable size reduction, annular sphincteric function during the cardiac cycle remained unaltered even with aggressive (TAR 5) area cinching. Septo-lateral annular dimension, measured between mid-anterior and mid-septal crystals, demonstrated an 8 ± 6%, 15 ± 6%, 26 ± 7%, 32 ± 8% and 37 ± 8% decrease with TAR 1–5, respectively (all P < 0.01 vs Baseline).
n = 8 . | Baseline . | TAR 1 . | TAR 2 . | TAR 3 . | TAR 4 . | TAR 5 . | P-value . |
---|---|---|---|---|---|---|---|
Area (mm2) | |||||||
Maximal | 596 ± 98 | 477 ± 92a | 387 ± 95a | 292 ± 92a | 240 ± 92a | 192 ± 75a | <0.001 |
Minimal | 536 ± 89 | 440 ± 76a | 357 ± 81a | 266 ± 89a | 218 ± 85a | 174 ± 70a | <0.001 |
Δ Maximum–minimum (%) | 10 ± 4 | 7 ± 3 | 7 ± 3 | 10 ± 4 | 10 ± 4 | 10 ± 4 | 0.334 |
Perimeter (mm) | |||||||
Maximal | 90 ± 7 | 81 ± 7a | 74 ± 8a | 66 ± 9a | 61 ± 9a | 56 ± 8a | <0.001 |
Minimal | 87 ± 7 | 79 ± 7a | 73 ± 8a | 64 ± 9a | 60 ± 8a | 55 ± 8a | <0.001 |
S-L diameterED (mm) | 25.2 ± 2.8 | 23.1 ± 2.2a | 21.4 ± 2.5a | 18.7 ± 2.8a | 17.2 ± 3.2a | 16.1 ± 3.2a | <0.001 |
n = 8 . | Baseline . | TAR 1 . | TAR 2 . | TAR 3 . | TAR 4 . | TAR 5 . | P-value . |
---|---|---|---|---|---|---|---|
Area (mm2) | |||||||
Maximal | 596 ± 98 | 477 ± 92a | 387 ± 95a | 292 ± 92a | 240 ± 92a | 192 ± 75a | <0.001 |
Minimal | 536 ± 89 | 440 ± 76a | 357 ± 81a | 266 ± 89a | 218 ± 85a | 174 ± 70a | <0.001 |
Δ Maximum–minimum (%) | 10 ± 4 | 7 ± 3 | 7 ± 3 | 10 ± 4 | 10 ± 4 | 10 ± 4 | 0.334 |
Perimeter (mm) | |||||||
Maximal | 90 ± 7 | 81 ± 7a | 74 ± 8a | 66 ± 9a | 61 ± 9a | 56 ± 8a | <0.001 |
Minimal | 87 ± 7 | 79 ± 7a | 73 ± 8a | 64 ± 9a | 60 ± 8a | 55 ± 8a | <0.001 |
S-L diameterED (mm) | 25.2 ± 2.8 | 23.1 ± 2.2a | 21.4 ± 2.5a | 18.7 ± 2.8a | 17.2 ± 3.2a | 16.1 ± 3.2a | <0.001 |
Data are presented as means ± 1 SD. S-L distance represents the distance between mid-anterior and mid-septal annulus. P-value from RM ANOVA.
P-value <0.05 versus Baseline using the Dunnett’s test.
ANOVA: analysis of variance; RM: repeated measures; SD: standard deviation; S-L: septo-lateral; TAR: tricuspid annular reduction.
n = 8 . | Baseline . | TAR 1 . | TAR 2 . | TAR 3 . | TAR 4 . | TAR 5 . | P-value . |
---|---|---|---|---|---|---|---|
Area (mm2) | |||||||
Maximal | 596 ± 98 | 477 ± 92a | 387 ± 95a | 292 ± 92a | 240 ± 92a | 192 ± 75a | <0.001 |
Minimal | 536 ± 89 | 440 ± 76a | 357 ± 81a | 266 ± 89a | 218 ± 85a | 174 ± 70a | <0.001 |
Δ Maximum–minimum (%) | 10 ± 4 | 7 ± 3 | 7 ± 3 | 10 ± 4 | 10 ± 4 | 10 ± 4 | 0.334 |
Perimeter (mm) | |||||||
Maximal | 90 ± 7 | 81 ± 7a | 74 ± 8a | 66 ± 9a | 61 ± 9a | 56 ± 8a | <0.001 |
Minimal | 87 ± 7 | 79 ± 7a | 73 ± 8a | 64 ± 9a | 60 ± 8a | 55 ± 8a | <0.001 |
S-L diameterED (mm) | 25.2 ± 2.8 | 23.1 ± 2.2a | 21.4 ± 2.5a | 18.7 ± 2.8a | 17.2 ± 3.2a | 16.1 ± 3.2a | <0.001 |
n = 8 . | Baseline . | TAR 1 . | TAR 2 . | TAR 3 . | TAR 4 . | TAR 5 . | P-value . |
---|---|---|---|---|---|---|---|
Area (mm2) | |||||||
Maximal | 596 ± 98 | 477 ± 92a | 387 ± 95a | 292 ± 92a | 240 ± 92a | 192 ± 75a | <0.001 |
Minimal | 536 ± 89 | 440 ± 76a | 357 ± 81a | 266 ± 89a | 218 ± 85a | 174 ± 70a | <0.001 |
Δ Maximum–minimum (%) | 10 ± 4 | 7 ± 3 | 7 ± 3 | 10 ± 4 | 10 ± 4 | 10 ± 4 | 0.334 |
Perimeter (mm) | |||||||
Maximal | 90 ± 7 | 81 ± 7a | 74 ± 8a | 66 ± 9a | 61 ± 9a | 56 ± 8a | <0.001 |
Minimal | 87 ± 7 | 79 ± 7a | 73 ± 8a | 64 ± 9a | 60 ± 8a | 55 ± 8a | <0.001 |
S-L diameterED (mm) | 25.2 ± 2.8 | 23.1 ± 2.2a | 21.4 ± 2.5a | 18.7 ± 2.8a | 17.2 ± 3.2a | 16.1 ± 3.2a | <0.001 |
Data are presented as means ± 1 SD. S-L distance represents the distance between mid-anterior and mid-septal annulus. P-value from RM ANOVA.
P-value <0.05 versus Baseline using the Dunnett’s test.
ANOVA: analysis of variance; RM: repeated measures; SD: standard deviation; S-L: septo-lateral; TAR: tricuspid annular reduction.
Right ventricular function and geometry
The successive steps of annular size reduction resulted in the progressive decrease of RV-ROC at basal and midventricular levels with no changes versus the baseline condition at the lower level (Table 3). These alterations in RV free wall curvature began to be significant at TAR 3 and beyond. No change in RV length was observed during any of the annular interventions. RV cross-sectional area change remained unaffected with TAR 1 and 2 and was mildly reduced at the midventricular level only with TAR 3 (Fig. 2). In contrast, TAR 4 and 5 produced significant deterioration of RV free wall function in all studied regions.
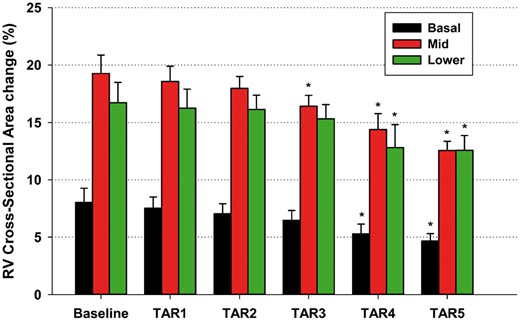
RV cross-sectional area change at basal, mid and lower regions at Baseline and 5 steps of tricuspid annular reduction. Data are presented as means ± 1 standard error of the mean, *P < 0.05 from repeated measures analysis of variance with the Dunnett’s post hoc test versus Baseline. RV: right ventricular; TAR: tricuspid annular reduction.
n = 8 . | Baseline . | TAR 1 . | TAR 2 . | TAR 3 . | TAR 4 . | TAR 5 . | P-value . |
---|---|---|---|---|---|---|---|
RV-ROCED (mm) | |||||||
Basal | 44.0 ± 1.5 | 43.6 ± 2.0 | 43.1 ± 2.2 | 42.6 ± 2.4a | 42.6 ± 2.4a | 41.8 ± 2.8a | <0.001 |
Mid | 39.0 ± 1.7 | 38.9 ± 2.0 | 38.4 ± 1.7a | 38.0 ± 1.8a | 37.9 ± 1.8a | 37.3 ± 1.8a | <0.001 |
Lower | 29.7 ± 1.7 | 29.9 ± 1.7 | 29.6 ± 1.6 | 29.4 ± 1.6 | 29.7 ± 1.8 | 29.4 ± 1.5 | 0.001 |
RV length (mm) | 65.2 ± 4.0 | 65.8 ± 4.9 | 65.9 ± 5.4 | 65.2 ± 4.4 | 65.2 ± 4.5 | 65.3 ± 4.5 | 0.47 |
n = 8 . | Baseline . | TAR 1 . | TAR 2 . | TAR 3 . | TAR 4 . | TAR 5 . | P-value . |
---|---|---|---|---|---|---|---|
RV-ROCED (mm) | |||||||
Basal | 44.0 ± 1.5 | 43.6 ± 2.0 | 43.1 ± 2.2 | 42.6 ± 2.4a | 42.6 ± 2.4a | 41.8 ± 2.8a | <0.001 |
Mid | 39.0 ± 1.7 | 38.9 ± 2.0 | 38.4 ± 1.7a | 38.0 ± 1.8a | 37.9 ± 1.8a | 37.3 ± 1.8a | <0.001 |
Lower | 29.7 ± 1.7 | 29.9 ± 1.7 | 29.6 ± 1.6 | 29.4 ± 1.6 | 29.7 ± 1.8 | 29.4 ± 1.5 | 0.001 |
RV length (mm) | 65.2 ± 4.0 | 65.8 ± 4.9 | 65.9 ± 5.4 | 65.2 ± 4.4 | 65.2 ± 4.5 | 65.3 ± 4.5 | 0.47 |
Data are presented as means ± 1 SD. P-value from RM ANOVA.
P-value <0.05 versus Baseline using the Dunnett’s test.
ANOVA: analysis of variance; RM: repeated measures; ROC: radius of curvature; RV: right ventricle; SD: standard deviation; TAR: tricuspid annular reduction.
n = 8 . | Baseline . | TAR 1 . | TAR 2 . | TAR 3 . | TAR 4 . | TAR 5 . | P-value . |
---|---|---|---|---|---|---|---|
RV-ROCED (mm) | |||||||
Basal | 44.0 ± 1.5 | 43.6 ± 2.0 | 43.1 ± 2.2 | 42.6 ± 2.4a | 42.6 ± 2.4a | 41.8 ± 2.8a | <0.001 |
Mid | 39.0 ± 1.7 | 38.9 ± 2.0 | 38.4 ± 1.7a | 38.0 ± 1.8a | 37.9 ± 1.8a | 37.3 ± 1.8a | <0.001 |
Lower | 29.7 ± 1.7 | 29.9 ± 1.7 | 29.6 ± 1.6 | 29.4 ± 1.6 | 29.7 ± 1.8 | 29.4 ± 1.5 | 0.001 |
RV length (mm) | 65.2 ± 4.0 | 65.8 ± 4.9 | 65.9 ± 5.4 | 65.2 ± 4.4 | 65.2 ± 4.5 | 65.3 ± 4.5 | 0.47 |
n = 8 . | Baseline . | TAR 1 . | TAR 2 . | TAR 3 . | TAR 4 . | TAR 5 . | P-value . |
---|---|---|---|---|---|---|---|
RV-ROCED (mm) | |||||||
Basal | 44.0 ± 1.5 | 43.6 ± 2.0 | 43.1 ± 2.2 | 42.6 ± 2.4a | 42.6 ± 2.4a | 41.8 ± 2.8a | <0.001 |
Mid | 39.0 ± 1.7 | 38.9 ± 2.0 | 38.4 ± 1.7a | 38.0 ± 1.8a | 37.9 ± 1.8a | 37.3 ± 1.8a | <0.001 |
Lower | 29.7 ± 1.7 | 29.9 ± 1.7 | 29.6 ± 1.6 | 29.4 ± 1.6 | 29.7 ± 1.8 | 29.4 ± 1.5 | 0.001 |
RV length (mm) | 65.2 ± 4.0 | 65.8 ± 4.9 | 65.9 ± 5.4 | 65.2 ± 4.4 | 65.2 ± 4.5 | 65.3 ± 4.5 | 0.47 |
Data are presented as means ± 1 SD. P-value from RM ANOVA.
P-value <0.05 versus Baseline using the Dunnett’s test.
ANOVA: analysis of variance; RM: repeated measures; ROC: radius of curvature; RV: right ventricle; SD: standard deviation; TAR: tricuspid annular reduction.
Right ventricular strains
Cardiac cycle strains
The RV surface cardiac strain analysis revealed less negative average circumferential and areal strains with the highest degrees of annular undersizing (TAR 4 and 5; Figs 3 and 4). The end-systolic circumferential strain at the RV base changed in magnitude from −4.7 ± 1.1% at Baseline to −2.5 ± 1.9% and −2.7 ± 1.0% with TAR 4 and 5, respectively (P < 0.05 vs Baseline). The magnitude of areal cardiac cycle strain changed from −17 ± 5% at Baseline to −13 ± 3% with TAR 4 and −12 ± 2% with TAR 5 (P < 0.05). There was no change in the longitudinal cardiac strain in any region (Supplementary Material, Fig. S2).
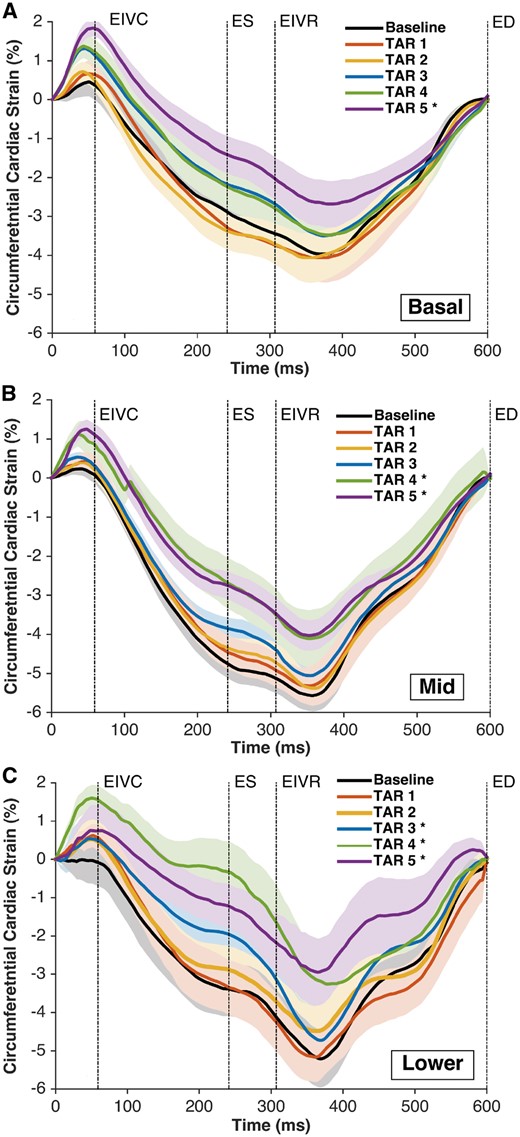
Right ventricular regional free wall average epicardial ‘circumferential’ cardiac strain at Baseline and TAR 1–5. (A) Basal region, (B) mid-region and (C) lower region. The strain was calculated with the reference configuration at ED and is presented as ± standard error of the mean during the averaged cardiac cycle (n = 8). Regions as depicted in Fig. 1. *P < 0.05 from repeated measures analysis of variance with the Dunnett’s post hoc test versus Baseline at ES. ED: end-diastole; EIVC: end of isovolumic contraction; EIVR: end of isovolumic relaxation; ES: end-systole; TAR: tricuspid annular reduction.
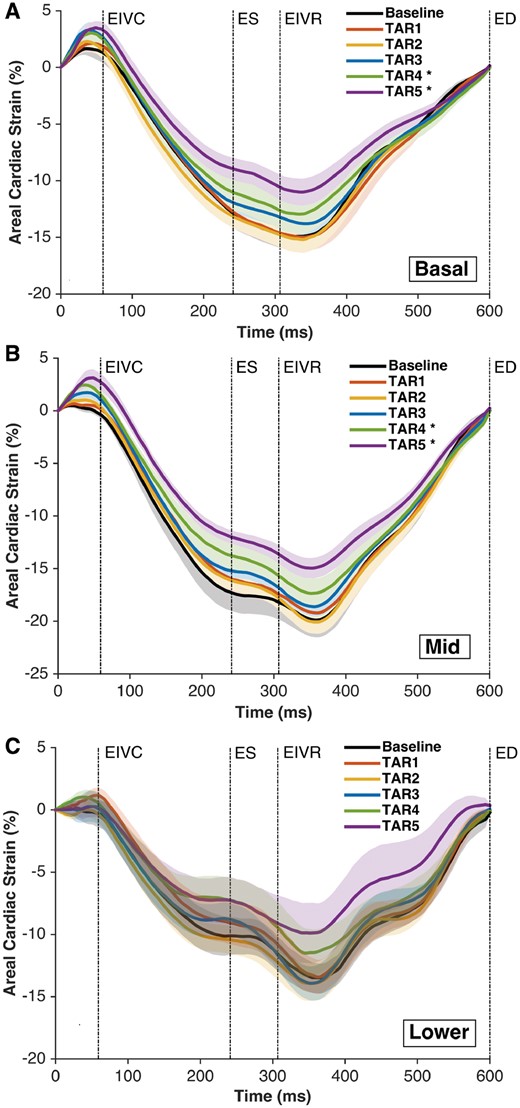
Right ventricular regional free wall average epicardial ‘areal’ cardiac strain at Baseline and TAR 1–5. (A) Basal region, (B) mid-region and (C) lower region. Strain was calculated with the reference configuration at ED and is presented as ± standard error of the mean during the averaged cardiac cycle (n = 8). Regions as depicted in Fig. 1. *P < 0.05 from repeated measures analysis of variance with the Dunnett’s post hoc test versus Baseline at ES. ED: end-diastole; EIVC: end of isovolumic contraction; EIVR: end of isovolumic relaxation; ES: end-systole; TAR: tricuspid annular reduction.
Interventional strains
Figure 5 presents the colour maps of interventional strains at both end-diastole and ES. Of note is the gradual increase of myocardial RV free wall compression with progression of annular size reduction becoming most evident with TAR 4 and 5. The compression was most pronounced in the basal ventricular region close to the posterior free wall/septal border. Concurrently, the mid-portion of the RV free wall was stretched in the longitudinal direction. The analysis of direction-independent areal strain confirmed significant diastolic and systolic compression of the RV base relative to Baseline (Video 1).
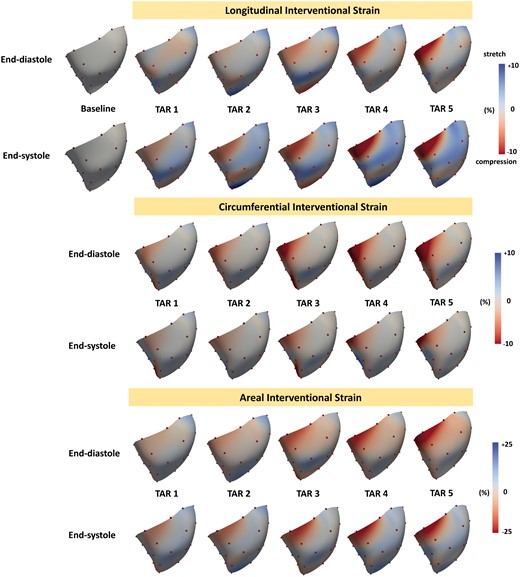
Colour maps of right ventricular (RV) free wall interventional strains during TAR 1–5: longitudinal RV epicardial strain (top panel), circumferential RV epicardial strain (mid-panel) and areal RV epicardial strain (lower panel). Strains are presented at end-diastole and end-systole for each intervention (TAR 1–5) with the reference configuration at Baseline. The positive values (blue colour) indicate the ventricular stretch, whereas the negative values (red colour) indicate the ventricular compression. TAR: tricuspid annular reduction.
Right ventricular free wall areal interventional strain during 3 averaged cardiac cycles with 70% tricuspid annular reduction (TAR 5).
DISCUSSION
Our experimental study performed on healthy animals revealed that progressive TAR significantly affected the RV geometry and function with an ∼50% reduction resulting in a smaller RV size and ROC and still preserved the regional myocardial function and strain patterns. Tricuspid annular reduction beyond 50% of the initial size not only altered the ventricular size and geometry but also significantly impaired the regional myocardial function and strains. On the other hand, annular restriction of substantially <50% had little influence on the RV performance or shape.
The concept of restrictive annuloplasty as therapy for functional valvular insufficiency stems from Boling’s introduction of undersized ring annuloplasty for the treatment of functional mitral regurgitation associated with end stage-cardiomyopathy as an ‘annular treatment of a ventricular problem’ [3]. Restrictive mitral annuloplasty has since been extensively studied and shown to be effective in both experimental [17] and clinical settings [18] with favourable effects on valvular insufficiency and left ventricular dimensions, ROC and wall stress [17, 19]. Subsequently, restrictive annular reduction was adopted for the treatment of functional TR [4], yet there are limited data regarding optimal annular downsizing to improve the subvalvular geometry [20] without negatively influencing the regional myocardial function [21]. The advocates of restrictive mitral annuloplasty suggest to downsize the mitral ring by 2 sizes of the actual annular measurement [18]. Ghoreishi et al. [4] suggested to simply use a 28- or 26-mm ring for functional TR, which demonstrated good immediate competency and satisfactory mid-term results [22], but it is not possible to ascertain the degree at which the tricuspid annulus was actually undersized from these reports. Late recurrent TR was predominantly associated with the development of RV dysfunction [22] perhaps partially due to over aggressive annular reduction in accordance with our experimental data. As such, the proposed ‘2 sizes fit all’ approach to secondary TR may achieve valvular competence yet jeopardize the right ventricle as these patients usually present with a broad spectrum of concomitant pathologies, including pulmonary hypertension, various stages of RV dysfunction and left-side valvular pathologies. A more guided method of implanting an annuloplasty prosthesis, 1 size smaller than suggested by anterior leaflet measurement, has shown in fact to be very effective in durably addressing TR and inducing RV reverse remodelling [8]. It is tempting to conjecture that a lesser degree of annular reduction may provide valvular competence and optimize the RV shape while maintaining regional function and strain patterns as observed with TAR 3 in our study.
With annular undersizing, increased transvalvular pressure gradients must also be taken into consideration [23], but clinically meaningful tricuspid stenosis is rare even with aggressive annular reduction [24]. These clinical data are substantiated by our findings of a mean gradient of <3 mmHg with TAR 5, which was associated with 68% annular area reduction in our animal study. However, our suture annuloplasty maintained annular dynamics even with very substantial reduction, which may not be true with ring prostheses. The observed maintenance of physiological annular dynamics corroborates the clinical success of a ‘tight’ DeVega annuloplasty [20].
Early surgical intervention for functional TR is recommended by the current guidelines [1, 25] with annular dilatation (>40 mm) alone [1], even in the absence of substantial TR, representing an indication for surgery. This approach is supported by our data, which demonstrate that annular reduction, in the absence of valvular incompetence, reduces the RV shape and ROC and may therefore optimize myocardial wall stress and energetics. Clinically, Bertrand et al. [7] demonstrated that ring annuloplasty for tricuspid annular dilation improved RV sphericity (less globular chamber) in the absence of significant TR. These findings suggest a direct effect of annular reduction on subvalvular geometry that is not mediated through reduction of volume overload from correcting significant valvular insufficiency. However, overall RV remodelling was more evident in patients with significant preoperative TR. Similarly, in a large patient cohort with over 80% of subjects presenting with mild or less TR, Chikwe et al. [5] found tricuspid annuloplasty to be a positive determinant of RV recovery at mid-term follow-up. Whether the positive effect of ring annuloplasty in the absence of significant TR is mediated through its effect on chamber geometry or prophylactic maintenance of valvular competence, or both requires further study.
Prior experimental ovine studies have shown that substantial mitral annular reduction can deleteriously affect basal LV function and strain [21]. To the best of our knowledge, the current study is the first to report the influence of tricuspid reduction on the RV strain patterns and regional function. Our experimental data revealed a heterogeneous response of the basal RV region to annular reduction with significant compression in the posterior basal portion of the right ventricle in both longitudinal and circumferential direction and no change, and even some myocardial stretch, in its anterior part. Regional differences in tricuspid annular anatomy may underlie these findings. Kawada found the posterior portion of the annulus to be distributed along the RV free wall, whereas the anterior segment is supported by the supraventricular crest [26]. Thus, without additional support structures engaging the forces from annular reduction, the effect of annuloplasty on the posterior portion of the ventricle may be expected to be more pronounced. Longitudinal cardiac strains remained unaffected by annular reduction perhaps due to orientation of RV myocardial fibres, which in the epicardium run mostly circumferentially [27]. Ventricular dysfunction and RV chamber remodelling are the central elements in the vicious cycle leading to tricuspid annular dilation, leaflets tethering and TR [28]. As RV dysfunction is a known predictor of survival after surgery [29], the treatment goal of restrictive annuloplasty should not only be to achieve a competent valve but to also adequately tailor surgery to allow for RV reverse remodelling. As such, the balance between valvular competence and optimal RV geometry and function should be contemplated when repairing functional TR. The reduction of RV-ROC can be expected to decrease wall stress and promote RV reverse remodelling. This subvalvular effect became evident in our study after a 50% annular size reduction (TAR 3), whereas baseline regional myocardial function and strain patterns were still relatively maintained.
Limitations
The result of the current study should be viewed in the context of several limitations. Our experiment was performed in open-chest healthy sheep under anaesthesia. The direct clinical extrapolation of the results of this experimental study must be done with great caution. However, we believe that keeping in mind the effect of annular reduction on the right ventricle may be beneficial when repairing the tricuspid valve. It is unclear whether the 50% reduction threshold seen in normal sheep hearts is also optimal in chronic RV dysfunction and functional TR, and this remains to be studied. Also, due to the lack of precise intraoperative tools to measure annular size pre- and post-reduction, reducing the annulus to a target size may not be easy to achieve clinically. However, conceptually, aggressive undersizing, based on these initial data, should probably be avoided.
The surgeries were performed on animals without RV dysfunction/dilation and with normal tricuspid annular size, but the use of healthy animals permitted the assessment of the direct effect of annular restriction on RV geometry, function and strain without the potentially confounding influence of volume/pressure overload associated with functional TR and its correction with annular reduction. We utilized a DeVega like polypropylene suture as a tool to undersize the annulus and perform multiple steps of annular restriction in each study animal, but this scenario differs from clinical implantation of annular prostheses of varying shapes and material properties. We evaluated only epicardial strains using wired sonomicrometry crystals, and no information regarding mid-wall and endocardial strains can be deemed from our study. Although the study demonstrated the relationship between the degree of tricuspid annular undersizing and RV geometry and function, further studies in a chronic model of functional TR and RV dysfunction are needed to validate these data.
CONCLUSION
In conclusion, in healthy sheep, tricuspid annular area size reduction of up to 35% had very little influence on RV free wall function, ROC and strain, whereas tricuspid restriction beyond 50% of its initial area significantly decreased RV-ROC, regional wall function and strains. The results of the current study suggest that annular reduction of ∼50% may be ideal for optimizing RV geometry while maintaining regional RV function and strain patterns.
ACKNOWLEDGEMENTS
M.M. and T.J. are the Peter C and Pat Cook Endowed Research Fellows in Cardiothoracic Surgery.
Funding
This work was supported by the internal grant from Meijer Heart and Vascular Institute at Spectrum Health.
Conflict of interest: none declared.
Footnotes
Presented at the 32nd Annual Meeting of the European Association for Cardio-Thoracic Surgery, Milan, Italy, 18–20 October 2018.